Purpose: We sought to characterize juxtapapillary (JP) and non-JP microvasculature dropout in patients with primary open-angle glaucoma and to compare their rate of retinal nerve fiber layer (RNFL) thinning.
Design: Retrospective cohort study.
Methods: A total of 141 eyes with primary open-angle glaucoma with ≥4 serial optical coherence tomography (OCT) images after initial OCT angiography for ≥2 years were included. Based on OCT angiography imaging, the 3 groups were matched by age and visual field mean deviation: JP group (parapapillary deep-layer microvasculature dropout in contact with the optic disc boundary, n = 47), non-JP group (dropout not reaching the optic disc boundary, n = 47), and no-dropout group (lacking the dropout, n = 47). The RNFL thinning rate was compared among the 3 groups.
Results: The rate of RNFL thinning tended to be fastest in the JP group followed by the non-JP group and no-dropout group in all areas except the temporal and nasal sectors. Post hoc analysis revealed that the JP group had significantly faster RNFL thinning than did the no-dropout group in the global area and the inferotemporal and inferonasal sectors ( P < .05). When subgroup analysis was performed for subjects in which the main sector of dropout was the inferotemporal sector, the JP group had significantly faster RNFL thinning than the other 2 groups in the corresponding inferotemporal sector ( P < .001).
Conclusion: Eyes with JP microvasculature dropout showed faster RNFL thinning than eyes without dropout. These findings suggest that deep-layer microvasculature dropout, especially in contact with the optic disc boundary, is associated with rapid glaucoma progression.
Glaucoma is a chronic optic neuropathy characterized by progressive optic nerve head (ONH) damage, such as neuroretinal rim thinning and excavation of the optic cup. Among the multiple proposed mechanisms of optic nerve damage, microvascular changes of the ONH have been proposed as a potential factor in the development and progression of glaucoma. , The peripapillary choroidal microvasculature is of particular interest in understanding whether there is a vascular mechanism contributing to the pathogenesis of glaucoma because it is supplied by the short posterior ciliary arteries that also perfuse the deep ONH tissue. Recently, optical coherence tomography angiography (OCT-A) has been shown to be able to identify parapapillary deep-layer microvasculature dropout. , Moreover, several studies have shown that OCT-A–derived deep-layer microvasculature dropout is related to progressive retinal nerve fiber layer (RNFL) and visual field (VF) changes in patients with glaucoma.
Typically, parapapillary deep-layer microvasculature dropout is observed in the juxtapapillary (JP) area adjacent to the clinical optic disc margin. In addition, other studies have found a distinctive type of microvasculature dropout in the non-JP area. Moreover, there is an increasing amount of evidence that non-JP dropout that is not in contact with the optic disc margin is associated with stretching of the sclera and Bruch membrane (BM) retinal pigment epithelium (RPE) rather than glaucomatous damage. , However, it is still unclear whether the rate of glaucoma progression differs according to the type of deep-layer microvasculature dropout.
The current study compared the rate of RNFL thinning among 3 groups: glaucomatous eyes without microvasculature dropout, eyes with JP dropout, and eyes with non-JP dropout.
Methods
This retrospective observational study was approved by the institutional review board of Haeundae Paik Hospital (Busan, South Korea). It included patients with primary open-angle glaucoma (POAG) who had been followed up for ≥2 years after initial OCT-A and spectral-domain OCT imaging. Both perimetric and preperimetric POAG showed the presence of glaucomatous optic nerve damage (ie, focal thinning, notching, and localized or diffuse atrophy of the RNFL) with an open angle. Patients with perimetric POAG had glaucomatous VF damage defined as a VF outside the normal limits in the glaucoma hemifield test, or 3 abnormal nonedge points in the pattern deviation plot with a P < .05 of being normal, with one having a P < .01, or pattern standard deviation (PSD) outside 95% normal limits as confirmed on 2 consecutive, reliable tests (fixation loss rates ≤33%, false positive and false negative rates ≤15%). Preperimetric POAG eyes did not have evidence of glaucomatous VF defects despite detectable glaucomatous ONH damage. All subjects were required to have β-zone parapapillary atrophy (βPPA). Eyes without βPPA were excluded from the study because deep-layer microvasculature dropout is difficult to detect in an area with an intact RPE.
