Included on DVD
INTRODUCTION
The laser is a technical miracle. It was Albert Einstein who predicted the existence of laser light but it took almost another half-century until the first laser was developed. Initially, they were known as masers (microwave amplification by stimulated emission of radiation), but they were soon renamed lasers (light amplification by stimulated emission of radiation).
Laser light is unique due to its three characteristic properties. It is:
- ⧫
monochromatic, which means it emits light of one specific wavelength
- ⧫
coherent, which means that all light waves are in the same phase
- ⧫
collimated, meaning that the light rays are all parallel.
A typical laser device consists of an optical medium, a source of excitation producing light or energy, and a set of mirrors positioned to potentiate the excitation light.
The use of lasers in selective photothermolysis was heralded in a 1980 paper by Rox Anderson, and since then lasers have found a wide range of medical applications.
‘[Selective photothermolysis] relies on selective absorption of a brief radiation pulse to generate and confine heat at certain pigmented targets. An absolute requirement is that the targets have greater optical absorption at some wavelength than surrounding tissues…’ (Anderson & Parrish)
CLASSIFICATION OF LASER DEVICES ( Tables 5.1–5.4 )
Basic Concepts
Laser light is widely thought to penetrate the skin, where it is specifically absorbed by the target. However, this is only partially true. Firstly, the light is reflected, not only by the skin surface but also by optical grenz zones; secondly, it is transmitted through the more-or-less transparent skin; thirdly, it is scattered whenever it hits particles; fourthly, it is absorbed and its relative energy transformed into heat ( Fig. 5.1 ). The tissue effect is caused by the absorption. The deeper the target, the more energy will be non-specifically lost by reflection and scattering, until a threshold is reached at which no tissue effects will be generated.
Lasing medium | Laser type |
---|---|
Gas | CO 2 , argon |
Liquid | Dye lasers |
Solid | Crystal, ruby, alexandrite, NdYag-diode |
Laser type | Wavelength | Color | Indications |
---|---|---|---|
Xenon chloride excimer | 308 nm CW | UV-B | Vitiligo, psoriasis |
Argon |
|
|
|
KTP (frequency doubled Nd-YAG) | 532 nm QS | Yellow | Telangiectasiae |
Copper vapor | 511 nm, 578 nm pseudo-continuous | Yellow, orange | Pigment, blood vessels |
Tunable dye (argon laser pumped) | 550–585 nm CW | Yellow, orange, red | Blood vessels |
Pulsed dye laser (flash-lamp pumped) | 585 nm pulse | Orange | Blood vessels |
Q-switched ruby | 694 nm QS | Red | Pigments |
Alexandrite | 755 nm QS, long-pulse | Near infrared | Pigments, tattoos |
Diode |
|
Near infrared | Hair removal |
Nd-YAG | 1060 nm CW, pulse, long-pulse, QS | Infrared | Pigments, hair removal |
Nd-YAG | 1360 nm | Infrared | Pigment, tattoos, coagulation, hair removal, etc. |
Diode | 1450 nm | Infrared | Photorejuvenation |
Er-glass | 1550 nm | Infrared | Photorejuvenation |
Er-YAG | 2940 nm pulse | Infrared | Laserablation, microablation, fractional |
Carbon dioxide | 10 600 nm CW, pulsed | Infrared | Resurfacing, cutting, vaporizing, fractional |
Intense pulsed light |
|
Broad range visible light | Photorejuvenation, pigment lesions, telangiectasiae |
|
|

A laser for medical use is characterized by its wavelength (in other words its color), its mode of light emission as a continuous wave (CW) or pulsed, by the pulse width (duration of a single light pulse), by its power and by its fluence.
Wavelength
The wavelength is the color of the light used. It determines the absorption of the light energy by the pigmented target (chromophore). Many of the intended targets for treatment are beneath the skin surface and the wavelength also plays a role in determining the amount of scatter and hence the penetration depth of the light energy; penetration of visible laser light is inversely related to wavelength.
