Introduction
The potentially damaging effects of light on the retina have been recognized since the time of Plato, who described eclipse blindness. Breakdown of the intrinsic ocular protective mechanisms and/or exposure to external high-risk conditions can produce light or photic damage to the retina. The development and severity of light-induced damage depends on a number of factors, including ocular anatomical protective mechanisms, area of tissue involved, and the parameters of the light source, such as the wavelength, duration of exposure, and the total energy exposure.
Light Interaction With the Retina
The eye primarily perceives radiation in the optical spectrum, comprising the visible (400–760 nm), ultraviolet (UV; 200–400 nm), and infrared (IR; >760 nm) wavelengths. Radiation in this region can be produced by the sun, ophthalmic instruments, and lasers.
Tissue effects produced by light are classified as mechanical, thermal, or photochemical and determined by irradiance (watt per square centimeter [W/cm 2 ]), wavelength, duration of light exposure and the absorption of target tissue. Table 6.10.1 outlines tissue effects induced by commercial ophthalmic lasers. Mechanical injury results from high irradiance, short-duration exposure in the nanosecond (10 −9 ) to picosecond (10 −12 ) range, and the energy strip electrons from molecules and disintegrates target tissue into plasma. This is the mechanism of photodisruption. At moderate irradiance and exposure greater than 1 microsecond (µsec), thermal effects result from a critical temperature rise in tissue. Elevation of retinal temperature by 10–20°C produces protein denaturation and enzyme inactivation, which results in photocoagulation, cellular necrosis, and hemostasis. Long visible and infrared (IR) wavelengths produce thermal injury to the retina and choroid during laser photocoagulation. Photochemical or phototoxic effects occur with low-to-moderate irradiances that are below coagulation thresholds and with shorter wavelengths, in particular UV and visible blue wavelengths. Damage to cellular components occurs at temperatures too low to cause thermal destruction, which may account for a delay of 24–48 hours before the appearance of a lesion. Absorption of a photon by the outer electrons of a molecule produces an excited molecular state, which can drive a chemical reaction. Because the energy per photon is inversely proportional to its wavelength, short-wavelength photons have more energy with which to induce a photochemical reaction. Long-wavelength visible light also can induce photochemical changes when tissues are sensitized by an exogenous photosensitizer, as with photodynamic therapy. At intermediate values of irradiance and exposure, more than one of the above mechanisms may be in effect.
Laser Modality | Mechanism of Damage |
---|---|
Argon laser | Photocoagulation |
Transpupillary therapy (TTT) | Photocoagulation |
Photodynamic therapy (PDT) | Photochemical injury |
Neodymium:yttrium–aluminum–garnet (Nd:YAG) laser | Photodisruption |
The ocular media transmit 75%–90% of electromagnetic radiation in the range of 400–1064 nm. Several mechanisms exist to reduce retinal light exposure. The cornea absorbs most UVB (280–315 nm) and UVC (<280 nm), as well as some IR radiation, and reflects up to 60% of incident light that is not perpendicular to its surface. The lens absorbs most UVA (315–400 nm) and visible blue wavelengths. Intrinsic ocular defenses against retinal light damage include xanthophyll absorption of near-UV and blue light to protect photoreceptors, temperature control by the choroidal circulation, intracellular molecular detoxification of free radicals, and retinal pigment epithelium (RPE)–mediated photoreceptor renewal. Physiological protective mechanisms include squint and blink reflexes, eyebrow ridge, aversion response, and pupillary miosis. Light damage to the retina may occur when protective mechanisms are impaired or as a result of deliberate gazing at a light source. Young patients may be at increased risk because of efficient light transmission through clear ocular media.
Photic Retinopathy
Photic retinopathy is a general term for light-induced retinal damage. It is most often caused by inadvertent exposure. The mechanism is typically photochemical, with potential enhancement by elevated tissue temperature and increased blood oxygen tension. Increased chorioretinal pigmentation facilitates light absorption in the RPE and may elevate the background retinal temperature and thermally enhance photochemical damage. It has been hypothesized that retinal defenses against toxic free radicals from light and oxygen are overwhelmed by supranormal light exposure. Damage manifests as a disorder of RPE and photoreceptor outer segments. Retinal phototoxic injury was originally considered permanent; however, visual recovery has been noted in solar retinopathy, laser pointer, welding arc, and operating microscope phototoxicity. Mild photochemical damage may not be symptomatic or visible ophthalmoscopically, and case reports represent the more severe injuries. The extent of retinal injury and the likelihood of visual recovery depend on multiple factors, including the location and area of exposed retina, duration, intensity, spectrum of the light source, and host susceptibility factors, including age, nutritional status, ocular pigmentation, core temperature, clarity of ocular media, and pre-existing retinal disease. Individuals with emmetropia and hyperopia may be at increased risk caused by effective light focusing on the retina. Systemic photosensitizing agents, such as tetracycline and psoralen, may predispose to photochemical damage.
