Key Features
- •
Combines lamellar surgery with the accuracy of the excimer laser.
- •
Hinged flap may be created either with a microkeratome or a femtosecond laser, however, femtosecond lasers produce thinner, more predictable flaps with fewer complications.
- •
Most widely used refractive surgery technique.
- •
Excellent option for refinement of refractive errors in pseudophakes especially with presbyopic and toric lenses.
- •
Recent advances including wave-front guidance, wavefront optimization, and topography guidance improve visual results and predictability.
- •
Recent rates of ectasia continue to fall due to better pre-operative screening and awareness.
Historical Review
Refractive corneal surgery principles date back at least to the nineteenth century. However, lamellar refractive surgery was not described until 1949, when Dr. José I. Barraquer realized that the refractive power of the eye could be altered by subtraction or addition of corneal tissue. The term keratomileusis, which is derived from the Greek roots keras (hornlike = cornea) and mileusis (carving), was used to describe the lamellar techniques. Unfortunately, there were many disadvantages to the procedure (i.e., complex instrumentation, steep learning curve, high rate of complications, and corneal scarring ), and the initial enthusiasm soon dissipated.
It was not until Dr. Luis Ruiz turned to keratomileusis in situ that the technical difficulties of the previous techniques were overcome. The introduction by Ruiz in the early 1980s of a microkeratome propelled by gears was a significant milestone for the development of modern refractive surgery. Dr. Leo Bores in 1987 performed the first keratomileusis in situ in the United States. This technique was still difficult to perform and had a significant complication rate.
Within a few years, advances in instrumentation allowed for the lamellar keratoplasty to be performed with greater accuracy of the resections; this became known as automated lamellar keratoplasty (ALK). Results of ALK for myopia showed improvement over previous lamellar techniques but still were far from predictable, with significant induction of irregular astigmatism and reduction of best-corrected visual acuity (BCVA).
The introduction of the excimer laser has had a greater impact on the practice of refractive surgery than any other event in the past 25 years. Trokel et al. suggested PRK after studying the effect of the excimer laser on animal corneas in 1983. It was later revealed that in myopia greater than 6.00 diopters (D), PRK resulted in significant central corneal haze, regression of the refractive effect, and poor predictability.
Buratto reported the use of the excimer laser in situ after a cap of corneal tissue was removed. In a further improvement of his technique, Pallikaris came up with the idea of combining the precision of PRK with the technique of ALK and creating a hinged flap that was replaced after treatment of the lamellar bed with the laser. In this way LASIK was introduced, designed, and developed at the University of Crete and the Vardinoyannion Eye Institute of Crete, Greece. LASIK avoided the inaccurate positioning of the corneal cap, which produced significant irregular astigmatism. Guimaraes et al. then reported that suturing could be eliminated by briefly drying the flap.
In summary, it was the combination of an old technique (keratomileusis) with new technology (excimer laser) that redefined corneal refractive surgery at the close of the twentieth century. Buratto and Pallikaris are credited with combining lamellar surgical techniques developed by Barraquer and excimer laser technology in a procedure they termed laser-assisted in situ keratomileusis.
LASIK
LASIK combines lamellar corneal surgery with the accuracy of the excimer laser. It involves the excimer laser ablation of the corneal stroma beneath a hinged corneal flap that is created with a microkeratome or a femtosecond laser.
LASIK has been used to correct up to 15.00 D of myopia, 6.00 D of hyperopia, and up to 6.00 D of astigmatism. The range of corrections utilized has not been clearly defined, and many surgeons have treated patients beyond these ranges with success. The results in the clinical evaluation of the procedure as far as predictability (percentage of eyes within a given postoperative target, i.e., ±0.5 D), efficacy (percentage of eyes with loss of best-corrected vision postoperatively, i.e., loss of two or more lines), stability (evaluation of stability of refraction at a certain interval postoperatively), and quality of vision (incidence of adverse visual phenomena, such as halos, glare, etc.) have been better in lower ranges than in higher ranges of correction. Most surgeons now limit their levels of correction to less than 8.00–10.00 D of myopia and 4.00 D of hyperopia.
The normal cornea has a prolate shape (greater curvature centrally than peripherally). Laser vision correction procedures for myopia reverse this natural prolate shape of the cornea and decrease the central corneal curvature to create an oblate shape ( Fig. 3.4.1 ). Corneal surgery for hyperopia differs in several important respects from surgery for myopia. The corneal refractive power must be increased to treat hyperopia, whereas it must be decreased to treat myopia. Excimer laser ablations for treatment of hyperopia are applied in the midperiphery and result in steepening of the central cornea and relative flattening of the periphery with minimal ablation occurring at the center of the cornea ( Fig. 3.4.2 ). Effective treatments for hyperopia have a larger ablation diameter than those for myopia.


Hyperopic astigmatism treatment is accomplished with the excimer laser by removing tissue along the paracentral area, promoting steepening of the flat meridian. Cylindrical ablation patterns for correction of mixed astigmatism include the bitoric and the cross-cylinder techniques. The bitoric LASIK technique consists of flattening the steep meridian with a cylindrical ablation in combination with a paracentral ablation over the flat meridian to steepen this axis. The cross-cylinder technique corrects astigmatism by dividing the cylinder power into two symmetrical parts. Half of the correction is treated on the negative meridian, and half is treated on the positive meridian.
Excimer Lasers
The excimer laser is used to reshape the surface of the cornea by removing anterior stromal tissue. The process by which the excimer laser removes corneal tissue is nonthermal ablative photodecomposition.
Laser delivery patterns include broad beam, scanning slit, and flying spot. Some lasers have a combination of mechanisms that allow for large and small treatment areas through a system termed variable spot scanning. This combines the advantage of a shorter treatment time by treating large areas all at once and the flexibility of treating smaller areas asymmetrically when needed with a small-diameter beam.
There are four basic types of excimer laser treatment profiles: conventional, wavefront-optimized, wavefront-guided, and topography-guided.
Conventional LASIK, also called standard or traditional LASIK, was the first profile to receive Food and Drug Administration (FDA) approval and still is used commonly today. Conventional LASIK applies a simple spherocylindrical correction obtained through a manifest refraction and based on the removal of tissue using Munnerlyn’s equation. However, conventional LASIK to treat myopia induces positive spherical aberration dependent on the amount of attempted correction.
In standard treatments, the best point to use for centration during the refractive procedure is still not clear, with the options of the corneal intercept of the visual axis, the entrance pupil, or the corneal light reflex. Eye-tracking devices rely on infrared lasers or cameras to follow small eye movements and move the laser ablation beam accordingly.
Studies have shown improvements in uncorrected visual acuity (UCVA), BCVA, and centration with eye-tracking devices. Larger ablations and blend zones may reduce the incidence of glare and halos.
Wavefront-guided (WFG) LASIK, also called custom LASIK, is a variation in which the excimer laser ablates a sophisticated pattern based on measurements from an aberrometer. The goal of WFG LASIK is to achieve an improved ablation based on the optical aberrations measured with the wavefront aberrometer, not just sphere and cylinder (lower-order aberrations). There are other types of optical aberrations in the visual pathway of the eye, such as coma and spherical aberration, collectively called higher-order aberrations (HOA). Wavefront technology measures both the lower-order aberrations and HOA, improving the precision of refraction to 0.1 D or smaller. There are several methods that can be used to measure the wavefront: Tscherning, dynamic skiascopy, ray tracing, and Hartmann–Shack. All methods evaluate how light is modified as it passes through the lens and cornea, and a wavefront is constructed by analyzing the exiting light rays. The shape of the wavefront describes the total aberration of the eye. The size of the wavefront (cross-sectional area) is determined by the size of the entrance pupil.
