1
Vision from starlight to sunlight
The human visual system operates effectively over an enormously wide range of intensities, of at least a billion-fold, from around 10 −4 cd m −2 under starlight conditions to around 10 5 cd m −2 under intense sunlight. Changes in pupil area account for only about 1 log unit of this 9 log unit range, since the pupil diameter changes from a maximum of 8 mm to a minimum of 2.5 mm, corresponding to about a 10-fold reduction in area. Instead, the great bulk of the operational range is achieved by the combination of, firstly, a switch between the scotopic (rod-based) and photopic (cone-based) pathways in our duplex visual system and, secondly, the ability of each of these photoreceptor systems to operate over a range of 5 log units (100,000-fold) or more.
Light adaptation versus dark adaptation
This ability of the visual system (or of any of its component parts, such as a photoreceptor) to adjust its performance to the ambient level of illumination is known as “light adaptation”. This adjustment typically occurs very rapidly (within seconds), whether the light intensity is increasing or decreasing. The term “dark adaptation” is reserved for the special case of recovery in darkness, following exposure of the eye to extremely bright and/or prolonged illumination that activates (and thereby bleaches) a substantial fraction of the visual pigment, rhodopsin or its cone equivalent. Dark adaptation occurs slowly, and full recovery of the scotopic visual system after a very large bleach can take as much as an hour.
Light adaptation and the changes that accompany it are beneficial to the possessor of the eye. At very low intensities, visual sensitivity is increased to the utmost that is possible, so that the rod photoreceptors reliably signal the arrival of individual photons and the scotopic visual system operates in a photon-counting mode. This ability of the scotopic system to operate at incredibly low intensities is enhanced by two deliberate trade-offs that permit more reliable detection of small signals in the presence of noise: firstly, reduced spatial resolution (i.e. increased spatial summation), and secondly reduced time resolution (i.e. increased temporal integration). Similar trade-offs are used in the photopic system, so that as the ambient illumination decreases from daylight levels towards twilight levels, one’s spatial and temporal resolution deteriorate, making it very difficult to play fast ball-games when the light fades.
In contrast, the changes that characterize dark adaptation are disadvantageous. To be effectively blind to dim stimuli, for some considerable time after intense light exposure, cannot in any way be useful to an organism. Indeed, for our ancestors (as well as ourselves) entering a cave from bright sunshine is likely to have been quite dangerous, given that visual sensitivity is greatly reduced for tens of minutes. Why should such an apparently unsatisfactory situation have persisted? One possibility is that this slowness of recovery represents an inevitable “cost” of the evolutionary changes that were needed to enable the scotopic system to signal individual photon hits.
Purposes of light adaptation
A photoreceptor that did not adapt to the ambient light intensity would have a very narrow operating range: low intensities would provide negligible response, whereas high intensities would saturate the cell. Accordingly, photoreceptor light adaptation can be viewed as a means for extending the operating range of the cell.
The purpose of light adaptation can be viewed more generally as being to permit the visual system (or any neuron within it) to provide the best possible performance at that particular level of illumination; however, in this context it is not always clear what constitutes “best”. For example, it is difficult for us to specify the time-course of response that is best at any given level of illumination. A brief response is likely to provide a better reaction time for the organism, but it may result in very poor sensitivity; conversely, allowing the response to integrate for a longer period will provide greater sensitivity, but may result in a very poor reaction time.
In setting the best performance of a photoreceptor, one crucial task is to endow the cell with very high sensitivity at low light levels yet prevent it from saturating at higher light levels. Light adaptation accomplishes this by reducing the cell’s sensitivity to light as the background light level increases; however, this task needs to be performed in a manner that avoids excessive reduction in sensitivity. Cone photoreceptors excel in this capacity, and are able to avoid saturation no matter how intense the steady light becomes, and to continue to signal contrast effectively. Rods, on the other hand, are only able to adjust their sensitivity over a relatively narrow range of intensities, before they are driven into saturation, thereby becoming completely unresponsive. One advantage of this saturation of the rods, though, is that it substantially reduces the metabolic demand of the rods, at intensities where the cones are already functional, and where the metabolic demand for chromophore re-synthesis begins increasing.
In addition to the very important function of optimizing the photoreceptor’s sensitivity over a broad range of light intensities, there are two other ways in which photoreceptor light adaptation optimizes the cell’s response. Firstly, as will be explained below when eqn (1) is presented, Weber Law light adaptation permits automatic extraction of the contrast in the visual scene, independent of the absolute level of illumination. Secondly, light adaptation provides real-time adjustment of the time-course of the response to an incremental flash of light, in a manner that is presumed to be optimal for the visual system, though, as explained above, we are not yet able to quantify optimality in this regard.