All of the participants underwent a complete ophthalmic examination, including measurement of best-corrected visual acuity, refraction tests, slit-lamp biomicroscopy, Goldmann applanation tonometry, gonioscopy, central corneal thickness measurement with the Pentacam Scheimpflug imaging system (Oculus Optikgeräte GmbH, Wetzlar, Germany), axial length measurement by IOL Master (Carl Zeiss Meditec, Dublin, California, USA), dilated stereoscopic examination of the optic disc, simultaneous color and red-free fundus photography (TRC-NW8; Topcon, Tokyo, Japan), standard automated perimetry (Humphrey Field Analyzer; 30-2 Swedish Interactive Threshold Algorithm; Carl Zeiss-Meditec), and spectral-domain OCT and OCT-A (Spectralis; Heidelberg Engineering GmbH, Heidelberg, Germany). Systolic and diastolic blood pressure (BP) was measured at the height of the heart with an automatic BP instrument (Model Easy X 800 [R/L]; JAWON Medical Co. Ltd., Kyungsan, Korea). Mean ocular perfusion pressure was calculated as the difference between mean arterial pressure and intraocular pressure (IOP), mean arterial pressure having been calculated as diastolic BP plus 1/3 (systolic BP – diastolic BP). The presence of disc hemorrhage (DH), defined as an isolated splinter- or flame-shaped hemorrhage on the ONH, was determined by 2 blinded observers (M.H.S., J.M.K) based on a standardized review of fundus photographs and clinical examinations performed at intervals of 3 to 6 months. , Baseline IOP was defined as IOP measured before the initiation of IOP-lowering treatment. The mean IOP and standard deviation IOP during the entire follow-up period were calculated. To avoid the undesired effect that numerous sequential IOP measurements taken during a short period would have on the final average, the average IOP for each 6-month period was used to calculate the mean follow-up IOP. , All IOPs measured 4 weeks after any type of incisional surgery or laser procedure were excluded to avoid the effect of transitory IOP changes during this period.
All subjects were required to have ≥4 OCT RNFL thickness measurements after initial OCT-A imaging during the ≥2-year follow up. The initial spectral-domain OCT and OCT-A scans were conducted within a 3-month interval.
Spectral-Domain OCT Imaging
The presence and microstructure of βPPA were determined by synchronous viewing of the infrared fundus image and ONH radial circle scans using Spectralis OCT2 Glaucoma Module Premium Edition software (version 1.9.17.0). βPPA was defined as the PPA without RPE, while βPPA −BM was defined as the PPA area with exposed scleral flange without BM; both had to have a temporal width of ≥100 µm on ≥1 radial scan. The width of βPPA with BM (βPPA +BM ) was obtained by subtracting the width of βPPA −BM from the width of the entire βPPA. The average widths of βPPA −BM and βPPA +BM were calculated using the built-in caliper from 6 radial scans of which the center was located at the fovea–BM opening (BMO) axis. Focal lamina cribrosa (LC) defects defined as laminar holes or laminar disinsertions violating the normal U- or W-shaped contour of the anterior laminar surface were determined by review of enhanced depth imaging spectral-domain OCT ONH 20-degree × 20-degree scans consisting of 48 radial B-scans centered on the optic disc. ,
Two blinded observers (J.M.K., D.H.J) evaluated the presence and width of βPPA +BM , βPPA −BM , and focal LC defects in a blinded fashion. For the presence of βPPA +BM , βPPA −BM , and focal LC defects, disagreements were resolved by a third adjudicator (M.H.S.) and subjects were excluded if agreements could not be reached. As for the width of βPPA +BM and βPPA −BM , the mean values of the 2 observers were used in the final analysis.
BMO area and RNFL thickness were measured from ONH radial circle scans using standard Spectralis software. Using the 3.5-mm diameter RNFL circle scan from the ONH radial circle, circumpapillary RNFL thickness was calculated as global thickness and in 6 sectors (superotemporal [TS], 46–90 degrees; inferotemporal [TI], 271–315 degrees; temporal [T], 316–45 degrees; superonasal [NS], 91–135 degrees; inferonasal [NI], 226–270 degrees; and nasal [N], 136–225 degrees). In the case of segmentation errors, the RNFL segmentation was manually corrected by an observer (J.M.K.) blinded to the patients’ information. The rate of RNFL thinning for each sector was derived by linear regression analysis over time (expressed in micrometers per year).