The first step in the wavefront-based LASIK technique is an examination with a wavefront device that measures the aberrations. The profile to correct these aberrations is created and imported into an excimer laser and used to guide the ablation during LASIK. ( Fig. 3.4.3A ). As the ablation patterns and treatments become more complex and more specific for the individual, the importance of precise registration of these patterns on the cornea increases. Significant cyclorotation could introduce significant postsurgical aberrations. Iris registration is able to compensate for pupil centroid shifts due to variable illuminations and pupil sizes by referencing to the outer iris boundary with improved centration of wavefront ablations.



The wavefront data can provide useful information in the postoperative setting, where it can be used to identify and describe specific HOA that may be consistent with a patient’s subjective visual symptoms.
Wavefront-optimized treatment profiles are population-based corrections designed to reduce or eliminate the induced spherical aberration of conventional LASIK. The wavefront-optimized treatment is based on a spherocylindrical correction that is adjusted by an internal algorithm to remove additional tissue in the periphery of the ablation zone, thereby creating a more prolate corneal shape.
In traditional myopic corrections, laser pulses at the peripheral cornea have a diminished effect due to the oblique angle of the laser beam, which induces spherical aberration. To compensate for this effect in a wavefront-optimized ablation, extra laser pulses are applied to the corneal periphery ( Fig. 3.4.3B ).
Topography-guided LASIK uses information from both the corneal shape and the refractive spherocylindrical correction to determine the excimer laser ablation profile ( Fig. 3.4.3C ).
Topographers, which are not limited by pupil size, can measure greater and wider points of curvature on the cornea compared with wavefront devices. Topography-guided treatments can be used successfully for highly aberrated eyes (such as corneas with opacities or irregular astigmatism) and are not affected by the state of accommodation, early cataracts, or vitreous opacities.
Patient Selection
Preoperative Evaluation and Diagnostic Approach
The first step in the evaluation should be to determine the goals of the patient in seeking refractive surgery and assess whether the patient has realistic expectations. Patients should understand the risks, benefits, and alternatives to the LASIK procedure. A stable refraction is important. Most surgeons now limit the upper range of correction to treatment of 8–10 D of myopia even though the lasers are capable of treating higher corrections.
Also important is a review of ocular and systemic conditions. Visual acuity is measured using manifest and cycloplegic refraction. The refraction is compared with prior spectacle corrections to assess the stability of refraction for the given eye. In addition, the wavefront refraction can be used as the baseline to obtain the wavefront-adjusted manifest refraction (WAMR). Pupil size, ocular dominance testing, and distance and near vision with and without correction should also be documented. Anterior and posterior segment examinations are performed to rule out other conditions that may adversely affect the surgical result.
Measurement of the central corneal thickness is an important element of the preoperative evaluation. WFG and optimized procedures usually remove more stromal tissue compared with conventional treatments. An estimated residual stromal bed is needed for surgical planning, because it is one factor that may predict postoperative ectasia. It is computed by subtracting the anticipated flap thickness and maximum ablation depth from the central corneal thickness measurement. The minimal residual bed for LASIK remains controversial, although 250–300 µm is generally recognized as a minimum.
Ectasia can occur with even thick residual stromal beds in eyes with keratoconus features. To account for variations in actual flap thickness, the stromal bed can be measured after the flap has been retracted to allow for a more precise determination of the residual bed. A percentage of anterior tissue depth altered (PTA) ≥40 at the time of LASIK was significantly associated with the development of ectasia in a study of eyes with normal preoperative topography.
Computer-assisted videokeratoscopy, corneal tomography, and Scheimpflug imaging are now available and used routinely in the preoperative and postoperative assessments of refractive surgery patients. These tools can help to screen for subclinical keratoconus or other corneal diseases.
Rigid contact lens wearers should remove their lenses for 3–4 weeks before examination, and soft contact lens wearers should have 2 weeks without lenses.
The possibility of monovision should be discussed with patients near or of presbyopic age; the discussion should include glare and halos, the possibility of under- and overcorrection, and any special considerations. Appropriate reading materials are helpful for patient education.
Informed consent should include a discussion of the most frequent side effects and potential risks involved with the surgery.
Alternative refractive treatments such as PRK should be discussed and may be preferred to LASIK in patients with anterior basement membrane dystrophy (ABMD), thin corneas, small and deep-set orbits, anterior scleral buckles, glaucoma after trabeculectomy, optic nerve disease, a risky occupation or activity, and corneal ectasia.
The option of phakic IOL implantation can be offered to patients with high myopic corrections, very thin corneas, or abnormal corneal topography and/or tomography. Natural lens replacement is an option for patients nearing cataract age or requiring higher hyperopic corrections.
Limitations and Contraindications
Laser vision correction may have a higher risk in patients with collagen vascular disease, patients with autoimmune or immunodeficiency diseases, women who are pregnant or nursing, patients with signs of keratoconus, and those taking isotretinoin or amiodarone. Other conditions potentially associated with more adverse outcomes include Fuchs’ corneal dystrophy, strabismus, ophthalmic herpes simplex or herpes zoster, and other systemic diseases likely to affect healing, such as diabetes and atopic disease. Caution should be exercised for patients with abnormal corneal topographies or with ocular abnormalities as well as systemic conditions that are likely to affect wound healing.
Microkeratomes and Femtosecond Lasers
A critical step in LASIK is the creation of the corneal flap. Several different microkeratomes are available for use in LASIK, ranging from modern automated steel microkeratomes to femtosecond lasers. The choice depends on the surgeon’s access and preference. In the past decade, the femtosecond laser has gained popularity and is replacing mechanical microkeratomes for LASIK flap creation in the United States. Femtosecond lasers use laser pulses to cause microcavitation bubbles at a preset depth in the corneal stroma. The cavitation bubble is composed primarily of water and carbon dioxide. Multiple cavitation bubbles coalesce, and an intrastromal cleavage plane is created. Hinge placement, flap diameters, and flap thickness can be set to exact specifications. The chance of a flap buttonhole or incomplete, decentered, or free flap appears to be reduced. Flaps are thinner and of uniform thickness from the center to the periphery. Femtosecond flap creation is very precise and flap thickness is usually within ±14 µm of the intended result. These differences in flap creation between femtosecond lasers and mechanical microkeratomes are thought to be responsible for better LASIK outcomes with the femtosecond laser.
Operative Technique
Careful candidate selection, as discussed earlier, is critical for optimal outcomes. Surgeon preparation, including a thorough knowledge of the patient, procedure, parameters, and equipment, is essential. Patient preparation, including preoperative explanation regarding the steps, sights, and sounds of the procedure, serves to maximize comfort and minimize anxiety. Approximately 5–10 minutes before the procedure, a mild sedative can be given to the patient to alleviate the anxiety of undergoing the procedure and to help them sleep postoperatively.
When performing a WFG LASIK procedure, obtaining an accurate capture of ocular aberrations is critical to ensure accurate measurements and optimal results ( Fig. 3.4.3A ).
Microkeratome Surgical Technique
Topical anesthesia is applied to the eye. The eyelids are prepared with dilute povidone–iodine solution. A lid speculum is inserted to open the eyelids. Eyelashes should be kept away from the surgical field by the use of adhesive drapes or a closed bladed lid speculum. The contralateral eye is taped shut to prevent cross-fixation and drying. The microkeratome should be inspected for any defects in the blade or function of the moving parts. It is vital to confirm that the excimer laser will be able to deliver treatment after the corneal flap is reflected.
The cornea can be marked with ink before creating the corneal flap with the microkeratome to more easily realign the corneal flap in the event that a free flap is created.