2
Performance of the photopic and scotopic divisions of the visual system
Photopic vision: the cone system is the workhorse of vision
For humans, the photopic cone system can be considered the “workhorse” of vision, because it is operational under almost all of the conditions that we experience (in the 21st century). Thus, it is the photopic system that underlies our sense of vision at all light levels apart from the exceptionally low intensities experienced in moonlit and starlit conditions. Under moonlight levels of illumination, our scotopic and photopic systems are both functional, over an intensity range that is termed “mesopic”. In order to determine whether you are using your photopic system under twilight or nighttime conditions, there is a simple test: if you are able to detect any color in the scene, then your cones are active; if you cannot, then it is likely that only your rods are active. As the ambient intensity increases at dawn, the photopic system remains functional, up to the brightest sunlit conditions that we ever experience.
Despite their enormous importance to our vision, cones make up only about 5 percent of the population of photoreceptors. The low density of cone photoreceptors in the peripheral retina is quite adequate for our peripheral vision, even in daytime, as we require only relatively low spatial acuity in the periphery. Even though the great majority of peripheral photoreceptors are rods, they are not in fact used under most of the circumstances that we think of as vision – instead, they are only used at exceedingly low ambient lighting levels. The reason for having a very high density of rods is to be able to capture every available photon under starlight conditions.
The responses of cones are rapid and moderately sensitive
One of the greatest advantages of cones over rods is their much faster speed of response. Our rods, even when they are light-adapted, have responses that are much too slow to allow us to function visually at the speeds that are required to escape predators and to capture prey. Cones, instead, are specialized so as to permit extremely rapid signaling of visual stimuli to the brain.
Cones are often described as being far less sensitive than rods, but this view is misleading especially when considered in terms of the rapidly changing visual stimuli that the cones are specialized for signaling. Although a cone may exhibit a peak sensitivity to a brief flash of light that is perhaps 30-fold lower than in a rod, the sensitivity to rapidly fluctuating stimuli is considerably higher in cones than in rods; thus, the slow response of the rod makes it very insensitive to rapidly changing stimuli. When calculated in terms of the efficacy of activation in the G-protein cascade of phototransduction, the “amplification” in cones and rods appears to be essentially indistinguishable. The observed difference in sensitivity measured at the peak of the response to a flash instead stems from a difference in the speed of response inactivation.
Comparison of photopic and scotopic light adaptation
A classical result comparing light adaptation in the photopic and scotopic divisions of the visual system is illustrated in Figure 20.1A , from the work of Stiles. The threshold of a human subject for the detection of a flash is plotted against background intensity in double logarithmic coordinates. In this panel, the stimulus conditions provided a rod-based scotopic sensitivity that was only about 30× better than the cone-based photopic sensitivity – thus, the parafoveal region was tested with a small diameter yellow/green test flash (1° dia., 60 ms, 580 nm) on a green background. The measurements are well described by Weber Law curves, in both the scotopic (blue trace) and photopic (red trace) regions; see eqn (1) below. However, the “dark light” that will be described shortly is over 10,000× (4.1 log units) higher in the cone system compared with the rod system.

Scotopic vision: the rod system provides specialization for night vision
Light adaptation is examined under conditions that optimized detection by the scotopic (rather than photopic) system using the blue symbols of Figure 20.1B . To achieve scotopic dominance, the test stimulus was presented in the peripheral retina, and comprised a large area, long duration green flash (9° dia., 200 ms, 520 nm) on a red background. The measurements are from Fig. 3 of and their troland values have been converted using a factor of K = 8.6 photoisomerizations s −1 per troland.
For comparison, the red symbols plot the desensitization of primate rod photoreceptors, obtained from Fig. 9A and Table III in . Their measurements of sensitivity have been converted to desensitization by taking the reciprocal; in addition the symbols have been shifted vertically to provide a good fit to the psychophysical data in the high-intensity range.
The crucial difference between the blue and red symbols is that the overall scotopic visual system begins desensitizing at intensities around 1000 times lower than those required to begin desensitizing the rod photoreceptors. Thus, a considerable region at the lowest end of scotopic light adaptation is post -receptoral, rather than being internal to the rods. Because the post-receptoral scotopic system is able to integrate photon signals from large numbers of rod photoreceptors, and thereby gain increased sensitivity, it needs to begin desensitizing at much lower background intensities in order to avoid saturation. As a result the rod photoreceptors maintain their maximal sensitivity for several log units of the lowest-intensity regimen (up to ~10 isomerizations s −1 ) over which the visual system exhibits gradual desensitization.
When the background intensity is reduced from relatively high scotopic intensities (moving from right to left along the x-axis in Fig. 20.1B ) the desensitization of the rods, and of the scotopic visual system, steadily falls. However, below the intensities indicated by the red and blue arrows, the desensitization of first the rods, and second the visual system, fails to continue falling, as if the respective mechanism were experiencing a phenomenon equivalent to light. Accordingly, the arrowed intensities for the rods and for the scotopic visual system have been referred to as “equivalent background intensities”, or “dark light”. Clearly, the equivalent background for the scotopic system (around 0.016 photoisomerizations s −1 ) is more than 1000× lower than the equivalent background intensity for the rods (around 50 isomerizations s −1 ).
