Introduction
Unlike methods of surface photoablation, the laser in situ keratomileusis (LASIK) technique requires a superficial corneal flap to be created prior to photoablation with an excimer laser. Mechanical microkeratomes and femtosecond lasers have been the two methods for LASIK flap creation. Safety is excellent with both methods, although the risk of complications cannot be eliminated. Femtosecond technology has allowed greater safety and studies have shown that compared with mechanical microkeratomes, femtosecond lasers provide more predictable flap dimensions. In theory, femtosecond lasers are advantageous because they can create precise cutting to allow variation of flap width, depth, hinge, and side cuts. However, femtosecond lasers can also lead to unforeseen complications.
In this chapter, we provide a general description of mechanical microkeratomes, followed by a detailed description of femtosecond lasers. Studies comparing the femtosecond lasers and mechanical microkeratome are discussed. Additionally, newer applications for the femtosecond laser, such as small incision lenticule extraction (SMILE) will be described.
Mechanical Microkeratomes
In 1958, José Ignacio Barraquer, the father of modern corneal refractive surgery, unveiled his first manual microkeratome, which he had designed for keratophakia and freeze keratomileusis. Until 1984, the microkeratome was used to cut a free corneal flap, which was frozen, cryolathed on its posterior surface, thawed, and then sutured back in place.
1985 saw the introduction of the nonfreeze keratomileusis technique by Krumeich and Swinger. The lenticule was processed unfrozen on its stromal side with a Barraquer-Krumeich-Swinger (BKS) refractive system 1000 refractive set.
In 1986, Ruiz introduced the in situ keratomileusis technique, in which a microkeratome was used to make two consecutive cuts with a diameter and depth that varied according to the degree of ametropia, using a set of suction rings of different sizes, calibrated applanation lenses, and various plates. Motorized microkeratomes, of which the Castroviejo electrokeratome (unveiled in 1963) was the precursor, became practical in 1991 with Ruiz and Lenchig’s automated corneal shaper (ACS; Chiron; Fig. 8.1 ), which made more reproducible cuts. The ACS was equipped with a system of gears to ensure a constant rate of movement on the suction ring to create a corneal flap with a nasal hinge. The height of the suction ring could be adjusted to vary the diameter of the second cut, avoiding the need to change rings. However, this automated lamellar keratoplasty (ALK) technique was imperfect and poorly reproducible. It was abandoned in 1995 in favor of LASIK, in which photoablation with a 193-nm excimer laser replaces the second refractive mechanical cut. The first human LASIK treatment was done in 1990.

In 1996, the Carriazo–Barraquer pivoted rotating microkeratome (CB) appeared on the market, with the theoretical advantage of allowing the hinge to be placed wherever desired. The use of an upper hinge limited the risk of flap displacement during blinking. Moria, the company that manufactured the device, also marketed the first disposable adaptable microkeratome heads. In 1997, Chiron released the Hansatome automated microkeratome, which allowed a corneal flap with an upper hinge. In 2001, Carriazo unveiled the first generation of pendular microkeratomes (Carriazo Pendular, Schwind). The cutting head moves vertically rather than horizontally, and the pendular motion requires a slightly lower cutting pressure than do conventional microkeratomes, leading to less mechanical friction and smaller size. Perhaps the most important advance in corneal flap creation technology is the introduction of the femtosecond laser microkeratome ( Fig. 8.2 ).

Pallikaris developed an alternative modality of epithelial separation with a blunt oscillating separator similar to a mechanical microkeratome used for LASIK flaps, which he called Epi-LASIK–Centurion . In contrast to laser-assisted subepithelial keratectomy (LASEK), this surgical approach does not require the use of alcohol for epithelial loosening.
Mechanical microkeratomes consist of the following basic components: (1) a peripheral part ( Fig. 8.3 ) that includes the suction ring, the microkeratome head, and the drive unit; (2) a central unit, which delivers the calibrated energy necessary to power the motors; and (3) connections/accessories that include two pedals, one to start and stop the vacuum and the other to control blade oscillation.