The suction ring is placed using a bimanual technique in which the shaft of the suction ring is held in the fingers of one hand and a finger from the other hand provides additional support on the ring itself. Once adequate placement has been achieved, the suction is engaged by foot control (usually done by the technician). Adequate intraocular pressure (above 65 mm Hg) is then verified, with one useful method being an applanation lens (Barraquer tonometer). When adequate suction is achieved, the patient will confirm the temporary loss of visualization of the fixation light.
Before the pass of the microkeratome, several drops of artificial tears are placed on the cornea. This lubrication reduces the likelihood of a corneal epithelial defect occurring during the microkeratome pass. If using a two-piece microkeratome, the head is slid onto the post of the suction ring and advanced until the gear on the microkeratome head engages the track. It is important to again verify that the suction ring is still firmly attached to the globe at this point by gently lifting the suction ring upward, making sure that the suction is not lost. The surgeon then activates the microkeratome using forward and reverse foot control, the suction is turned off after the microkeratome pass, and then the suction ring can be carefully removed. Prompt attention at this point is extremely important in the case that a free cap or buttonhole has been created. In cases in which the stromal bed is too small or irregular for a good result, the laser ablation should not be performed, and the flap should be placed carefully back into position.
Before the flap is lifted, a wet cellulose sponge is used to prevent any cells, debris, or excess fluid from getting onto the stromal bed. With use of a flat cannula, iris sweep, or smooth forceps, the flap can be lifted and directed toward the hinge. Assessment of the thickness of the residual corneal bed may be performed by using ultrasound pachymetry. Microkeratome flap thickness may vary, with differences in thickness occurring even with the same microkeratome, making this measurement more important in eyes that may require a deeper ablation with the excimer laser, thus leaving less tissue in the residual corneal bed.
Femtosecond Laser Flap Creation
The femtosecond laser is a highly precise, computer-guided laser that enables surgeons to program flap parameters, such as diameter, depth, hinge location, and edges, based on patients’ anatomical characteristics or surgeons’ preferences ( ). Flap parameters such as pattern, hinge position, flap depth, flap diameter, bed energy and spot separation, side cut angle and energy, hinge angles, and pocket parameters need to be carefully programmed or reviewed by the surgeon before the surgical procedure is initiated.
A suction ring is applied to the eye with slight downward pressure, and suction is then enabled to affix the ring firmly to the eye. Proper centration is extremely important and is a critical step that ensures greater accuracy of all subsequent steps of flap creation ( Fig. 3.4.4 ). With the eye fixated, the cornea is then fully applanated. Centration and flap size should be confirmed on the monitor before the laser is initialized for treatment. It is critical to instruct the patient to abstain from eyelid squeezing during this step to prevent suction loss ( Fig. 3.4.5A ).



Once the procedure is complete, suction can be released and the same technique can be repeated on the contralateral eye.
Lifting femtosecond flaps can be quite different from lifting a microkeratome flap and is rather a blunt dissection, best accomplished with the use of a spatula ( Fig. 3.4.5B ). The spatula should be entered near the hinge and gently elevated in one to four swipes. The flap is then reflected, and the surgeon can proceed with excimer laser treatment.
Excimer Laser Ablation
The patient should be positioned under the microscope with the head carefully aligned to make sure that the iris is perpendicular to the laser beam. Careful centration with the eye aligned in the x, y, and z planes is crucial. Alignment is even more critical with wavefront-guided laser treatments because most HOA are not radially symmetrical. Torsional misalignment—either cyclotorsion or head tilt—during surgery can result in undercorrection of the aberration or even in the induction of additional aberrations. The most basic technique to ensure alignment is to mark the limbus, typically at the 3 and 9 o’clock positions, immediately before surgery while the patient is seated. These marks are then used to align the head when the patient is lying under the laser. Most modern laser systems are now equipped with either pupil tracking or iris registration.
The surgeon should always verify the entered computer data before starting the ablation. The microscope should be adequately focused on the corneal surface. A dry cellulose sponge is then used to carefully remove any excess fluid from the stromal surface. Hydration of the stromal bed needs to be adjusted evenly and consistently in all cases. It is important at this point to minimize the procedure time in order to prevent stromal dehydration and subsequent overcorrection. Uneven hydration can lead to central islands and/or irregular astigmatism. Excess pooling of fluid often can be found near the hinge after folding back the flap and should be wicked away. The patient should be instructed to fixate on the target light. Adequate centration over the pupil should be reassessed ( Fig. 3.4.6 ).

The laser eye tracker and iris registration are activated, and the laser ablation initiated. If fluid starts accumulating over the stromal surface, the laser ablation can be halted, and the fluid should be removed with a cellulose sponge. The hinge should be protected if it is within the ablation zone.
After the ablation, the flap is then repositioned onto the bed using an irrigation cannula or an iris sweep. Saline solution is used to remove debris from the interface ( Fig. 3.4.7 ). A wet cellulose sponge is then used to realign the flap. Sweeping movements should be performed from the hinge toward the periphery of the flap.

Good adhesion of the flap is verified by stretching the flap toward the gutter. If good adhesion is present, there is minimal space in the gutter and no movement of the flap occurs when stroking the flap with a dry sponge. When the flap is felt to be securely in position, a drop of an antibiotic, a corticosteroid, and a lubricating agent may be applied to the cornea before removal of the speculum. If bilateral LASIK will be performed, the operated eye is covered and the procedure repeated in the contralateral eye. Both eyes are then protected with transparent plastic shields until the following day.
Postoperative Care
Postoperative care of the typical patient who has undergone LASIK is still quite important. Generally, some tearing and burning occurs immediately after surgery, for which it is recommended that the patient take a 2-hour nap. The patient is placed on topical prophylactic antibiotics and topical corticosteroids four times per day for 1–2 weeks. Preservative-free lubricating drops are helpful in most patients for the first several weeks after surgery, and frequent use should be encouraged.
On the first postoperative day, careful evaluation of the corneal flap should be performed at the slit lamp. The patient may resume most activities if the postoperative examination is normal. Instructions not to rub the eyes or swim underwater should be reinforced to prevent flap displacement or infectious keratitis.
Complications
Intraoperative Complications
An incomplete flap may result from the premature termination of the microkeratome advancement or ineffective passes or suction loss during a femtosecond flap creation. Reasons for a microkeratome incomplete pass include inadequate globe exposure due to interference of eyelid, lashes, speculum, and/or drape as well as loss of suction during the pass. If there is not enough room beneath the flap to perform the ablation, then the surgeon should reposition the flap and abort the surgery. Incomplete flaps also can result when the femtosecond laser cannot photodisrupt the corneal stroma in portions of the intended interface or if there is resistance within the interface from scar tissue. Typically, surface laser treatment with mitomycin-C can be used later to complete the refractive correction.
When suction loss occurs during a femtosecond LASIK flap creation, resulting in an incomplete flap, a second femtosecond pass can create an intact flap. Tomita and colleagues reported successful immediate lamellar recut in their series of eyes with suction loss. Some controversy surrounds the rationale for immediate recut, as other authors have demonstrated the potential for creating new cleavage planes if immediate recut is undertaken. When suction loss occurs during the side cut or just before starting the side cut, a repeat side cut can be performed with a smaller diameter.
A flap buttonhole is one of the most serious flap complications, and the excimer laser ablation should be aborted. The flap should be repositioned and smoothed into place. Treatment of the second eye is not advisable at the same setting, as the same complication is likely to happen in the presence of a steep cornea or poor suction. However, these almost always occur in the second eye with a thinner flap in mechanical microkeratomes. Femtosecond laser flaps also are prone to similar complications, albeit usually from a different mechanism: vertical gas breakthrough. Epithelial ingrowth or haze may occur in the area of the buttonhole and may require further intervention. Typically, retreatment can be performed immediately or later with phototherapeutic keratectomy (PTK)/PRK and mitomycin-C.