Parapapillary Deep-Layer Microvasculature Dropout
The Spectralis OCT-A module incorporated into the OCT2 platform, with a central wavelength of 880 nm, an acquisition speed of 85 kHz, and lateral and axial resolutions of 5.7 µm and 3.9 µm per pixel, respectively, was used to visualize the parapapillary deep-layer microvasculature. Scans were obtained from a 15-degree × 10-degree scan pattern consisting of 256 clusters of 5 repeated B-scans centered on the optic disc. OCT-A images judged to be of poor quality by 2 observers (M.H.S., J.M.K.) according to the following criteria were excluded: 1) quality score <25; 2) poor clarity; 3) residual motion artifacts visible as an irregular vessel pattern or disc boundary on the enface angiogram; 4) local weak signal; or 5) choroidal layer segmentation errors.
Parapapillary deep-layer microvasculature dropout was defined as complete loss of the choriocapillaris or microvasculature contained in the scleral flange within the βPPA based on a qualitative OCT-A analysis. Full details on the determination of dropout are available elsewhere. Briefly, dropout was required to be present in ≥4 consecutive horizontal scans, with a diameter of >200 µm in ≥1 scan. Dropout was classified as JP when it was present in both the choroid and border tissue of Elschnig and was in contact with the optic disc boundary ( Figure 1 , A2 through A5) and as non-JP when it was present only in the choroid and did not reach the optic disc boundary ( Figure 1 , B2 through B5). Clinical optic disc margin was defined as the innermost border of hyperreflective tissue internal to the pigmented tissue on both infrared fundus images and OCT ( Figure 1 , A1 through A5, B1 through B5, and C1 through C5). Eyes were assigned to the no-dropout group when no visible deep-layer microvasculature dropout was observed ( Figure 1 , C2 through C5). One eye from each patient was included in the analysis. If both eyes of a patient were included in the same group, 1 eye was randomly selected. If both eyes were eligible for different groups, they were assigned preferentially to the non-JP group, followed by the no-dropout and JP groups. Both eyes of 53 patients were eligible for different groups. Among them, 21 patients had JP dropout in 1 eye and non-JP dropout in the other eye, 20 had JP dropout in 1 eye and no dropout in the other eye, and 12 had non-JP dropout in both eyes. The 3 groups were matched by age and VF mean deviation (MD) using the individual matching method. The circumferential location of the dropout was determined based on the circumpapillary scanning circle as divided by the Spectralis OCT system as well as the hemispheric location ( Figure 2 , A2). Of the 6 sectors (TI, TS, NS, N, NI, and T), 5 sectors (TI, TS, NS, N, and NI) were adjusted according to those of the OCT-derived circumpapillary scanning circle. If the location of the dropout corresponded to T, it was divided into the upper-temporal (UT) or lower-temporal (LT) sector based on the fovea–BMO center ( Figure 2 , A2). All 7 sectors (TI, TS, NS, N, NI, UT, and LT) involved in dropout were documented and included in the analysis. The main microvasculature dropout sector was determined as the one with the largest proportion of dropout in each of superior and inferior hemispheres, respectively ( Figure 2 , A2). Two independent observers (M.H.S., J.M.K.) blinded to the patients’ characteristics and optic disc features independently identified the presence and location of dropout. Disagreements were resolved by consensus and subjects were excluded if consensus could not be reached.


Statistical Analysis
Baseline characteristics and test results including the rate of RNFL thinning were compared among the eyes of the JP, non-JP, and no-dropout groups by 1-way analysis of variance. To adjust for multiple comparisons between groups within each analysis, the Tukey-Kramer honest significant difference (HSD) post hoc test was performed. Longitudinal differences of RNFL thinning rate were assessed with linear mixed effects models with time, type of the deep-layer microvasculature dropout, and mean IOP, a parameter associated with the glaucoma progression. Categorical variables were compared with the χ 2 test. To measure the interobserver agreement for microvasculature dropout determination, focal LC defect, and DH, kappa values were derived. The interobserver reproducibility of the measurement of βPPA +BM and βPPA −BM width was assessed by intraclass coefficient. Analyses were conducted using MedCalc (MedCalc, Inc., Mariakerke, Belgium) and statistical software R version 3.6.3 (R Foundation for Statistical Computing, Vienna, Austria). P < .05 was considered statistically significant.
