The ultimate goal of glaucoma treatment is to preserve enough vision during the patient’s lifetime to meet their functional needs; ideally, treatment should also delay, stop, and sometimes reverse the damage to the optic nerve and ganglion cell layer caused by the glaucomatous process. The only way currently proven to slow or stop damage from progressing is to reduce intraocular pressure (IOP) below the level that will cause continued damage to the optic nerve. Although a medication that would directly protect the optic nerve or reverse the damage from glaucoma would be most welcome, no such medication has yet been proven to be effective. Medications that have some promise in this regard are discussed in a later chapter.
Although the concept that lowering IOP truly helps prevent or reduce glaucomatous damage has been called into question, the overwhelming majority of studies produce evidence that lowering IOP is indeed beneficial. As has been noted in Chapters 17 and 21, animal studies and secondary glaucomas clearly point to IOP as an important risk factor, if not a causal factor. Other studies strongly suggest that the lower the IOP, the less likely optic nerve damage is to develop or progress. Some of these same data indicate that past efforts to lower IOP into the ‘normal’ range of 21 mmHg or lower may have been inadequate and that ‘control’ really means an IOP of less than 15 or 16 mmHg, especially in advanced glaucoma. One recent long-term study showed that lowering IOP in normal-pressure glaucoma by at least 30% protects at least some patients from progression. The issue has been put to rest by the publication of four long-term, controlled trials that conclusively show that lowering IOP will slow or delay the appearance or progression of glaucomatous damage; these include the Ocular Hypertension Treatment Study (OHTS), the Advanced Glaucoma Intervention Study (AGIS), the Collaborative Normal-Tension Glaucoma Study (CNTGS), and the Early Manifest Glaucoma Trial (EMGT). Many of these studies have helped to identify risk factors that auger progression within fully manifest glaucoma and from ocular hypertension to manifest glaucoma. These risk factors are outlined in Boxes 22-1 and 22-2 . Fluctuation of IOP has also been identified as an important risk factor for progression.
Corneal thickness under 535 microns
Elevated IOP
Increasing age
Enlarged vertical cup-to-disc ratio
Increased pattern standard deviation on static threshold perimetry
Established
Elevated IOP
Over 18 mmHg any of the time
Increased fluctuation of IOP
Increasing age
Exfoliation of the lens capsule
Advanced cupping
Advanced visual field loss
Putative
Sleep apnea
Thin corneas
Nocturnal systemic hypotension
In approaching the treatment of someone who has glaucoma or who is at such high risk for its development that treatment is in order, many issues have to be considered. Among these are the level of IOP at which damage has occurred (or is likely to occur), the age of the patient, the degree of cupping and visual field loss, the presence of secondary features such as signs of exfoliative disease or pigmentary dispersion, systemic conditions or medications that might intereact with glaucoma progression or treatment, social issues such as presence or absence of support systems, and economic issues. Most physicians today try to tailor therapy to the individual patient and his or her situation. Many find it useful to estimate and then aim for a ‘target pressure.’
TARGET PRESSURE
The concept of target pressure arose from the observation that progression in advanced glaucoma, and occasionally even in early glaucoma, often occurs at what are thought to be ‘physiologic’ pressures. An IOP of 21 mmHg or lower – the previously sought goal – may not be low enough for many glaucomatous eyes. This observation was supported by the findings of the AGIS study which showed that the risk of progression was greatly reduced if all IOPs were under 18 mmHg. The goal should be to lower the IOP to a level that is ‘safe’ for that particular eye. Because our current knowledge does not indicate with any certainty what a safe IOP level may be for any given patient, the target pressure is estimated for each patient based on initial IOP, degree of existing damage, how hard the patient has to work to achieve the goal, the realities of adherence expectations for that invidual, and what potential side effects, complications, and cost that particular regimen might entail. The target pressure is then continually reassessed and reset based on the clinical course.
The less the initial pretreatment IOP, the more advanced the optic nerve damage, and the older the patient, the lower the target pressure should be set. The presence of a vasculopathy such as diabetes or arteriosclerotic cardiovascular disease should also lower the target pressure. A young person with glaucoma secondary to trauma with a pretreatment IOP of 32 mmHg and a 0.5 cup-to-disc ratio with early visual field loss may do quite well with an initial target IOP in the low 20s. An elderly patient with a pretreatment IOP of 22 mmHg, a cup-to-disc ratio of 0.9, and advanced visual field loss may require a target pressure of 15 mmHg or lower.