Most microkeratomes are fully reusable after dismantling, cleaning, and sterilization, apart from the blade, which is always single use. The single-use components can include all the peripheral components (suction ring, preassembled head and blade, and handpiece; Steritome); the head alone, equipped with a preassembled blade (CBSU and M2SU); and both the head (with the preassembled blade) and the suction ring (LSK One Use and One Use-plus).
Most mechanical microkeratomes require the use of both hands, one holding the suction ring and the other the microkeratome. Additionally, all mechanical microkeratomes have some safety devices, for example, a manually adjustable hinge stop (LSK One, M2, and M2SU), and a tunnel-shaped ring protecting the cutting head progression against mechanical obstruction.
Laser Microkeratomes: The Femtosecond Lasers
The safety of mechanical microkeratomes has improved markedly over the years but complications still occur, especially in patients with thin, flat, or steep corneas. In addition, the mechanical nature of the cut can be off-putting for the patient. Preparation of the corneal flap with a femtosecond laser appears to overcome these problems ( Fig. 8.3 ). Flap size, flap thickness, edge angle, hinge width, and hinge location are more controlled with the computer-guided femtosecond laser platforms.
Physical Principles of the Femtosecond Laser
The femtosecond laser delivers ultrashort pulses lasting about 10 −15 s. Its wavelength is situated in the infrared (about 1000–1053 nm). As the cornea is infrared transparent, femtosecond laser energy is not absorbed by the cornea, contrary to the excimer laser beam. The main feature of femtosecond lasers is that high powers can be obtained with low-energy pulses. Higher energies would immediately destroy the laser components.
Several femtosecond laser systems are available today, including the IntraLase (Abbott Medical Optics, Inc.), the Wavelight (Alcon Surgical, Inc.), the Visumax (Carl Zeiss Meditec AG), the Victus (Bausch & Lomb Technolas GmbH), and the Femto LDV (Ziemer Ophthalmic Systems AG) ( ). Newer-generation femtosecond platforms with higher frequency (60 kHz, 150 kHz, 500 kHz) produce more predictable LASIK flaps with a more uniform flap interface and less inflammation. The IntraLase was the first commercially available platform. The system has been continuously updated with changes in the laser pulse rate (from 15–30 to 60–150 kHz). Laser energy is created through Nd:glass (amplification glass matrix mixed with neodymium). Emission of low-energy pulses necessitates a pulse amplifier placed downstream of the generator (chirped pulse amplification [CPA] system). The delivery system, composed of two perpendicular galvanometers (rotary mirrors), allows 3-dimensional scanning of the laser. The beam is focused to the desired corneal depth by an aspheric convergent lens.
The Visumax is another widely used system. Multiple studies have compared the IntraLase to the Visumax. No significant differences were found in safety, efficacy, and predictability. The efficiency of an eye tracker after LASIK flap creation among the two systems was also evaluated with no significant differences. Using higher-frequency lasers, Yu and Manche in two prospective studies compared the morphologic features of LASIK flaps created with the FS 60 kHz and the iFS 150 kHz, and the visual outcomes. With regard to morphology, both lasers produced uniform planar flaps, but mean thickness was greater with the IntraLase 60 kHz. With regards to visual outcomes, while both systems produced excellent visual results, a higher intraoperative patient preference and a trend toward faster visual recovery was observed with the IntraLase 150-kHz platform.