Each of the curves in Figure 20.1 plots desensitization according to Weber’s Law, though the curves in Figure 20.1B additionally include saturation at high intensities, as described by the equation:
D e s e n s D e s e n s D = { 1 + ( I / I 0 ) } exp ( I / I sat )
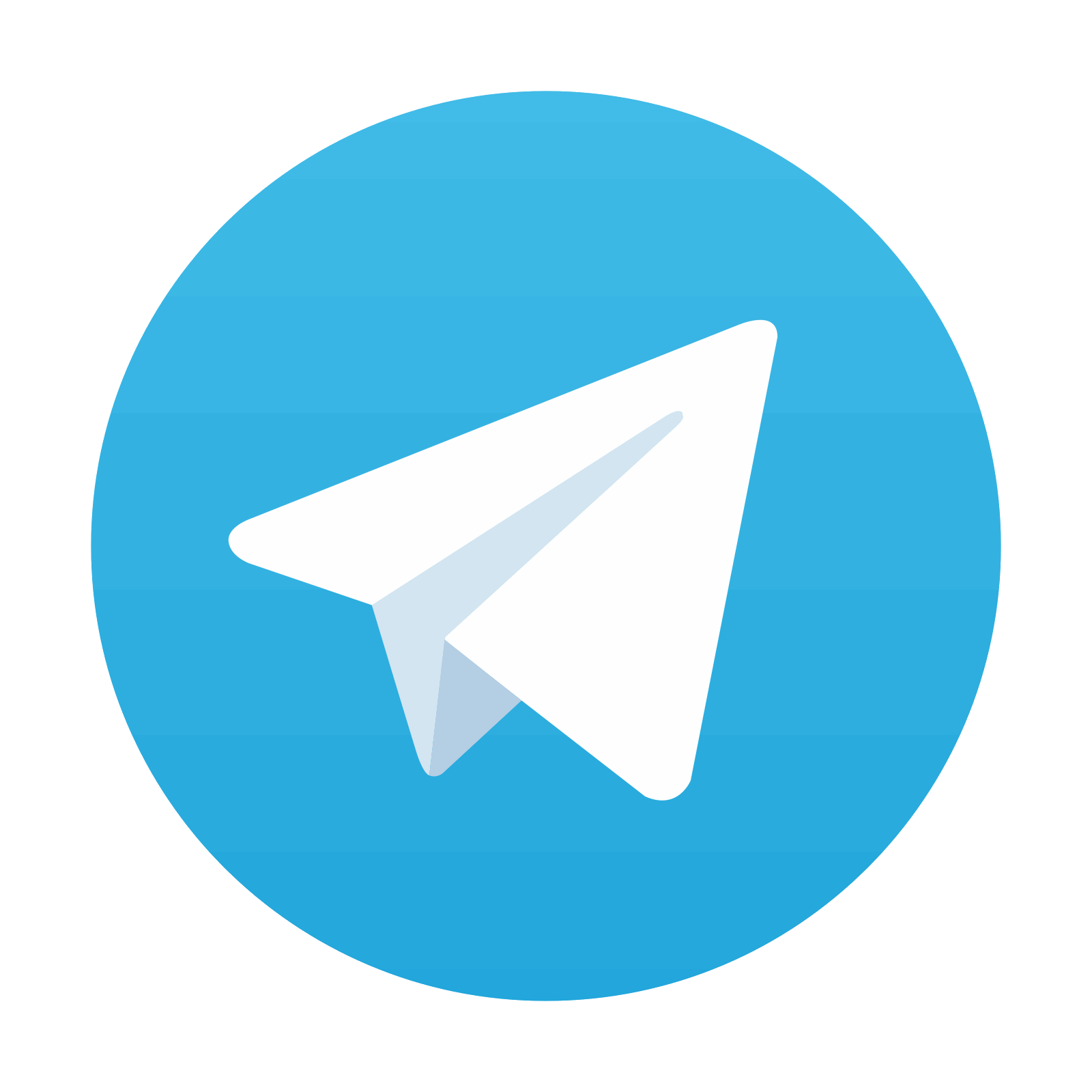
Stay updated, free articles. Join our Telegram channel

Full access? Get Clinical Tree
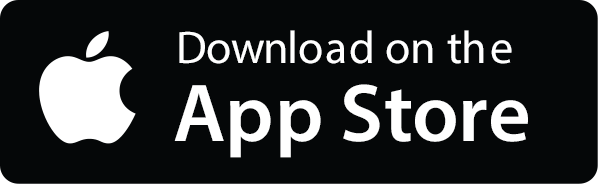
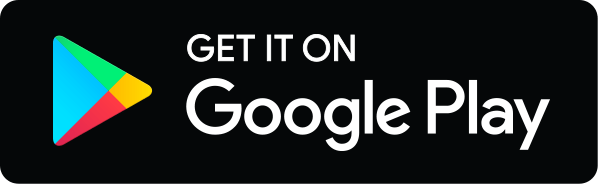