The currently available approaches to lower IOP include medical therapy, laser trabeculoplasty, filtering surgery, and cyclodestructive procedures – each of these has its own risk:benefit ratios. Whichever method is chosen, it should ideally be predictable, safe, and easy for the patient to use. Unfortunately, there is no ideal treatment for glaucoma. Because the risk:benefit ratio for medical therapy seems to be lowest, both historical and current practice has been to attempt medical treatment before resorting to other alternatives. However, this approach has been called into question in recent years.
MEDICAL THERAPY
ADVANTAGES
Medical therapy has been the mainstay of initial glaucoma treatment for over a century. The vast majority of patients respond to a simple regimen with a desirable reduction in IOP. Side effects are usually few and tolerable. When they do occur, side effects are often easily reversible by stopping the medication. Only rarely are vision- or life-threatening side effects seen. Medical therapy is less costly over the short term, and because many glaucoma patients are elderly, the cost of medical treatment may never exceed that of surgery. Finally, most patients expect a trial of medical therapy first and are likely to be somewhat suspicious of a too rapid suggestion for surgery.
DISADVANTAGES
Over the last decade, the disadvantages of medical therapy have been increasingly highlighted. Several prospective studies have pointed to medical therapy as less effective in lowering IOP and perhaps in preventing further visual field loss than either laser trabeculoplasty or filtering surgery. However, some of the differences between the medically and surgically treated groups in these studies may be the result of not setting the target IOP low enough in the medically treated group (the surgically treated groups had pressures ‘automatically’ set at low levels). The Glaucoma Laser Trial showed that after 2 years, only 30% of patients initially treated with medications were still being controlled with a single topical β-blocker. The Collaborative Glaucoma Initial Therapy Trial at the end of 5 years showed no difference in the progression of visual field loss with either medication as initial therapy in glaucoma or trabeculectomy. While quality of life issues were similar in both groups, the medication group had more local eye symptoms and those in the surgery group were more likely to develop cataract and reduced visual acuity early. However, by 5–8 years, cataract incidence and severity was again even in the two treatment groups.
Medically treated eyes may not have as good a success rate from subsequent filtering surgery compared with eyes treated with surgery from the outset. This may be due to an increased number of inflammatory cells and a decreased number of goblet cells in the conjunctiva of medically treated eyes compared with eyes treated initially with surgery. Medical treatment has the potential for significant and serious side effects, not all of which may be obvious to the patient or physician. During an 8-year period (1978–1985) in the United States, 32 deaths (of about 2 million glaucoma patients) were attributed to complications arising from the use of topical timolol maleate. Carbonic anhydrase inhibitors also can produce rare fatal reactions.
Less dramatic side effects of topical medications include allergic reactions, toxic corneal changes, induced refractive errors, cataract formation, asthma, tachycardia, bradycardia, orthostatic hypotension, gastrointestinal symptoms, decreased libido, impotence, mood changes, possible memory loss, and alopecia. Systemic carbonic anhydrase inhibitors are notorious for significant side effects such as lethargy, depression, diarrhea, and loss of appetite. All of these may have subtle or profound effects on the patient’s quality of life.
In addition, many older patients are also taking a number of other medications, which may add to the potential for cross-reaction and confusing side effects. Patients and their primary care physicians often do not associate systemic side effects with topical eye medications, and the ophthalmologist may fail to ask about systemic symptoms; in these cases, the cause of a systemic problem induced by antiglaucoma medications may go undetected.
A further complication of antiglaucoma medications is their high cost. Retail prices for any one type of topical eyedrop may run as high as $80 for a month’s supply. If the patient is using multiple glaucoma medications, the cost of antiglaucoma therapy could well be over $120 per month. For someone whose only source of income is a modest pension or social security, this may mean choosing between eating and taking needed medication. Because glaucoma is a chronic, lifetime condition, the cost of medications over the long term may be astronomic, both personally and from a public health/medical economics point of view. This is a major factor in nations with limited resources and can be a significant factor even in the most wealthy countries.
Finally, the issue of compliance must be addressed. It is probably unrealistic to think that a typical elderly patient who is taking medications for hypertension, diabetes, arthritis, and hiatal hernia is going to be able to accurately stick to a regimen that includes two or three topical medications and a carbonic anhydrase inhibitor. Kass and co-workers have shown that compliance is surprisingly poor, even with just one medication used four times a day. Similar studies more recently have shown surprisingly low persistence rates even with once a day medication (prostaglandin or similar), although once daily dosing seems significantly better from a persistence point of view than medications requiring more frequent dosing during the day.