Pulse Width
Pulse width is the time interval over which the energy is delivered. For effective treatment, this pulse width should be shorter than the thermal relaxation time (TRT) of the intended target. The TRT is a measure of the time it takes for 50% of the energy absorbed by the target (i.e. heat) to diffuse into the surrounding tissue.
Fluence
Fluence is the amount of light energy per area delivered to heat a target. Sufficient energy must be provided to damage the tissues of the target. For a given laser energy a smaller spot size creates a higher fluence. To calculate the fluence the equation below is used:
Spot Size
Spot size is the diameter of the beam at the skin surface. This parameter affects the treatment area and depth.
Repetition Rate
Repetition rate is the number of pulses per second and determines the speed of the treatment.
Energy
Energy is measured in joules and is defined as force × distance (N × m). Power is energy divided by time (J/s). Irradiance is power density, i.e. power divided by area (W/cm 2 ), and fluence is power × time divided by area (J/cm 2 ). These variables are of the utmost importance as they allow the laser parameters to be accurately determined and measured, and understanding of them makes the difference between a physician and a beautician. Lasers can be classified by power as low-level, medium-level or strong lasers.
While the specific wavelength of a laser defines its absorption spectrum and thus its principal biophysical effects, the duration of the laser emission also has a profound impact on the end result. CW lasers can be used to coagulate, cut, carbonize or vaporize tissue, or to stimulate certain cells. They usually exert important thermal effects on the tissues that absorb the energy, but there is also an effect on neighboring tissue, due to heat convection. The argon laser is a typical example of a CW laser that is used to coagulate blood vessels (port-wine stains, sun-burst vessels) and to heat up tissue (xanthelasma, small fibromas, small seborrheic keratoses).
Different techniques have been used to reduce the undesirable effect of CW lasers on adjacent tissue. The superpulse and ultrapulse techniques use surgical carbon dioxide lasers with multiple interruptions, which give time for the tissue to cool down between pulses. Another technique is to use scanners, which reduce the possibility of inaccurate application of the laser beam by the physician.
Pulsed lasers emit short bursts of high energy light. This extreme power can lead to a number of undesirable side effects: when treating port-wine stains or telangiectasiae with a flash-lamp pumped dye laser, the power of a single pulse can be so high that the target tissues – the blood vessels and erythrocytes – explode and cause purpura, a reddish-black discoloration, which is cosmetically embarrassing. On the other hand, short pulses are shorter than the so-called thermal relaxation time, defined as half the time needed for 50% of the heat to pass to neighboring structures. When pulses remain below the thermal relaxation time of a given target no heat damage of the adjacent structures occurs.
To make a continuous wave laser into a pulsed one, a quality switch (Q-switch; QS) is necessary. This changes many of the laser properties, examples being the CW neodymium-YAG and the QS Nd-YAG lasers. Another way to modify a laser is frequency doubling, which changes the biophysical properties such as light absorption.
TYPES OF LASER
Continuous Wave Lasers for Aesthetic Enhancement
CW lasers are now rarely used in aesthetic dermatology. The three main CW lasers still used are argon, tunable dye and CO 2 lasers, now seen by many as ‘historical’. Three parameters can be set by the operator: power, spot size and speed of movement (or length of shutter).
The CO 2 laser was the most important surgical laser used for cutting, vaporizing, carbonizing and, above all, for skin resurfacing. The latter procedure gives excellent results that cannot be achieved with modern non-invasive procedures. However, its main disadvantage is the long downtime period and the comparatively high risk of adverse side effects. Because its use is invasive, it will not be dealt with here. The Er-YAG laser, the light of which is absorbed 10 times more specifically by water, leaves much less thermal damage and healing is therefore faster. It may be an alternative for the treatment of prominent scars, mesh graft-like scars, excess graft tissue, some burn scars and traumatic tattoos.
The argon laser, with its strong blue and weaker green emission, was the first vascular laser. Its emission wavelengths do not coincide with any of the oxyhemoglobin absorption maxima, and heat conduction from the target to neighboring structures is an additional source of tissue damage. Furthermore, before even reaching the vessels, melanin of the epidermis absorbs much of its light energy. Depigmentation and epidermal necrosis are the consequences, often accompanied by scarring. Today’s indications are mainly cherry angiomas, syringomas and xanthelasmata and other soft tissue lesions ( Fig. 5.2 and 5.3 ).