Solar Retinopathy
Solar retinopathy, which describes retinal injury induced by direct or indirect solar viewing, is also referred to as foveomacular retinitis, photoretinitis, and eclipse retinopathy . The harmful effects of solar viewing have been recognized for centuries. Foveomacular retinitis was initially described as bilateral decreased vision and foveal lesions in military persons after solar viewing. Solar retinopathy has been associated with religious sun gazing, solar eclipse observing, telescopic solar viewing, sunbathing, psychiatric disorders, and psychotropic drug use. Solar radiation induces photochemical retina damage, which may be enhanced by elevated tissue temperature. Direct solar observation through a 3-mm pupil produces a 4°C temperature rise, which is below thermal damage thresholds. Sustained solar viewing for more than 90 seconds through a constricted pupil exceeds the threshold for photochemical retinal damage. Solar observation through a dilated 7-mm pupil produces a 22°C increase in retinal temperature, which is above photocoagulation thresholds.
Symptoms usually develop 1–4 hours after solar exposure and may include unilateral or bilateral decreased vision, metamorphopsia, scotomata, chromatopsia, photophobia, afterimage, and periorbital ache. Acuity ranges from 20/40–20/200 acutely. A small yellow spot with a gray margin measuring up to 200 µm may develop in the parafoveal area corresponding to the image of the sun ( Fig. 6.10.1A ). A lesion may not be visible in mild cases. Histopathology of acute solar retinopathy demonstrates RPE injury with necrosis, detachment, irregular pigmentation, and minimal photoreceptor damage. Fluorescein angiography (FA) may be normal or reveal RPE transmission defects (see Fig. 6.10.1B ). The yellow lesion is replaced by focal depression with RPE mottling or lamellar hole and vision usually improves to 20/20–20/40 within 6 months, although scotomata or metamorphopsia can persist. Optical coherence tomography (OCT; see Fig. 6.10.1C ) demonstrates disrupted reflectivity in the outer retina and ellipsoid layer. Ultrastructural findings in experimentally induced solar retinopathy have demonstrated parafoveal cone and rod outer segment changes and scattered RPE degeneration. The good visual prognosis has been attributed to the resistance of the foveal cones to photochemical damage.




Eclipse retinopathy describes similar macular damage after solar eclipse viewing. Evaluation of visual morbidity associated with the full solar eclipse on August 11, 1999, demonstrated abnormal macular appearance in 84% of symptomatic patients with rare cases of persistent symptoms. Excessive light exposure in rats has been shown to induce irreversible neuronal apoptosis of retinal cells, which may account for permanent visual impairment as well as gliovascular responses, which may be responsible for the transient clinical symptoms.
No specific therapy exists for solar retinopathy. Further episodes of solar viewing should be discouraged. Eclipse viewing without proper protective eyewear with tested solar filters should be discouraged. Oral corticosteroids have been used to treat acute lesions, but their beneficial effect has not been demonstrated conclusively because vision often improves spontaneously.
Welding Arc Exposure
Welding arcs emit radiation, and the most common injury is keratitis caused by corneal absorption of UV rays. Retinal injury is rare but can occur after a welding arc viewing without proper ocular protection. The retinal temperature increase is below photocoagulation thresholds, and injury is produced by photochemical effects from UV and short blue wavelength exposure. The symptoms and clinical course are similar to solar retinopathy. A yellow edematous foveal lesion develops acutely ( Fig. 6.10.2 ), which is replaced over time by RPE irregularity or lamellar hole. No effective therapy exists, and vision usually improves with time, with permanent vision effects being rare.


Phototoxicity has also been described following brief incidental light exposure from camera flashes or welding arcs in four patients who were taking photosensitizing drugs (hydrochlorothiazide, furosemide, allopurinol, and benzodiazepines).