SURGICAL THERAPY
ADVANTAGES
As noted previously, surgical treatment (either laser trabeculoplasty or a filtering operation) is more likely than is medical treatment to keep IOP ‘under control’ and at a lower level for a longer period of time. Visual function may be better preserved with surgery than with medical therapy but visual acuity is more immediately affected negatively. Quality of life in the long run may be affected less negatively with surgery because fewer or no medications are likely to be necessary after a short postoperative period. Surgery is also less dependent in the long run on compliance than is medical therapy. Finally, the cost of surgery, assuming no complications, may actually be less than the cost of medication if the positive effects of the surgery last at least 8 years.
DISADVANTAGES
Surgery is irreversible. The Rubicon is crossed, and the eye cannot be returned to its original status. Patients whose surgical intervention is successful are often quite happy to no longer require medications, but those who have a major complication are usually much more unhappy than patients who never had surgery. Significant vision- and life-threatening complications can occur from surgery. Such complications include respiratory arrest and cardiovascular failure from retrobulbar anesthesia, perforation of the globe, suprachoroidal hemorrhage, maculopathy from hypotony, corneal decompensation, cataract formation, and postoperative endophthalmitis. Visual distortions may occur due to induced astigmatism, cataract, or large iridectomy. Prolonged postoperative discomfort may occur from a large bleb, dellen, or surgically-induced ptosis. A large bleb or iridectomy may be a cosmetic problem for some patients. Each episode of conjunctivitis, normally a minor annoyance, becomes a major medical emergency for a patient with a filtering bleb. Cosmetic contact lens use in the presence of a filtering bleb is usually inadvisable. Yet, in the Collaborative Initial Glaucoma Treatment Study (CIGTS) study, serious complications from initial trabeculectomy were uncommon.
In the Glaucoma Laser Trial, at least 40% of those treated initially with laser surgery needed supplemental medical therapy by 2 years after the laser treatment. The beneficial effect of laser treatment seems to be time limited and is frequently gone by 3–5 years after surgery. Finally, none of the prospective studies on initial laser or surgical treatment of glaucoma had follow-up beyond 5 years at the time of this writing. This is a short period compared with the lifetime nature of glaucoma to measure the true effect or actual longevity of the surgical approach. The advantages and disadvantages of medical and surgical treatment are presented in Tables 22‑1 and 22-2 .
Medical therapy | Surgical therapy |
---|---|
Most patients are easily controlled Serious side effects are rare Side effects are usually tolerable Side effects are usually reversible Costs are reasonable over short haul | Intraocular pressure likely to be lower than with medical treatment Visual function may be better preserved Quality of life may be better than with medical treatment Less dependent on patient compliance Costs less over long haul |
Medical therapy | Surgical therapy |
---|---|
Less effective in lowering intraocular pressure than surgery Medical treatment tends to escalate with time May interfere with success of filtering surgery Potential for serious side effects Eye medications are not always identified as cause of side effects Potential for cross-reaction with systemic medications Nuisance factor and side effects may interfere with quality of life Long-term costs may be high Compliance often poor | Irreversible damage to eye or vision possible Death or serious illness possible Complications can occur late (e.g., endophthalmitis, hypotony, leak) Effects may not last for life Contact lens use limited Medical therapy may be needed anyway Patient disabled during initial operative and postoperative period Initial costs high Long-term follow-up lacking Surgery may have to be repeated |
BASIC PHARMACOLOGY
Most drugs used for glaucoma therapy are administered topically to the eye. Before discussing these drugs individually, some of the general pharmacologic principles of topical ocular treatment should be considered.
To be effective, a drug must penetrate the eye and achieve an adequate concentration at its site of action. The eye is protected by a number of mechanisms (e.g., blinking, tear flow, active transport, blood–aqueous and blood–retinal barriers), however, that limit exposure to endogenous and exogenous noxious agents. These same protective mechanisms make it difficult to reach and maintain an effective intraocular drug concentration. This problem is compounded by the structure of the eye itself, which isolates ocular tissues from the ocular and systemic blood circulation and from the ocular surface. One of the major problems in ocular therapeutics is achieving the desired effect of topical medication despite these protective mechanisms and structural obstacles (i.e., achieving an adequate concentration of drug at the site of action without creating potentially dangerous concentrations elsewhere).