The tunable dye laser, pumped by an argon laser, could be adjusted to the 577 nm oxyhemoglobin absorption maximum. It was better adapted for vascular lesions but because of its CW nature and relatively weak output its results were not really superior.
Pulsed and Q-switched Lasers
Pulsed lasers with an emission peak at 577 nm, where absorption by melanin is weaker, and with a pulse duration below the thermal relaxation time should be optimal to target superficial vessels without additional damage to surrounding structures. Q-switched lasers used in conjunction with preferentially absorbing pigments are optimal for melanotic lesions.
The flash-lamp pumped pulsed dye laser (PDL) with rhodamin G as the lasing medium emits light between 577 and 585 nm at a pulse duration of 450 μs. This is below the thermal relaxation time and thus no heat conduction occurs. Side effects are therefore rare except for transitory purpura lasting for about 2 weeks. These purpuras are due to the impact of the power being confined to a short time scale and can be prevented by using a long-pulse dye laser. A comparative trial with fluence of 1 J/cm 2 below and 0.5 J/cm 2 above the purpura threshold showed that clearance of telangiectasiae is possible with both modes. However, a longer pulse requires higher power densities, which are achieved with a frequency doubled Nd-YAG laser. This allows pulse widths of 1–30 ms and fluence rates of 15–20 J/cm 2 .
A novel approach is the combination of a pulsed dye laser with a Nd-YAG laser. It has been shown that blood changes its absorption characteristics during subpurpuric photocoagulation, due to methemoglobin generation increasing infrared absorption 3–5 fold. This allows the energy densities of both lasers to be reduced, thus minimizing potential side effects such as scars. A study using a device with one hand-piece, delivering PDL light of 595 nm (10 J/cm 2 , 10 ms pulse width, 7 mm spot size) and 100 ms later another pulse of 1064 nm Nd-YAG (70 J/cm 2 , 15 ms, 7 mm) was significantly more effective than either laser alone.
Pigments, both endogenous melanin as well as tattoo ink, are the main target for the so-called pigment lasers such as ruby and alexandrite. Melanin absorbs from 300–1064 nm, but minimizing interference with blood while still maintaining relatively high absorption makes a wavelength of 510 nm most suitable. Although not at precisely this wavelength, the light from ruby and alexandrite lasers is absorbed at a wavelength where absorption by hemoglobin is virtually zero, allowing melanin and tattoos to be effectively treated. Although ruby laser light at 694 nm is maximally absorbed with minimal absorption by hemoglobin and water, its light pulses are too short to damage hair follicles sufficiently for it to be useful in hair removal. The QS Nd-YAG laser penetrates deeper and it therefore is now also widely used for hair removal as well as the removal of dermal melanin and black tattoos.
Non-ablative lasers minimize the risks of temporary and permanent scarring because they leave the epidermis intact while delivering dermal irradiation with concomitant surface cooling. The absence of epidermal damage is associated with the absence of a true wound-healing response and therefore non-ablative lasers have minimal resurfacing efficacy. Because of this, multiple treatment sessions are required and there is variable and modest clinical improvement.
Fractional (Pixellated) Lasers
Fractional lasers are novel devices the use of which is grounded in the theory of selective photothermolysis. Their use involves creation of microscopic treatment zones (MTZs) of controlled width, depth and densities, which are surrounded by viable skin.
While ablative lasers produce excellent skin rejuvenation but with considerable downtime, non-ablative photorejuvenation is much less efficacious but has the advantage that there is no downtime. Ablative and non-ablative lasers both create large macroscopic zones of thermal damage, which are defined by the laser spot size. A new type of laser light delivery with patterns of 100 to over 1500 microscopic treatment zones/cm 2 was developed both with non-ablative lasers and with CO 2 and Er-YAG lasers. Small channels of controlled width, depth and densities of connective tissue damage are produced that are less than 200 μm wide. Within 1 hour of non-ablative laser irradiation, well-defined columns of both epidermal and dermal thermal damage are seen, with an intact overlying stratum corneum. Within 24 hours, viable cells migrate from the periphery toward the MTZs and microscopic epidermal necrotic debris (MEND) is formed. MEND comprises epidermal and dermal thermally damaged cells as well as melanin and elastin. It is extruded transepidermally within 3–7 days. Cellular markers of dermal wound healing and neocollagenesis are expressed within the treatment areas.