A free cap can occasionally occur when using a microkeratome to create a flap, and the surgeon should be prepared to deal with this problem. If the cap is small and/or decentered, it should be replaced without ablation and the procedure aborted. If it is well centered and of adequate size, the cap is typically placed on the conjunctiva with the epithelial side down during the photoablation. Care must be taken to reposition the cap into the same orientation after the ablation. Adequate drying time should be allowed for the cap to adhere without sutures. The most frequent cause of a free cap is a flat or small cornea in which there is less tissue to be brought forward into the microkeratome. Poor suction also can cause small free flaps. Marking of the cornea before flap creation can facilitate alignment in the event of a free cap.
Epithelial defects can be prevented with adequate lubrication of the cornea before the microkeratome pass. Also, toxic anesthetics should be kept to a minimum before the procedure. If a large epithelial defect occurs during the microkeratome pass, the ablation should be aborted in the affected eye. It is not recommended to proceed with flap creation in the contralateral eye, as the same complication is likely to occur. On the other hand, if the epithelial defect is small, a contact lens can be placed over the cornea to decrease significant discomfort to the patient. An epithelial defect may lead to greater flap edema with poorer adherence in the area of the defect, increasing the risk of epithelial ingrowth and diffuse lamellar keratitis.
Epithelial breakthrough is a rare complication during femtosecond laser corneal flap creation and is generally observed in patients with prior corneal incisions in the ablation zone. In these situations, increasing femtosecond energy or microkeratome flap creation should be considered. In patients with severe corneal scars that obscure visualization of anterior segment structures, femtosecond flap creation should be avoided. It is important to be attentive for this complication intraoperatively and to abstain from lifting the flap to prevent more serious flap problems such as buttonholes or flap tears.
An opaque bubble layer (OBL) and anterior chamber gas bubbles can present during corneal flap creation with a femtosecond laser. An OBL forms when gas bubbles created by laser photocavitation are trapped inside the stoma. Gas also can appear in the anterior chamber and persist up to several minutes or even hours. The OBL can make lifting of the flap difficult, and care must be taken to perform a gentle blunt dissection to prevent a flap tear in the area of the OBL. If the OBL or anterior chamber bubbles are over the pupillary area, they may interfere with an eye-tracking mechanism during excimer laser ablation. In these situations, it is usually recommended to wait for bubbles to dissipate before proceeding with laser treatment. Other factors associated with increased frequency of OBL include steeper, thicker corneas and a hard docking technique. Although the OBL makes the LASIK procedure more difficult, it does not appear to affect the postoperative optical quality and visual outcome ( Fig. 3.4.8 ).

Ablation Complications
Central islands are small central elevations in the corneal topography that may occur for a variety of reasons. Beam profile abnormalities, increased hydration of the central corneal stroma, or particulate material falling onto the cornea may block subsequent laser pulses. This was more common with broad-beamed lasers. Typically these central islands resolve with time as epithelial remodeling fills in the surrounding area.
Decentration ( Fig. 3.4.9 ) can result from poor fixation and alignment, eye movement during the laser procedure, significant pupil shift with light, or asymmetrical hydration of the cornea. The higher the myopic correction, the greater the risk of a decentered ablation, which can result in glare, irregular astigmatism, and a decrease in BCVA. Low-contrast visual acuity is a more sensitive measurement of visual function than high-contrast Snellen acuity and can be used to assess these patients more accurately. Decentration may be decreased with the use of current lasers with incorporated eye-tracking systems and iris registration, yet careful attention must still be paid to patient fixation. Typically, if the ablation is more than 1 mm decentered, the irregular astigmatism that occurs is symptomatic. Management of decentration by treatment based on wavefront or topographical information may decrease symptoms in patients with an unsatisfactory outcome with the first procedure.

Under- and overcorrection may result from errors of refraction, improper surgical ablation, malfunctioning of the excimer laser, abnormal corneal hydration status, or an excessive or inadequate wound-healing response. It is crucial to maintain consistent hydration of the cornea, because excessive fluid on the cornea results in an undercorrection. If desiccation of the corneal stroma is present, then overcorrection and haze may occur. The higher the refractive error, the greater the chance of regression. Many surgeons find that adjusting the amount of treatment using a nomogram based on their actual surgical results improves their refractive outcomes.
Postoperative Complications
Interface debris is common even with aggressive interface irrigation. The most frequent source of debris is meibomian gland material from the lids that is trapped in the interface. Careful draping of lashes and cleaning of the flap interface with balanced salt solution before and after the flap is floated into position can help to reduce the incidence of this problem.
Flap displacement usually occurs in the first 24 hours postoperatively. When a flap displacement occurs, it should be lifted and repositioned as soon as possible. The epithelium at the flap edge grows remarkably fast to cover the stromal bed. Care must be taken to clean the bed and back of the flap of debris and epithelial cells. Stroking the cap with a cellulose sponge can minimize persistent folds in the flap and properly line up the cap with the bed.
Punctate epithelial keratopathy (dry eye) is the most frequent complication of LASIK. Several possible mechanisms contributing to LASIK-induced dry eye have been proposed. The mechanisms include injury to the afferent sensory nerve fibers, a reduction in neurotrophic influences on epithelial cells, a decreased blinking rate, decreased tear production, altered tear-film stability and distribution, increased tear evaporation, and injury to limbal goblet cells. The preponderance of data supports the hypothesis that the most important factor in the pathophysiology of LASIK-induced dry eye is the transection of afferent sensory nerves in the anterior third of the stroma during the lamellar cut. The disorder tends to be more common and more severe in the context of underlying chronic dry eye. It has become evident, however, that the disorder is multifactorial. Studies in patients in whom the flap was created with a microkeratome showed significantly more signs and symptoms of LASIK-induced dry eye than those in which a femtosecond laser was used for flap creation. Treatment involves frequent lubrication of the ocular surface with artificial tears, topical anti-inflammatory therapy, and punctual plugs; management of any eyelid disorder also may be of benefit.
Diffuse lamellar keratitis (DLK), also known as Sands of Sahara syndrome, is an interface inflammatory process that occurs in the early postoperative period after LASIK ( Fig. 3.4.10 ). Patients are initially asymptomatic and often have no visual impairment. A fine granular-appearing infiltrate that looks like dust or sand typically presents initially in the interface periphery. If left untreated, the inflammation can progressively worsen and may lead to corneal scarring with resultant irregular astigmatism. The cause of DLK is likely multifactorial. Bacterial toxins or antigens, debris on the instruments, eyelid secretions, or other factors may play a role. Femtosecond LASIK flap creation has been associated with a higher risk for DLK than microkeratome flap creation. Studies suggest that newer generation femtosecond lasers with higher frequency are associated with decreased DLK rates because lower energy settings are used in creating LASIK flaps.

Treatment involves frequent topical corticosteroids, and if severe enough, interface irrigation. However, some authors have reported excellent results in the treatment of severe DLK with high-dose topical and oral corticosteroids without flap lifting and interface irrigation.
Flap striae and microstriae are common complications after LASIK. Most striae are asymptomatic and can be visualized if the flap is carefully examined with retroillumination. When microstriae occur over the pupil or when macrostriae exist, irregular astigmatism with visual aberrations and monocular diplopia may result. In such cases, the flap should be lifted again, hydrated, and stretched back into position.