The usefulness of a drug is determined by its efficacy, potency, duration of action, and therapeutic index. Efficacy is the maximum therapeutic effect obtainable. Potency is defined as the dose producing 50% of the maximum drug effect, usually expressed as E50 or an I50. Duration of action is the length of time a drug dose exerts a biologic action. Therapeutic index is the ratio of the dose of a drug producing a toxic effect divided by the dose producing the desired effect.
Therapeutic index = Dose of a drug producing a toxic effect Dose of a drug producing the desired effect
The physician seeks drugs with a high therapeutic index (e.g., 10 or higher if possible). However, an acceptable therapeutic index depends on the nature of the drug and the nature of the disease being treated. Physicians use different standards when choosing chemotherapy for cancer than they do when choosing eyedrops for minor ocular irritation.
Bioavailability refers to the rate and extent of absorption across a surface or tissue. The bioavailability of topical medication is considered in the following pages under the headings of tear film, corneal barriers, drug formulation, and drug elimination ( Box 22-3 ).It is important to emphasize that the most important factors limiting the bioavailability of many common topical ocular medications are compliance and efficiency of instillation. Discussion of these issues follows.
- I.
Tear factors
- A.
Rate of tearing
- B.
Punctal occlusion
- C.
Nasolacrimal drainage
- D.
Orbicularis function (eyelid squeezing)
- E.
Dilution with a second drug
- A.
- II.
Corneal factors
- III.
Drug and formulation factors
- A.
Concentration and volume instilled
- B.
Solubility characteristics
- C.
Dissociation constant
- D.
Molecular weight
- E.
pH
- F.
Tonicity
- G.
Electrolyte composition
- H.
Wetting agents/preservatives
- I.
Viscosity
- J.
Buffers
- K.
Vehicle or delivery system
- A.
- IV.
Drug elimination
- A.
Tear flow
- B.
Diffusion into the vascular system
- C.
Diffusion from the cornea to the tear layer
- D.
Bulk flow of aqueous humor
- E.
Metabolism
- F.
Active transport
- G.
Binding to melanin
- H.
Binding to protein
- A.
- V.
Miscellaneous
- A.
Genetic variation in ocular structure and function
- B.
General metabolic factors
- 1.
Blood flow
- 2.
Hormonal regulation
- 3.
Neural regulation
- 4.
Availability of nutrients
- 5.
Age
- 1.
- C.
Drug-related factors
- 1.
Local and systemic side effects
- 2.
Drug–drug interaction
- 3.
Tolerance
- 4.
Compliance
- 1.
- A.
- VI.
Disease factors
- A.
Inflammation
- B.
Intactness of epithelium
- C.
State of blood–aqueous barrier and blood–retinal barrier
- D.
Aqueous humor and tear protein concentrations
- A.
Modified from Bergamini MVW. In: Drance SM, Neufeld AH, editors: Glaucoma: applied pharmacology in medical treatment, New York, Grune & Stratton, 1984.
BIOAVAILABILITY OF TOPICAL OCULAR MEDICATION
The penetration of topically applied medication into the eye is proportional to the concentration of the drug that comes in contact with the cornea over time. For many drugs this relationship holds true over a wide range of concentrations (e.g., 10 −6 to 10 −1 M for pilocarpine). The drug concentration in contact with the cornea is diluted by tears and washed out into the lacrimal drainage system. The half-life of various drugs in the tear layer is estimated to be 2–20 minutes. Alteration of the molecule or vehicle in a way that increases the half-life, such as increasing the viscosity, will increase the efficacy and duration.
The normal volume of the conjunctival cul-de-sac is 7 μl. After instillation of an eyedrop, the volume temporarily increases to 30 μl. Most commercial eyedrops have a volume of 30–75 μl. Thus even a single drop exceeds the capacity of the cul-de-sac, so that the excess drains onto the skin and into the lacrimal system. Some investigators have postulated that the relative bioavailability of many topical medications could be increased by decreasing the volume of the eyedrops to 5–20 μl.
TEAR FILM
Under normal circumstances the turnover rate of the tear film is 15% per minute ; this depends on the rate of tearing rather than the capacity of the lacrimal drainage system. The instillation of eyedrops stimulates tearing and increases the turnover rate to 30% per minute. Eyedrop instillation also causes contraction of the orbicularis muscle (e.g., blinking, squeezing), which propels tears toward the lacrimal drainage system. It is possible to reduce the flow of tears and medication into the lacrimal system and prolong drug–cornea contact time by placing pressure over the canaliculi and closing the eyelids gently after administering eyedrops.