Fractional ablative lasers produce tiny holes reaching into the epidermis and upper dermis which are surrounded by normal skin. They increase in depth and width with increasing pulse energies. Channels up to 1600 μm deep can be safely made with the CO 2 or Er-YAG fractional lasers. The effect of ablative fractional lasers is much more pronounced, but healing takes at least a week.
Hair Removal Lasers
Hair density and distribution are important factors in psychosocial relations. Both too little and too much hair can be extremely embarrassing.
Hair removal with lasers is one of the most popular cosmetic treatment methods. Depending on hair color – the darker the better – and skin complexion – the lighter the better – long-lasting results are usually achieved after 5–10 sessions.
Long-lasting to almost permanent removal of unwanted hair has become possible with new laser devices. They are mainly based on long-pulsed alexandrite, diode and Nd-YAG lasers or intense pulsed light (IPL) sources, and by 2004 more than 22 different types of machine were available. It has been shown that photoepilation is much more efficacious than needle epilation, hurts less, is about 60 times faster and the results are aesthetically better. However, skillful photoepilation requires a great deal of experience.
The basis of photoepilation is the interaction of light and its target. About 5% of light is reflected by the stratum corneum. Light scatter on tissue inhomogeneities is the most important cause of energy loss because the laser beam loses its collimation and coherence (see Fig. 5.1 ). This loss can be reduced by choosing a greater spot size. Laser wavelength is another important variable: the longer the wavelength the deeper the penetration, up to about 1050 nm, when absorption by tissue water begins to increase. Choosing the right wavelength allows the target structure to be specifically heated without damaging the neighboring structures; this phenomenon is called selective photothermolysis. Follicular melanosomes are believed to be the targets of laser and IPL epilation, and dendritic melanocytes have also been found in the bulge, the region of the follicular stem cells. In the hair bulb, most melanin is present in anagen phases III–VI and this is the phase to be chosen for the treatment. Since only about 50–70% of facial hair is in anagen, multiple treatment sessions are necessary for a good result.
Intense Pulsed Light Sources
IPL has a broad spectrum of wavelengths potentially targeting several skin structures. It is used for vascular and pigment lesions as well as hair removal and photorejuvenation.
While the first IPL device was a very complex machine, demanding expert skills in order to achieve good results, the newer units are simpler to operate. They usually have at least two different treatment windows in order to either target hemoglobin or melanin. For hair removal and induction of collagen neosynthesis, longer wavelengths in the near infrared are preferred. The light pulses are commonly divided into two or three portions that are temporally spaced so that the surrounding tissue can cool down while the target remains hot, thus adding heat to the target with each subpulse.
IPL has, until now, been associated with minor and only transient side effects. Pain, erythema, hypo- or hyperpigmentation, and superficial crust or vesicle formation have been the most commonly reported adverse effects in applications for both photorejuvenation and depilation. The potential for ocular side effects has been alluded to, with previously reported cases of facial treatments deliberately avoiding direct application of IPL to the upper lids and protecting them from exposure by small external plastic shields.