Epithelial ingrowth into the interface between the flap and the stromal bed occurs in up to 3% of patients following myopic LASIK surgery ( Fig. 3.4.11 ). Known risk factors for this complication include epithelial defects at the time of surgery, history of recurrent corneal erosions, corneal basement membrane epithelial dystrophy, history of ingrowth in the other eye, hyperopic LASIK correction, flap striae or folds (as when the flap dislocates after surgery because of trauma or poor adhesion), flap instability, type 1 diabetes, and repeated LASIK surgeries. Rarely, the epithelial ingrowth progresses into the central visual axis, causing irregular astigmatism and loss of BCVA. In some cases, the epithelial cells will block nutritional support for the overlying stroma and lead to flap melt. If this is the case, the flap should be lifted, and careful scraping of the epithelium should be performed at the stromal bed as well as under the flap. In recurrent cases, suturing or flap gluing may help to reduce the incidence of recurrent epithelial ingrowth. Nd:YAG laser application has been described as a technique for the treatment of epithelial ingrowth after LASIK surgery.


Corneal haze can occur following LASIK. With fears of corneal ectasia following LASIK, surgeons continue to aim for thinner flaps. Although thin-flap LASIK has been reported to be successful, there have been reports of interface haze formation with thin-flap femtosecond LASIK (<90 µm), especially in young patients and could be related to focal disruptions of the Bowman’s layer and injury to the epithelial basement membrane.
Infectious keratitis after LASIK is a devastating, vision-threatening complication. Fortunately, the estimated incidence is low and reported to be between 1 in 1000 and 1 in 5000 procedures. Reported organisms include Mycobacterium spp., fungi, Nocardia spp., Staphylococcus aureus, Streptococcus viridans, coagulase-negative Staphylococcus spp., and Streptococcus pneumoniae. The most common organism cultured in a worldwide survey was methicillin-resistant Staphylococcus aureus (MRSA). Symptoms may include pain, photophobia, watering, decreased visual acuity, ghost images, and halos. Slit-lamp examination may reveal ciliary injection, epithelial defect, anterior chamber reaction, and hypopyon. In the case of mycobacteria and fungi, presentation is usually delayed several weeks after the LASIK procedure and then has a smoldering course. Clinically, the mycobacteria and fungi usually are seen in the interface, often with a feathery or indistinct margin. Gram-positive infections are usually seen shortly after the procedure, often at the flap margin, and usually have distinct, sharp margins.
It is important to maintain a high suspicion for atypical organisms. The flap should be lifted and cultures should be inoculated on blood, chocolate, Sabouraud and Lowenstein–Jensen agar, and blood–heart infusion. Smears should also be taken for Gram, Giemsa, and Calcofluor white stains as well as Ziehl–Neelsen for acid-fast bacteria.
The mycobacteria species associated with keratitis following LASIK belong to the nontuberculous mycobacteria group. These species are resistant to chemical disinfectants such as chlorine, which is probably why such infections may occur after surgical procedures. Clarithromycin and amikacin are the antibiotics of choice, but poor penetration of topical medications leads to persistent infection. Early flap lifting and soaking of the flap and bed with amikacin 0.08% and/or clarithromycin 1% followed by aggressive topical therapy leads to the best results.
Perhaps the most important factor within our control is prevention. Meibomian gland disease should be treated before LASIK. Instruments must be properly sterilized, and intraoperative sterile techniques should be employed, including the use of sterile gloves and drapes and disinfection of the skin and eyelids with povidone–iodine. During the procedure, instruments should be sterile and sterile plastic bags used for the nonsterile portions of the laser. Efforts should be made to avoid irrigating meibomian secretions into the interface. Suction lid specula may be helpful in removing excessive fluids and debris. Postoperatively, the patient should be instructed to wear shields and not to rub the eye. Prophylactic antibiotics should be used for 1 week postoperatively.
Keratectasia
Retrospective analysis of patients with ectasia suggests the following risk factors for progression of ectasia after laser vision correction: (1) abnormal preoperative topography, (2) low residual bed thickness, (3) young age, (4) low preoperative corneal thickness, and (5) high myopia. General agreement exists on leaving 250–300 µm of untouched posterior cornea stroma. Ablation below that limit may cause biomechanical weakening, causing the cornea to bulge forward. Another important cause of iatrogenic keratectasia is LASIK performed on unrecognized keratoconus suspects. Videokeratographic clues for a keratoconus suspect may include steep keratometry, inferior steepening of the cornea, asymmetry of the corneal curvature, or nonorthogonal astigmatism. Iatrogenic keratectasia has been reported as early as 1 week and as late as 2 years postoperatively.
Although ectasia is rare after laser vision correction, many management options are available. Most are similar to those available for naturally occurring keratoconus. Most patients can still function well with nonsurgical options, such as contact lenses. Intracorneal ring segments may be used to manage patients with ectasia after refractive surgery. Corneal collagen crosslinking (CXL) with riboflavin and ultraviolet-A (UVA) radiation is now being used to treat keratoconus and post-LASIK ectasia; the principal goal of the technique is to stabilize the progression of these corneal diseases. In previous studies of CXL outcomes, patients had improvement in corrected distance visual acuity (CDVA), uncorrected distance visual acuity (UDVA), maximum and average keratometry (K) values, several corneal topography indices, and corneal and optical HOA.
Although corneal transplantation (penetrating or lamellar) is not often required, transplantation does have a high rate of success in eyes with keratoconus and would likely yield similarly good results in eyes with ectasia after refractive surgery.
Results
The efficacy and predictability of laser refractive surgery have greatly improved in recent years, even with standard conventional techniques. Most of this improvement is due to the development of flying spot lasers, tracking systems, and 8- to 9-mm transition zones that allow for blending the steep step at the periphery of the ablation zone.
With traditional LASIK, the accuracy is greater for lower degrees of myopia. In one study of 130 eyes with an average preoperative spherical equivalent (SE) of −3.61 D followed for 12 months after LASIK, 98% obtained a correction within ±1 D from target and 93% obtained 20/40 or better UCVA.
Another study showed that in low myopia (−0.75 D to −6.00 D of myopia and 0–0.75 D of preoperative astigmatism), 50% of eyes achieved 20/25 or better and 90% of eyes achieved 20/40 or better at 1 month postoperatively, and the SE was within ±1.00 D of emmetropia in 89% of the patients. In high myopia (−6.00 to −20.00 D of myopia and 0 to 4.5 D of preoperative astigmatism), at 1 month, 35% of eyes were 20/25 or better and 71% of eyes were 20/40 or better, and the mean SE was within ±1.00 D of emmetropia in 63%. The results of this and other studies suggest more predictable results for low myopia without astigmatism than for high myopia correction or in eyes requiring astigmatic correction.
Data obtained from multicenter trials on wavefront-guided LASIK ablation for low to moderate myopia and astigmatism revealed that 98% of eyes achieved 20/20 UCVA, and 71% were at 20/16 or better uncorrected. What is even more impressive is the fact that postoperative UCVA was better than the preoperative best corrected results in 47% of patients.
Ongoing improvements in the custom wavefront treatments have resulted in more precise treatments and postoperative results even for higher corrections. The results achieved with VISX Star S4 laser (Advanced Medical Optics, Inc.; Santa Ana, CA) treatment of high myopia and astigmatism with Custom Vue customized ablations were remarkable. The mean preoperative refractive spherical error was −8.00 D (±1.4 D, range −5.5 to −11.3 D). The average cylinder was −1.00 D (±1.00, range 0.0 to −5.3 D). The manifest refraction spherical equivalent (MRSE) was −8.5 D (±1.3, range −6.4 to −11.8 D). At 6 months, 98% of the eyes were seeing 20/40 or better uncorrected, 84% were 20/20 or better, and 65% were 20/16 or better. Three quarters had the same or better postoperative UCVA compared with their preoperative best spectacle-corrected visual acuity (BSCVA). Among the spherical myopes, 99% were at 20/20 or better uncorrected, with 84% at 20/16 or better at 6 months. Patients’ satisfaction with their quality of vision was also high. This demonstrated that the customized approach offers excellent quantity as well as quality of vision even for higher corrections.