Drugs that pass into the nasolacrimal system can be absorbed rapidly by the heavily vascularized mucosa of the nasopharynx and oropharynx. Drugs absorbed in this manner do not pass through the gastrointestinal tract and the liver and are not metabolized by these tissues (i.e., there is no first-pass effect). Thus drugs absorbed from the nasolacrimal passages can act as if they have been given by intravenous injection rather than oral administration. For this reason the instillation of multiple eyedrops over a short interval is more likely to produce increased systemic absorption and side effects than increased therapeutic benefit.
If saline solution is instilled in rabbits within 30 seconds of pilocarpine administration, the effect of pilocarpine on pupil diameter is reduced by 45% ( Fig. 22-1 ). If saline solution is administered within 2 minutes, pilocarpine’s effect is diminished by 17%. If saline solution is added after 5 minutes, there is little loss of response to pilocarpine. Patients should be warned about this washout effect.

CORNEAL BARRIERS
Most topically administered drugs enter the eye by passing through the cornea. (The movement of drugs across the conjunctiva and sclera accounts for less than 2% of the intraocular concentration.) This process usually occurs by passive diffusion and follows first-order kinetics (i.e., the absorption depends on the drug concentration gradient, the solubility characteristics of the substance, and the dissociation constant for ionizable substances). Thus the rate of entry is initially high but declines rapidly.
To understand drug absorption, it is useful to think of the cornea as a lipid–water–lipid sandwich. The lipid content of the epithelium and endothelium is approximately 100 times greater than that of the stroma, and thus these former layers are more permeable to lipophilic than to hydrophilic substances. The corneal epithelium is the major barrier to drug penetration except for the most lipid-soluble substances. When the epithelium is disrupted (e.g., by irritation, ulcer, or abrasion), drug penetration is often increased. The corneal stroma is more permeable to hydrophilic than to lipophilic substances. Because of the dual nature of the corneal barriers, drugs possessing both lipid and water solubility penetrate the cornea more readily.
Many topical ophthalmic medications are weak acids or bases. The non-ionized drug molecule traverses the epithelium and then dissociates. The ionized form of the drug passes through the stroma more readily and then associates for passage through the endothelium.
Many topically administered medications accumulate in the cornea, which then becomes a temporary drug reservoir. When the medication concentration in the tears falls to low levels, there is a net movement of drug from the cornea to the tear layer. After absorption, many medications distribute rapidly and reach similar concentrations in the aqueous, iris, and ciliary body. Drug concentrations are usually lower in the lens and vitreous. Although there is controversy, most studies indicate that medications do not pass against aqueous flow from the anterior chamber through the pupil into the posterior chamber. Under some circumstances, however, certain agents such as topically applied epinephrine may get into the vitreous. Drugs may reach the ciliary body by following the uveoscleral flow of aqueous humor.
DRUG FORMULATION
A number of properties of a drug and its formulation (e.g., molecular weight, pH, tonicity, electrolyte composition, wetting effect, stability, viscosity) influence ocular penetration. Most topical ocular drugs have a low molecular weight (i.e., less than 500 Da), which favors corneal passage. As mentioned previously, many topical medications are weak acids or bases. These drugs are often more stable at non-physiologic pH levels (e.g., pilocarpine is more stable at pH 6.5 than at pH 7.4). Eyedrop solutions are adjusted to this pH but are not buffered so that the tear layer can return rapidly to physiologic pH levels. This ensures drug stability and prevents excessive irritation, which leads to tearing on instillation, diluting and washing away the drug.
Ocular penetration increases with increasing concentration of the drug in the eyedrop. However, if the drug concentration in the eyedrop is too great, the resulting increase in osmolality pulls fluid across the conjunctiva, diluting the medication in the tear film. The ocular penetration of many topical medications is aided more by prolonging drug–cornea contact time than by increasing the concentration of the eyedrop. A number of systems have been used to prolong drug–cornea contact time and to enhance pulsed entry of medication into the eye. These include presoaked contact lenses, the addition of soluble polymers to eyedrop solutions, soluble gels, aqueous suspensions, ointments, solid hydrophilic inserts, binding to polymers, and binding to liposomes. A viscous vehicle can increase ocular drug penetration by 50–100%. The increased viscosity produces more rapid drug saturation and slower washout of medication by the tears.