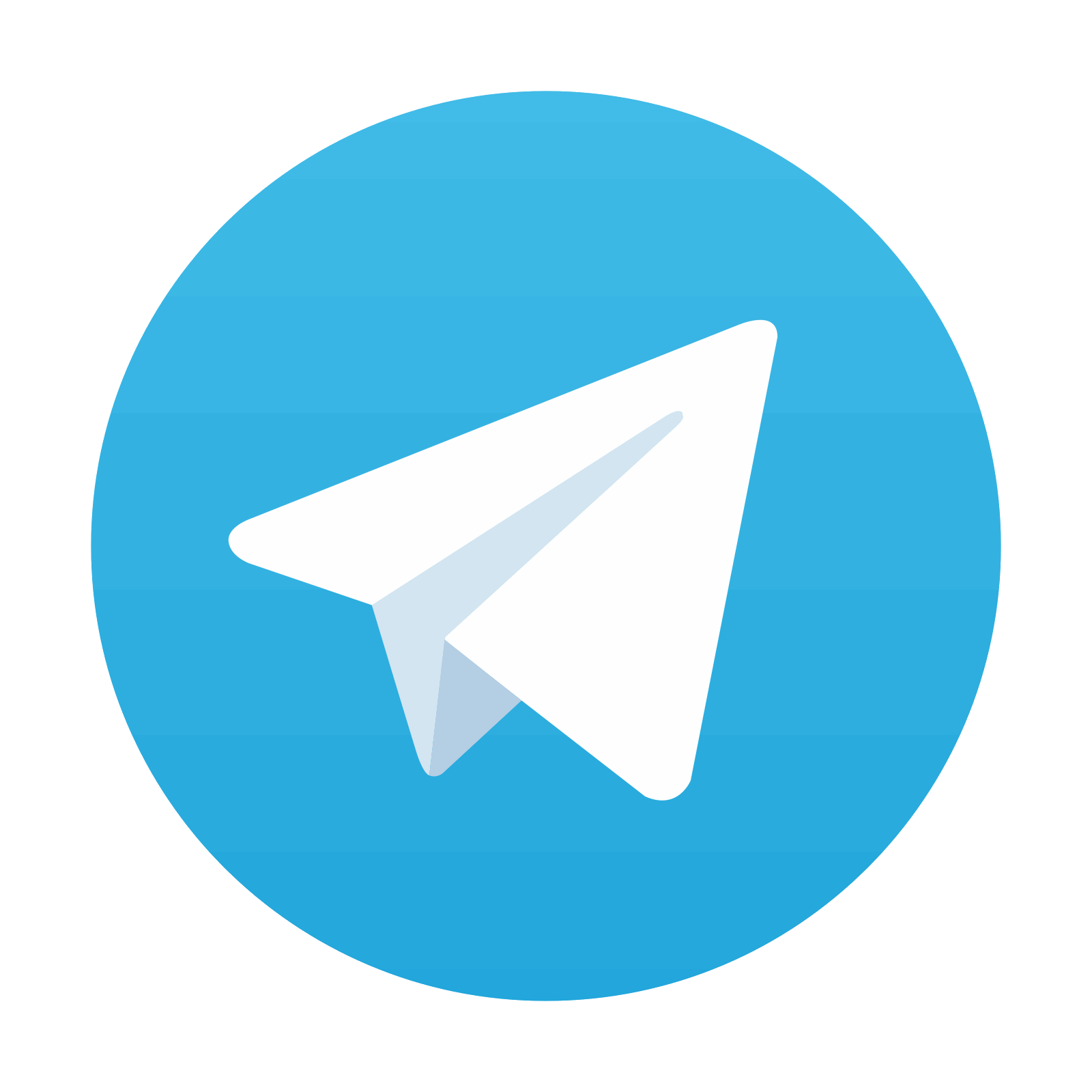
Stay updated, free articles. Join our Telegram channel

Full access? Get Clinical Tree
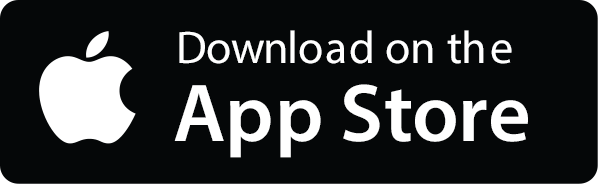
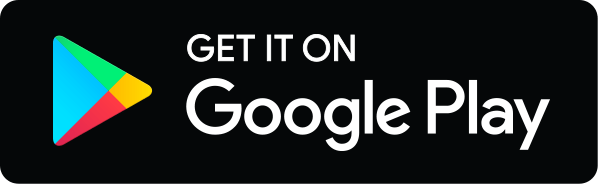