Low to moderate levels of spherical hyperopia, simple hyperopic astigmatism, and compound hyperopic astigmatism can be effectively and safely corrected with LASIK. The results of a study evaluating patients with primary and secondary hyperopia who underwent traditional LASIK demonstrated that patients with primary hyperopia and a mean manifest SE of +1.73±0.79 D before surgery obtained a postoperative SE of −0.13±1.00 D at 6 months after surgery and an SE of −0.18±1.08 D at 1 year after surgery. At 6 months, 84% of patients with secondary hyperopia had a UCVA of 20/40 or better; 76% were within ±1 D of emmetropia. At 1 year, 85% had a UCVA of 20/40 or better and 85% were within ±1 D of emmetropia. No patients with secondary hyperopia lost two or more lines of BCVA at 1 year. For higher levels of correction, the predictability within ±1 D of attempted correction decreases to approximately 50%–80%, and the loss of BCVA generally ranges from 0% to 7%. However, LASIK for hyperopia greater than +5.00 D is not recommended, as it may result in a loss of BSCVA in a significant number of eyes (13%–15%).
Multicenter clinical trials of wavefront-guided LASIK for the correction of hyperopia and hyperopic astigmatism demonstrated significant improvements compared with traditional LASIK. Mean preoperative spherical error was +1.67±1.00 (up to +4.59 D), the average astigmatism was +0.65±0.48 D (up to +2), and MRSE was +1.99±1.00 D (up to 4.84 D). At 6 months, 95% of the eyes had a UCVA of 20/40 or better, 62% were at 20/20 or better, and 20% were at 20/16 or better. The UCVA at 9 months was 20/16 or better in 24% of eyes and 20/20 or better in 72% of eyes.
For mixed astigmatism, multicenter trials for custom wavefront LASIK results 6 months after surgery were a UCVA of 20/40 or better in 96% of eyes and 20/20 or better in 60% of eyes.
Wavefront-guided and wavefront-optimized procedures attempt to minimize induced aberrations in different ways. Aspherical or wavefront-optimized ablation profiles have been developed to avoid inducing spherical aberrations. Stonecipher and Kezirian reported 3-month results of an FDA trial for LASIK with the Allegretto Wave comparing wavefront-optimized versus wavefront-guided LASIK for myopic astigmatism, finding no statistically significant differences between either treatment group in regard to visual acuity and refractive outcomes. They stated that 93% of patients in each group had achieved 20/20 vision or better. These results are comparable to those reported in a prospective, randomized study by Miraftaf et al., who compared wavefront-guided and wavefront-optimized LASIK in contralateral eyes with myopia up to 7.00 D and astigmatism up to 3.00 D, also reporting no statistical differences in the UCVA, BCVA, or contrast sensitivity between both groups with 20/20 vision or better in 83.8% of the wavefront-optimized eyes and 89.2% of the wavefront-guided eyes.
Stonecipher and Kezirian concluded in their study that wavefront-guided treatments may be considered if the magnitude of preoperative root mean square (RMS) HOA is >0.35 µm, and in their study population, 83% of eyes had preoperative RMS HOA of <0.3 µm.
Studies using topography-guided LASIK have been encouraging. A prospective study of topography-guided LASIK using the WaveLight Allegretto Wave Eye-Q Laser included 249 eyes of patients with up to –9.00 D of SE myopia at the spectacle plane with up to 6.00 D of astigmatism. Topography-guided treatment resulted in a significant reduction in MRSE and cylinder, reaching stability at 3 months after treatment. The mean MRSE was 0.06±0.33 D at 3 months and 0.00±0.27.00 D at 1 year. At 1 year, 94.8% of eyes were within 0.50 D of plano. At 1 year, 15.7% of eyes saw 20/10 or better without correction; 34.4% of eyes saw 20/12.5 or better; 64.8% of eyes saw 20/16 or better; 92.6% of eyes saw 20/20 or better; and 96.5% of eyes saw 20/25 or better. Eyes treated with topography-guided treatment achieved an improvement in UCVA compared with preoperative BSCVA, with 29.6% of eyes gaining one or more lines of UCVA and 89.9% of eyes seeing at least as well without correction postoperatively as they did with best spectacle correction preoperatively.
In a comparative study, both topography-guided and wavefront-optimized LASIK for myopia in virgin eyes provided excellent results. However, topography-guided LASIK was associated with better contrast sensitivity, lower induction of HOA, and a smaller amount of tissue ablation.
With regard to patient satisfaction following LASIK, a comprehensive literature review was conducted in 2008 by the Joint LASIK Study Task Force. The results showed that 95% of patients were satisfied with their visual outcome after myopic and hyperopic LASIK.
Modern LASIK outcomes support the safety, efficacy, and patient satisfaction of the procedure and appear better than those reported in summaries of the safety and effectiveness of earlier laser refractive surgery systems approved by the FDA. In a recent literature review of LASIK articles published between 2008 and 2015, the aggregate loss of two or more lines of corrected distance visual acuity was 0.61% (359/58 653). The overall percentage of eyes with a UCVA better than 20/40 was 99.5% (59 503/59 825). The SE refraction was within ±1.00 D of the target refraction in 98.6% of eyes (59 476/60 329), with 90.9% (59 954/65 974) within ± 0.50 D. In studies reporting patient satisfaction, 1.2% (129/9726) of patients were dissatisfied with LASIK.
In addition, Price et al. compared visual satisfaction between LASIK and contact lens wear over a 3-year period. Current LASIK technology improved ease of night driving, did not significantly increase dry eye symptoms, and resulted in higher levels of satisfaction at 1, 2, and 3 years of follow-up.
Excimer Lasers
The excimer laser is used to reshape the surface of the cornea by removing anterior stromal tissue. The process by which the excimer laser removes corneal tissue is nonthermal ablative photodecomposition.
Laser delivery patterns include broad beam, scanning slit, and flying spot. Some lasers have a combination of mechanisms that allow for large and small treatment areas through a system termed variable spot scanning. This combines the advantage of a shorter treatment time by treating large areas all at once and the flexibility of treating smaller areas asymmetrically when needed with a small-diameter beam.
There are four basic types of excimer laser treatment profiles: conventional, wavefront-optimized, wavefront-guided, and topography-guided.
Conventional LASIK, also called standard or traditional LASIK, was the first profile to receive Food and Drug Administration (FDA) approval and still is used commonly today. Conventional LASIK applies a simple spherocylindrical correction obtained through a manifest refraction and based on the removal of tissue using Munnerlyn’s equation. However, conventional LASIK to treat myopia induces positive spherical aberration dependent on the amount of attempted correction.
In standard treatments, the best point to use for centration during the refractive procedure is still not clear, with the options of the corneal intercept of the visual axis, the entrance pupil, or the corneal light reflex. Eye-tracking devices rely on infrared lasers or cameras to follow small eye movements and move the laser ablation beam accordingly.
Studies have shown improvements in uncorrected visual acuity (UCVA), BCVA, and centration with eye-tracking devices. Larger ablations and blend zones may reduce the incidence of glare and halos.