Water-soluble polymers are commonly used to increase drug–cornea contact time. Currently used polymers have been assessed with respect to corneal retention time or delivery of a marker compound and are listed in order of decreasing effectiveness: hydroxypropyl cellulose, hydroxyethyl cellulose, hydroxypropyl methylcellulose, and polyvinyl alcohol. However, the effect of water-soluble polymers on drug penetration appears to be greater in rabbit eyes than in human eyes because rabbits have a lower blink rate and a slower tear turnover.
Aqueous suspensions contain a drug dispersed as fine particles. The vehicle, often combined with a dispersion agent, is a saturated solution of the sparingly soluble drug. Tissue absorption occurs from the solution. As tearing dilutes the medication, the suspended drug particles go into the solution. The particles are retained longer in the cul-de-sac than are standard solutions and thus provide greater drug bioavailability. Ointments increase drug bioavailability by increasing drug–cornea contact time, decreasing dilution by tears, and slowing nasolacrimal drainage. Ointments are not used frequently, however, because they are difficult to instill and interfere with vision.
It is also possible to prolong drug–cornea contact time with systems that produce a controlled (i.e., steady) release of medication. Eyedrops produce a pulsed or biphasic pattern of drug delivery consisting of a transient overdose followed by a prolonged underdose. The initial overdose can be associated with systemic side effects. Controlled release requires a system that delivers medication with zero-order kinetics (i.e., drug delivery is independent of the amount of drug remaining). Therefore the rate of medication delivered remains constant until the supply is exhausted. Controlled-release systems have several potential advantages, including achieving a therapeutic effect with less medication, reducing side effects, and placing less reliance on patient compliance. The Ocusert system, the best example of this approach in ophthalmic therapy, uses a membrane that allows continuous diffusion of pilocarpine from a central reservoir.
Preservatives added to eyedrop solutions often increase drug penetration. For example, the therapeutic effect of carbachol is enhanced greatly by the presence of benzalkonium chloride, which acts both as a preservative and a wetting agent. However, evidence exists that the effect of preservatives may be mediated by structural changes in the cornea and conjunctiva that are not necessarily favorable.
DRUG ELIMINATION
The bioavailability of a drug at its active site within the eye depends on many processes besides the rate of transcorneal penetration. These include drug metabolism, active transport out of the eye, binding to melanin and protein, diffusion into the vascular system, and bulk flow with aqueous humor. Most of the drug loss occurs soon after instillation in the cul-de-sac because of overflow onto the face and drainage into the nasolacrimal system. Once in the anterior chamber, the drug is eliminated with the bulk flow of aqueous humor via both the trabecular and uveoscleral outflow pathways. Some portion of the drug also diffuses into the vascular system of the anterior uvea, conjunctiva, and episclera. The diffusional loss to the vascular system is greater in inflamed eyes with dilated, more permeable vessels. As mentioned previously, the corneal epithelium serves as a drug reservoir for many medications. Drug from the cornea is also lost to the limbal blood vessels and the tear film.
The eye contains a variety of enzyme systems capable of metabolizing drugs. Some drugs (e.g., pilocarpine and dipivefrin) are even metabolized during corneal passage. This metabolic capability is used by the prodrug dipivefrin, which is converted to its active agent, epinephrine, by esterase enzymes located in the cornea and aqueous humor. In addition to metabolism, active transport systems in the ciliary body and retina remove drugs from the eye (e.g., penicillin, indomethacin). These transport systems are similar to those found in the renal tubule and liver.
Many drugs (e.g., pilocarpine, timolol) bind to melanin pigment in the anterior uveal tract. This binding has a complex effect on drug bioavailability. On one hand, the melanin binding competes with the active site for the drug. Conversely, the melanin binding also creates a type of drug reservoir in the eye. The net effect of the binding process is to require higher concentrations of some drugs in pigmented eyes to produce the same therapeutic effect. It has been suggested that the difference in bioavailability of some drugs in pigmented eyes is not totally explained by melanin binding. The difference in bioavailability of some drugs also involves greater metabolism in pigmented eyes (e.g., the metabolic conversion of pilocarpine to pilocarpic acid is greater in pigmented rabbits). Drugs also bind to protein in the tears, cornea, and aqueous humor, thereby reducing bioavailability. Protein binding increases when the eye is inflamed and the blood–aqueous and blood–retinal barriers are compromised.