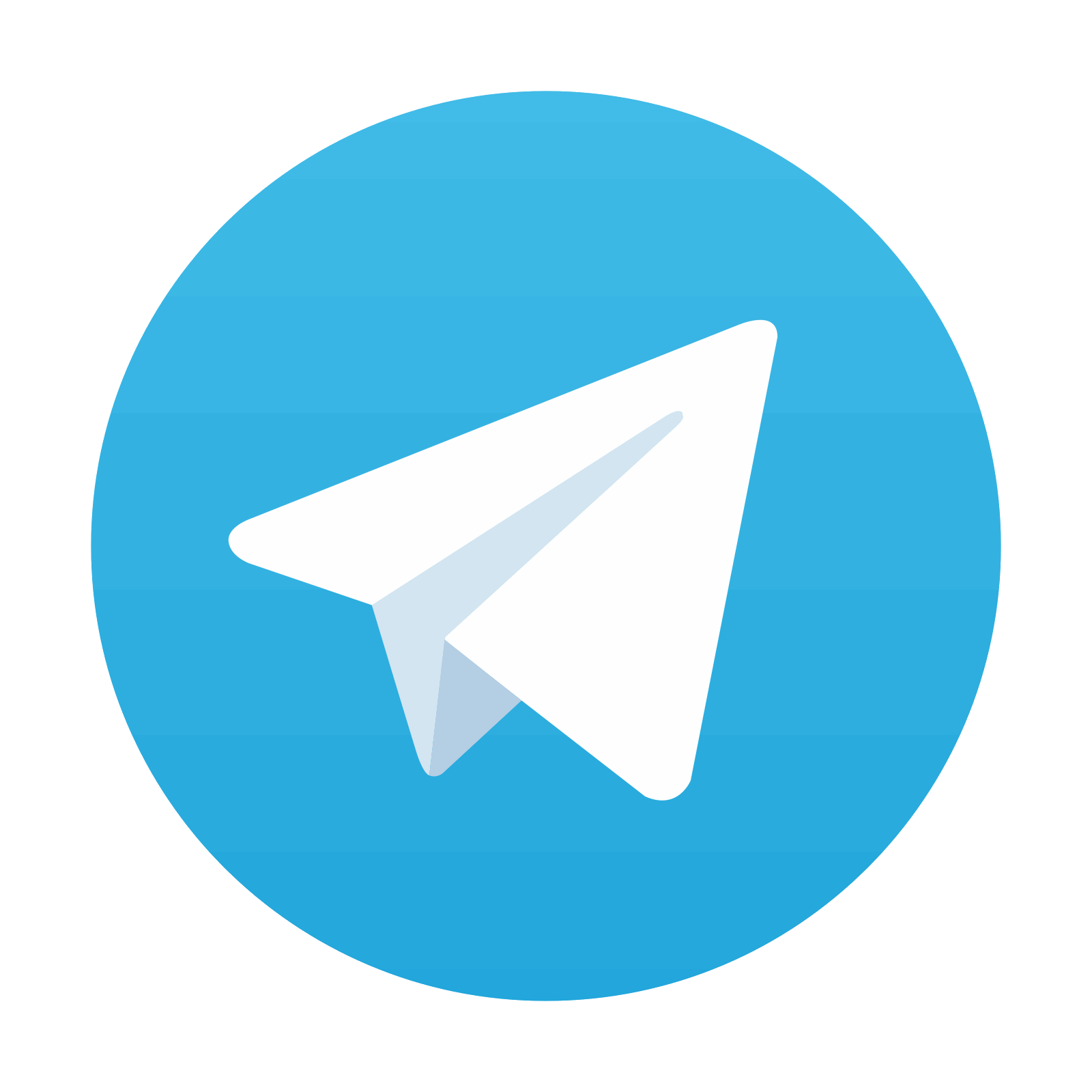
Stay updated, free articles. Join our Telegram channel

Full access? Get Clinical Tree
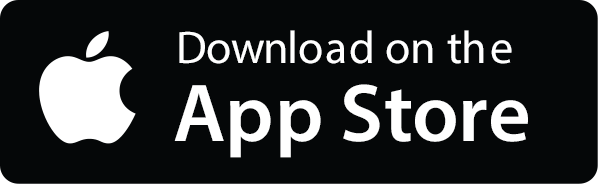
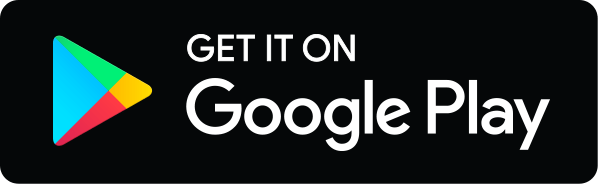