Wavefront-guided (WFG) LASIK, also called custom LASIK, is a variation in which the excimer laser ablates a sophisticated pattern based on measurements from an aberrometer. The goal of WFG LASIK is to achieve an improved ablation based on the optical aberrations measured with the wavefront aberrometer, not just sphere and cylinder (lower-order aberrations). There are other types of optical aberrations in the visual pathway of the eye, such as coma and spherical aberration, collectively called higher-order aberrations (HOA). Wavefront technology measures both the lower-order aberrations and HOA, improving the precision of refraction to 0.1 D or smaller. There are several methods that can be used to measure the wavefront: Tscherning, dynamic skiascopy, ray tracing, and Hartmann–Shack. All methods evaluate how light is modified as it passes through the lens and cornea, and a wavefront is constructed by analyzing the exiting light rays. The shape of the wavefront describes the total aberration of the eye. The size of the wavefront (cross-sectional area) is determined by the size of the entrance pupil.
The first step in the wavefront-based LASIK technique is an examination with a wavefront device that measures the aberrations. The profile to correct these aberrations is created and imported into an excimer laser and used to guide the ablation during LASIK. ( Fig. 3.4.3A ). As the ablation patterns and treatments become more complex and more specific for the individual, the importance of precise registration of these patterns on the cornea increases. Significant cyclorotation could introduce significant postsurgical aberrations. Iris registration is able to compensate for pupil centroid shifts due to variable illuminations and pupil sizes by referencing to the outer iris boundary with improved centration of wavefront ablations.



The wavefront data can provide useful information in the postoperative setting, where it can be used to identify and describe specific HOA that may be consistent with a patient’s subjective visual symptoms.
Wavefront-optimized treatment profiles are population-based corrections designed to reduce or eliminate the induced spherical aberration of conventional LASIK. The wavefront-optimized treatment is based on a spherocylindrical correction that is adjusted by an internal algorithm to remove additional tissue in the periphery of the ablation zone, thereby creating a more prolate corneal shape.
In traditional myopic corrections, laser pulses at the peripheral cornea have a diminished effect due to the oblique angle of the laser beam, which induces spherical aberration. To compensate for this effect in a wavefront-optimized ablation, extra laser pulses are applied to the corneal periphery ( Fig. 3.4.3B ).
Topography-guided LASIK uses information from both the corneal shape and the refractive spherocylindrical correction to determine the excimer laser ablation profile ( Fig. 3.4.3C ).
Topographers, which are not limited by pupil size, can measure greater and wider points of curvature on the cornea compared with wavefront devices. Topography-guided treatments can be used successfully for highly aberrated eyes (such as corneas with opacities or irregular astigmatism) and are not affected by the state of accommodation, early cataracts, or vitreous opacities.
Patient Selection
Preoperative Evaluation and Diagnostic Approach
The first step in the evaluation should be to determine the goals of the patient in seeking refractive surgery and assess whether the patient has realistic expectations. Patients should understand the risks, benefits, and alternatives to the LASIK procedure. A stable refraction is important. Most surgeons now limit the upper range of correction to treatment of 8–10 D of myopia even though the lasers are capable of treating higher corrections.
Also important is a review of ocular and systemic conditions. Visual acuity is measured using manifest and cycloplegic refraction. The refraction is compared with prior spectacle corrections to assess the stability of refraction for the given eye. In addition, the wavefront refraction can be used as the baseline to obtain the wavefront-adjusted manifest refraction (WAMR). Pupil size, ocular dominance testing, and distance and near vision with and without correction should also be documented. Anterior and posterior segment examinations are performed to rule out other conditions that may adversely affect the surgical result.
Measurement of the central corneal thickness is an important element of the preoperative evaluation. WFG and optimized procedures usually remove more stromal tissue compared with conventional treatments. An estimated residual stromal bed is needed for surgical planning, because it is one factor that may predict postoperative ectasia. It is computed by subtracting the anticipated flap thickness and maximum ablation depth from the central corneal thickness measurement. The minimal residual bed for LASIK remains controversial, although 250–300 µm is generally recognized as a minimum.
Ectasia can occur with even thick residual stromal beds in eyes with keratoconus features. To account for variations in actual flap thickness, the stromal bed can be measured after the flap has been retracted to allow for a more precise determination of the residual bed. A percentage of anterior tissue depth altered (PTA) ≥40 at the time of LASIK was significantly associated with the development of ectasia in a study of eyes with normal preoperative topography.
Computer-assisted videokeratoscopy, corneal tomography, and Scheimpflug imaging are now available and used routinely in the preoperative and postoperative assessments of refractive surgery patients. These tools can help to screen for subclinical keratoconus or other corneal diseases.
Rigid contact lens wearers should remove their lenses for 3–4 weeks before examination, and soft contact lens wearers should have 2 weeks without lenses.
The possibility of monovision should be discussed with patients near or of presbyopic age; the discussion should include glare and halos, the possibility of under- and overcorrection, and any special considerations. Appropriate reading materials are helpful for patient education.
Informed consent should include a discussion of the most frequent side effects and potential risks involved with the surgery.
Alternative refractive treatments such as PRK should be discussed and may be preferred to LASIK in patients with anterior basement membrane dystrophy (ABMD), thin corneas, small and deep-set orbits, anterior scleral buckles, glaucoma after trabeculectomy, optic nerve disease, a risky occupation or activity, and corneal ectasia.
The option of phakic IOL implantation can be offered to patients with high myopic corrections, very thin corneas, or abnormal corneal topography and/or tomography. Natural lens replacement is an option for patients nearing cataract age or requiring higher hyperopic corrections.
Limitations and Contraindications
Laser vision correction may have a higher risk in patients with collagen vascular disease, patients with autoimmune or immunodeficiency diseases, women who are pregnant or nursing, patients with signs of keratoconus, and those taking isotretinoin or amiodarone. Other conditions potentially associated with more adverse outcomes include Fuchs’ corneal dystrophy, strabismus, ophthalmic herpes simplex or herpes zoster, and other systemic diseases likely to affect healing, such as diabetes and atopic disease. Caution should be exercised for patients with abnormal corneal topographies or with ocular abnormalities as well as systemic conditions that are likely to affect wound healing.
Preoperative Evaluation and Diagnostic Approach
The first step in the evaluation should be to determine the goals of the patient in seeking refractive surgery and assess whether the patient has realistic expectations. Patients should understand the risks, benefits, and alternatives to the LASIK procedure. A stable refraction is important. Most surgeons now limit the upper range of correction to treatment of 8–10 D of myopia even though the lasers are capable of treating higher corrections.
Also important is a review of ocular and systemic conditions. Visual acuity is measured using manifest and cycloplegic refraction. The refraction is compared with prior spectacle corrections to assess the stability of refraction for the given eye. In addition, the wavefront refraction can be used as the baseline to obtain the wavefront-adjusted manifest refraction (WAMR). Pupil size, ocular dominance testing, and distance and near vision with and without correction should also be documented. Anterior and posterior segment examinations are performed to rule out other conditions that may adversely affect the surgical result.
Measurement of the central corneal thickness is an important element of the preoperative evaluation. WFG and optimized procedures usually remove more stromal tissue compared with conventional treatments. An estimated residual stromal bed is needed for surgical planning, because it is one factor that may predict postoperative ectasia. It is computed by subtracting the anticipated flap thickness and maximum ablation depth from the central corneal thickness measurement. The minimal residual bed for LASIK remains controversial, although 250–300 µm is generally recognized as a minimum.
Ectasia can occur with even thick residual stromal beds in eyes with keratoconus features. To account for variations in actual flap thickness, the stromal bed can be measured after the flap has been retracted to allow for a more precise determination of the residual bed. A percentage of anterior tissue depth altered (PTA) ≥40 at the time of LASIK was significantly associated with the development of ectasia in a study of eyes with normal preoperative topography.