COMPLIANCE
No discussion of general pharmacologic principles would be complete without examining the issue of compliance or adherence which is the newer preferred term. Adherence refers to the patient’s behavior in following the prescribed regimen, including medications, follow-up visits, and lifestyle changes.
Most forms of open-angle glaucoma are chronic, slowly progressive, and asymptomatic; in fact, the only symptoms may arise from the therapy. Thus open-angle glaucoma is a fertile situation for patient defaulting. The physician should be very aware of the possibility that failure of medical treatment to meet the therapeutic goals may be caused, at least in part, by defaulting rather than by lack of efficacy of the drug regimen. Studies using eyedrop medication monitors have confirmed the longstanding suspicion that some glaucoma patients do not take their medication(s) as prescribed. In one study, 6% of patients administered less than one-quarter of prescribed pilocarpine doses, 15% less than one half of prescribed doses, and 35% less than three-quarters of prescribed doses. The corresponding figures for timolol are somewhat better but still indicate considerable room for improvement (i.e., 8% administered less than half of the prescribed doses, and 27% administered less than three-quarters of prescribed doses). Furthermore, patients sometimes compress doses during the day and skip entire days (i.e., take drug holidays); 24% of patients have at least 1 day per month in which they take no doses of pilocarpine, and 47% have at least 1 day per month in which they take no doses of timolol. It is likely that defaulting also occurs with epinephrine or dipivefrin treatment and is even more common with the systemic carbonic anhydrase inhibitors. Poor compliance may account for as much as 10% of the vision loss seen in glaucoma.
Patients usually remember to take their medication on the day they return to the office or clinic. This leads to IOP readings that may be misleading and not truly reflective of the patient’s status over the past several weeks or months. This observation may explain some of the cases of progressive glaucomatous damage despite what appears to be good IOP control. Patients will often not admit to poor compliance even if directly asked. Patients who admit they forget to take their medications generally are telling the truth; those who swear they never miss a dose may or may not be telling the truth. No matter how well they think they know their patients, physicians are unable to identify those who are defaulting from antiglaucoma treatment.
Somewhat surprisingly, compliance (or lack thereof) is not associated with age, marital status, socioeconomic level, race, occupation, education level, or intelligence level. However, increased frequency of missed appointments, lack of understanding of the disease, dissatisfaction with physician or clinic, increased waiting times to see the physician, disinterest in one’s health, and unstable family condition are associated with increased rates of poor compliance. Interestingly, even an excellent understanding of glaucoma and its potential for blindness does not guarantee good compliance; in one study, only two-thirds of the glaucomapatients who seemed to have an adequate understanding of their condition were compliant. Conversely, of those in the samestudy who did not understand their condition, only one-third were compliant.
From 3% to 7% of patients do not even fill the prescriptions given to them by the physician. Many do not understand the chronic nature of glaucoma therapy and may take the drops only for some fixed period such as a month or until the first bottle runs out. The continued use of a prescribed medication is referred to as persistence . As it is with most asymptomatic, chronic diseases, the persistence with glaucoma medications is surprisingly poor with, at best, only about 50% still refilling their prescriptions at 1 year. Two recent surveys of large medication insurance plans show that persistence is best (although not terrific) for the prostaglandin group of agents and significantly poorer with the rest of the antiglaucoma agents.
Another problem relates to the fact that patients do not always know a satisfactory technique for administering eyedrops. This is particularly true of ointments. The doctor often assumes (incorrectly) that the patient will somehow, perhaps intuitively, know how to administer drops or ointments to themselves. Patients with poor vision may not be able to distinguish the different eyedrop containers without color-coded caps or other markings. Elderly patients or those with difficulty using their hands may not be physically able to administer drops to themselves or may have great difficulty with some bottle designs. The small bottles associated with prostaglandin analogs may be particularly difficult for those with arthritic fingers. Patients may assume that if one drop is good, then two or even more may be better. Patients with busy or erratic lifestyles may not be able to keep to a regular dosing schedule.