Lightning Maculopathy
Lightning maculopathy describes acute visual loss and macular changes after lightning injury. Vision loss may be severe to light perception and lesions may include macular edema, macular hole, cyst, or a solar retinopathy–like picture, cataract, retinal detachment, retinal artery occlusion, and relative afferent pupillary defect. Visual recovery often occurs over time, even with severe maculopathy. High-dose intravenous methylprednisolone treatment may play a role in vision recovery, and its use was associated with reversal of lightning-induced blindness in two cases.
Retinal Phototoxicity From Ophthalmic Instruments
Ophthalmologists use a variety of powerful light sources. Retinal injury caused by light exposure from the operating microscope and endoillumination has been described. Iatrogenic phototoxicity has been reported after cataract extraction, combined anterior segment procedures, and vitreous surgery. The most frequent cause is the operating microscope as initially described after uncomplicated extracapsular cataract extraction, with reported incidence ranging from 0% to 7%. The mechanism of intraoperative phototoxicity is photochemical, with potential thermal enhancement. Because operating microscopes generate little UV radiation, photochemical damage probably is caused by short-wavelength visible blue and green light. The incorporation of UV and IR filters in the intraocular lens (IOL) and microscope may reduce, but do not completely eliminate, the risk of photic and thermal effects, respectively, as demonstrated by experimentally induced human photic retinal injury in a blind phakic eye after 60 minutes of operating microscope exposure with UV and IR filtered light.
Few patients show symptoms immediately after exposure, and the level of vision depends on lesion size and location. A foveal lesion can produce severe permanent vision loss, whereas an eccentric lesion is compatible with good acuity and pericentral scotoma. The lesion shape matches the surgical illuminating source. Immediately after exposure, there is little evidence of macular pathology but within 24–48 hours, a yellow lesion, measuring 0.5–2.0 disc diameter at the level of the RPE, with retinal edema develops. FA acutely reveals dye leakage ( Fig. 6.10.3 ), which may simulate choroidal neovascularization (CNV). Over weeks, the yellow lesion is replaced by RPE clumping and atrophy ( Fig. 6.10.4A ), which correspond angiographically to blocking and transmission defects, respectively (see Fig. 6.10.4B ). Other long-term sequelae include postoperative erythropsia, retinal surface wrinkling, and choroidal neovascularization. Mild light-induced retinal injuries may be overlooked clinically because subtle postoperative pigmentary changes may be attributable to other causes.




Histopathology of acute operating microscope–induced injury has revealed RPE and photoreceptor damage. In primates, early photic lesions have demonstrated photoreceptor damage and disruption of RPE tight junctions, with regeneration of photoreceptor outer segments 3–5 months after injury. This may correspond to recovery of vision after injury noted in some human eyes. Operating microscope phototoxicity has been associated with surgical factors, including microscope brightness, wavelength of light exposure, surgical duration, and technique. Retinal phototoxic lesions may occur after short-duration (under 30 minutes) phacoemulsification and were associated with near emmetropia and diabetic retinopathy. The risk of photic damage may increase after IOL insertion, which can focus incoming light on the retina. Patient susceptibility factors include increased body temperature and blood oxygenation, chorioretinal pigmentation, pre-existing maculopathy, pupillary dilatation, diabetes mellitus, retinal vascular disease, deficiencies of either ascorbic acid or vitamin A, and photosensitizer use. Photic damage secondary to endoillumination during vitrectomy is uncommon and can be avoided by minimizing length of surgery and light output, filters, maximizing light pipe distance from the retina and using eccentric endoillumination techniques.
There is no specific treatment for acute lesions, and spontaneous visual improvement usually occurs within months, even when lesions involve the macula. Methods to reduce the risk include reduction of coaxial illumination and operative time, use of IR and UV filters in the microscope and IOL, placement of an air bubble in the anterior chamber to defocus incident light, and use of a corneal cover.
The irradiance produced by the indirect ophthalmoscope and the fundus camera is lower than experimentally determined retinal injury thresholds. Furthermore, the total energy delivered to the eye is less under nonoperative conditions than in operative conditions. These instruments have not been shown to produce acute retinal injury in humans; however, prolonged exposure to the indirect ophthalmoscope has been shown to produce lesions in primates. The cumulative effect of repeated examination is unknown, and it is recommended that retinal examinations be performed with the minimal illumination required.