Computer-assisted videokeratoscopy, corneal tomography, and Scheimpflug imaging are now available and used routinely in the preoperative and postoperative assessments of refractive surgery patients. These tools can help to screen for subclinical keratoconus or other corneal diseases.
Rigid contact lens wearers should remove their lenses for 3–4 weeks before examination, and soft contact lens wearers should have 2 weeks without lenses.
The possibility of monovision should be discussed with patients near or of presbyopic age; the discussion should include glare and halos, the possibility of under- and overcorrection, and any special considerations. Appropriate reading materials are helpful for patient education.
Informed consent should include a discussion of the most frequent side effects and potential risks involved with the surgery.
Alternative refractive treatments such as PRK should be discussed and may be preferred to LASIK in patients with anterior basement membrane dystrophy (ABMD), thin corneas, small and deep-set orbits, anterior scleral buckles, glaucoma after trabeculectomy, optic nerve disease, a risky occupation or activity, and corneal ectasia.
The option of phakic IOL implantation can be offered to patients with high myopic corrections, very thin corneas, or abnormal corneal topography and/or tomography. Natural lens replacement is an option for patients nearing cataract age or requiring higher hyperopic corrections.
Limitations and Contraindications
Laser vision correction may have a higher risk in patients with collagen vascular disease, patients with autoimmune or immunodeficiency diseases, women who are pregnant or nursing, patients with signs of keratoconus, and those taking isotretinoin or amiodarone. Other conditions potentially associated with more adverse outcomes include Fuchs’ corneal dystrophy, strabismus, ophthalmic herpes simplex or herpes zoster, and other systemic diseases likely to affect healing, such as diabetes and atopic disease. Caution should be exercised for patients with abnormal corneal topographies or with ocular abnormalities as well as systemic conditions that are likely to affect wound healing.
Microkeratomes and Femtosecond Lasers
A critical step in LASIK is the creation of the corneal flap. Several different microkeratomes are available for use in LASIK, ranging from modern automated steel microkeratomes to femtosecond lasers. The choice depends on the surgeon’s access and preference. In the past decade, the femtosecond laser has gained popularity and is replacing mechanical microkeratomes for LASIK flap creation in the United States. Femtosecond lasers use laser pulses to cause microcavitation bubbles at a preset depth in the corneal stroma. The cavitation bubble is composed primarily of water and carbon dioxide. Multiple cavitation bubbles coalesce, and an intrastromal cleavage plane is created. Hinge placement, flap diameters, and flap thickness can be set to exact specifications. The chance of a flap buttonhole or incomplete, decentered, or free flap appears to be reduced. Flaps are thinner and of uniform thickness from the center to the periphery. Femtosecond flap creation is very precise and flap thickness is usually within ±14 µm of the intended result. These differences in flap creation between femtosecond lasers and mechanical microkeratomes are thought to be responsible for better LASIK outcomes with the femtosecond laser.
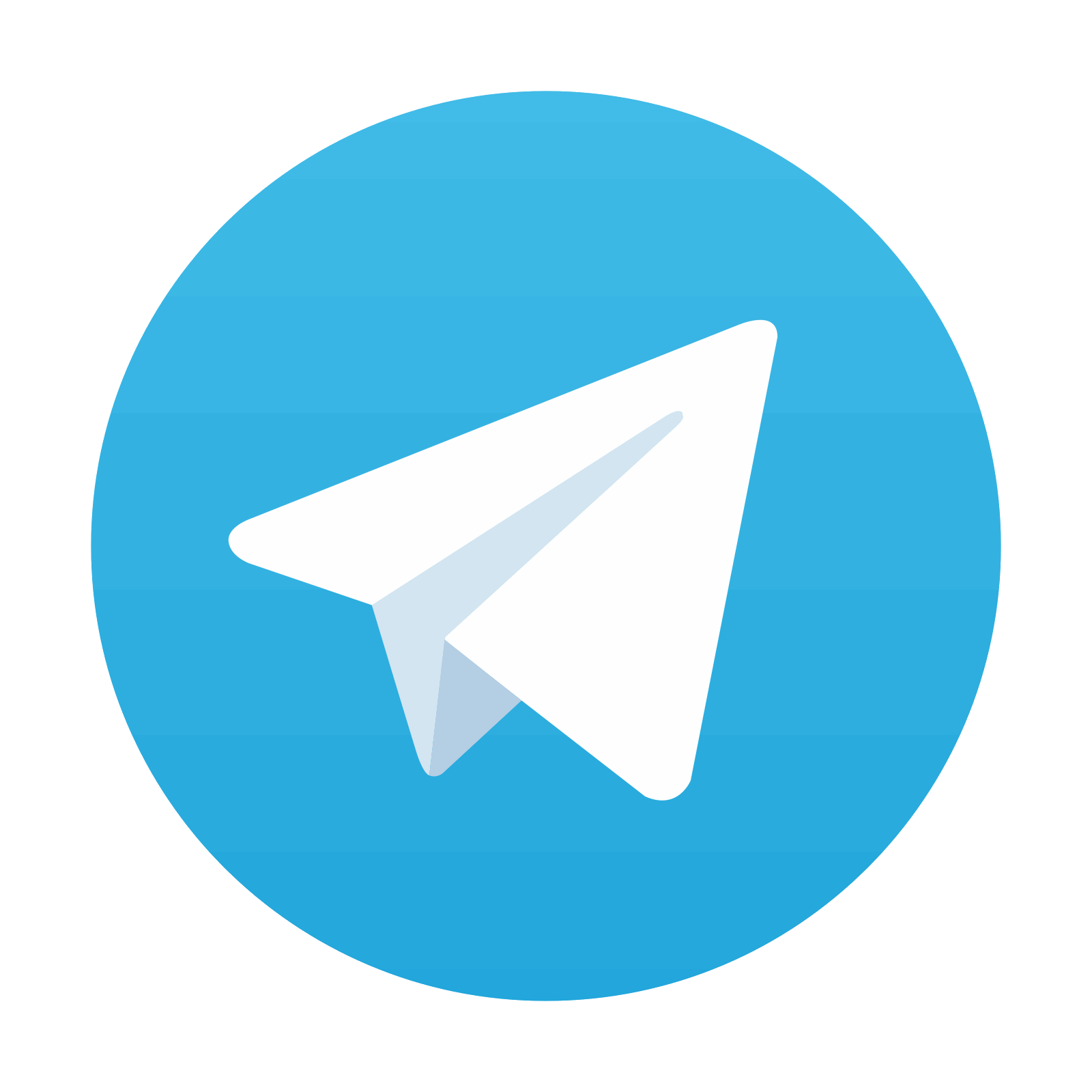
Stay updated, free articles. Join our Telegram channel

Full access? Get Clinical Tree
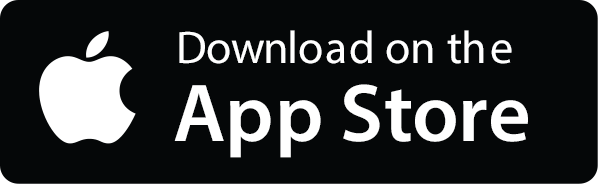
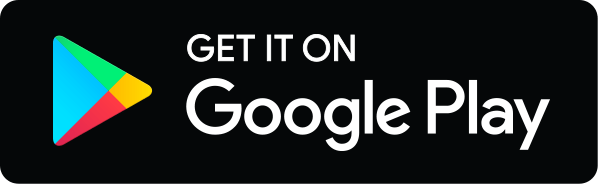