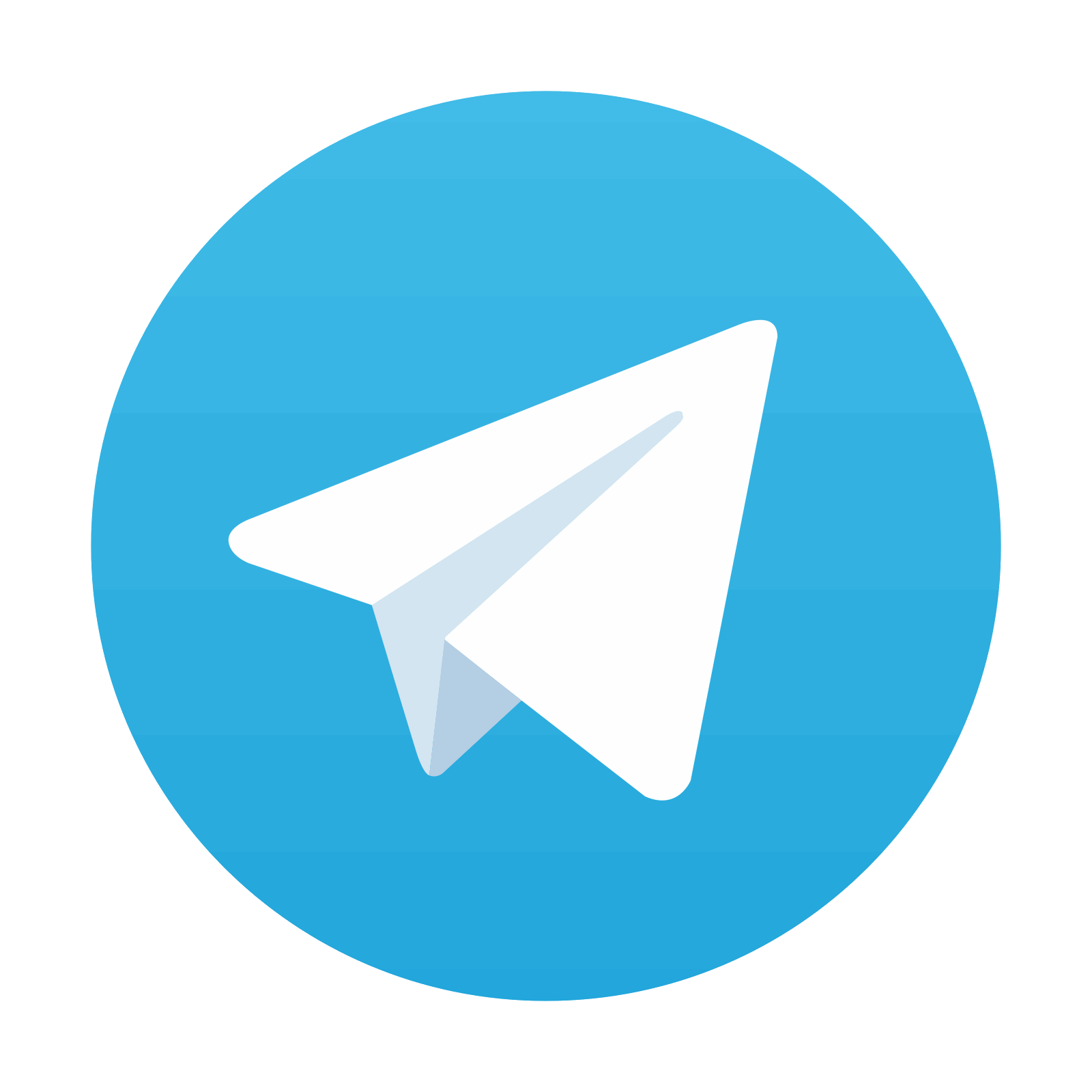
Stay updated, free articles. Join our Telegram channel

Full access? Get Clinical Tree
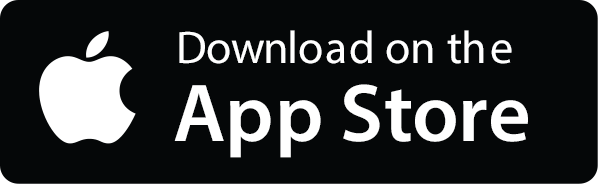
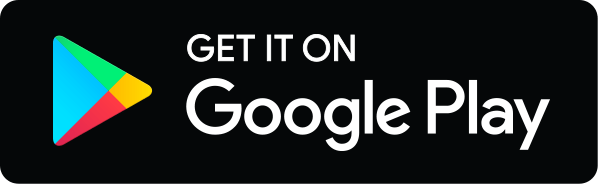