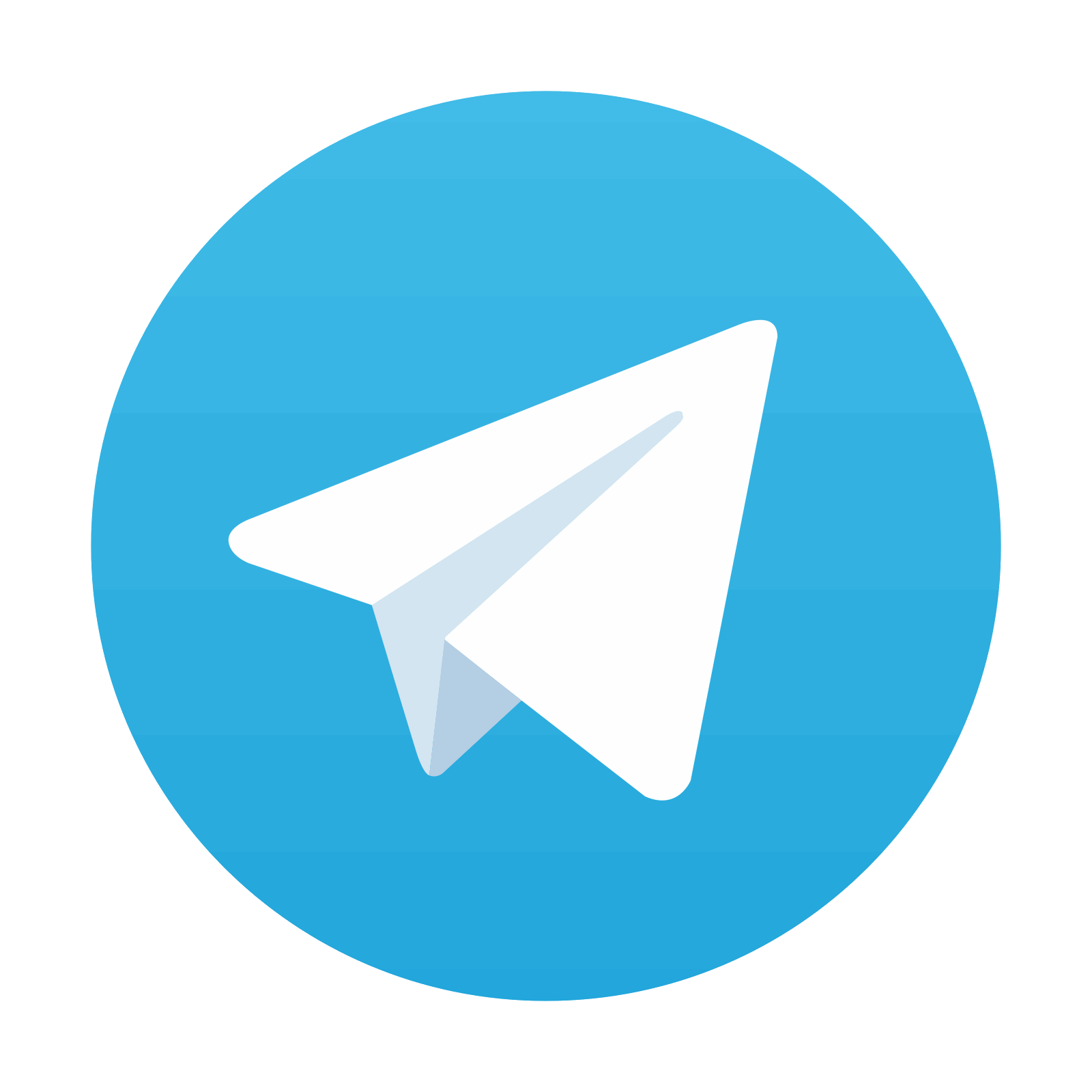
Stay updated, free articles. Join our Telegram channel

Full access? Get Clinical Tree
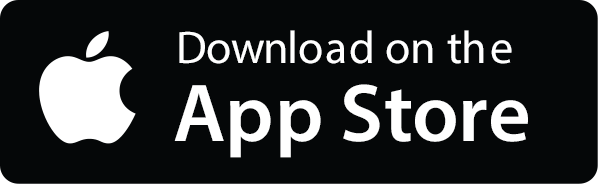
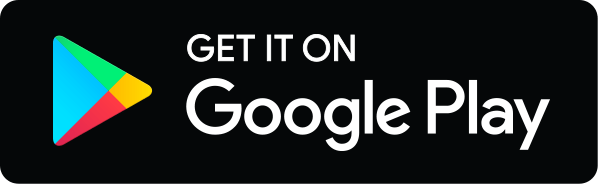