Results
Among the 198 eyes meeting the eligibility criteria, 57 were excluded because of poor OCT-A image quality (n = 9), poor OCT quality (n = 5), lack of βPPA (n = 27), and disagreement between the 2 observers in their determination of the dropout pattern (n = 19). One hundred forty-one eyes were included in the analysis.
There was good interobserver agreement for determination of the microvasculature dropout pattern (κ = 0.809), focal LC defect (κ = 0.809), and DH (κ = 0.837). There was good interobserver reproducibility for measurement of βPPA +BM width (intraclass correlation coefficient, 0.95) and βPPA −BM width (intraclass correlation coefficient, 0.97).
The baseline characteristics of the subjects are shown in Table 1 . The 3 groups differed in terms of axial length, number of topical glaucoma medications, βPPA +BM width, βPPA −BM width, and baseline RNFL thickness in the temporal and inferotemporal sectors ( P < .05). Post hoc testing revealed that the non-JP group had significantly longer axial length ( P < .001) and thicker RNFL in the temporal sector ( P = .005) than the other 2 groups. The JP group had significantly thinner RNFL than the no-dropout group in the TI sector ( P = .006). The number of topical glaucoma medications was higher in the JP group than the other 2 groups ( P = .037). The widths of βPPA +BM ( P = .001) and βPPA −BM ( P < .001) were significantly smaller in no-dropout group than in the other 2 groups. The other baseline parameters, including age, central corneal thickness, the number of preperimetric and perimetric glaucoma eyes, baseline and mean IOP, baseline VF MD and PSD, baseline RNFL thicknesses in all areas except temporal and inferotemporal sectors, BMO area, DH, and focal LC defect, did not differ among the 3 groups ( P > .05).
Variables | Group 1, Juxtapapillary (n = 47) | Group 2, Non-Juxtapapillary (n = 47) | Group 3, No Microvasculature Dropout (n = 47) | P Value | Post Hoc a |
---|---|---|---|---|---|
Age (y) | 56.7 ± 16.1 (25–88) | 55.4 ± 12.5 (28–84) | 61.2 ± 12.8 (32–79) | .110 b | |
Gender (male/female) | 24/23 | 21/26 | 17/30 | .350 c | |
Self-reported diabetes, n (%) | 5 (10.6) | 6 (12.8) | 12 (25.5) | .107 c | |
Self-reported hypertension, n (%) | 9 (19.1) | 9 (19.1) | 11 (23.4) | .841 c | |
Diabetes medication, n (%) | 5 (10.6) | 6 (12.8) | 12 (25.5) | .107 c | |
Antihypertensive medication, n (%) | 9 (19.1) | 9 (19.1) | 11 (23.4) | .841 c | |
Follow-up period (y) | 2.4 ± 0.3 | 2.5 ± 0.3 | 2.6 ± 0.3 | .059 b | |
Axial length (mm) | 24.6 ± 1.8 | 26.0 ± 1.4 | 24.2 ± 1.4 | <.001 b , d | 1 = 3 < 2 |
Central corneal thickness (µm) | 536.4 ± 35.9 | 538.1 ± 40.2 | 538.5 ± 38.2 | .962 b | |
Type of glaucoma, no. of eyes | .418 c | ||||
Preperimetric | 10 | 15 | 15 | ||
Perimetric | 37 | 32 | 32 | ||
Topical glaucoma medications, n (%) | .037 c , d | 1 > 2 = 3 | |||
0 | 3 (6.4) | 7 (14.9) | 8 (17.0) | ||
1 | 5 (10.6) | 12 (25.5) | 14 (29.8) | ||
>1 | 39 (83.0) | 28 (59.6) | 25 (53.2) | ||
IOP (mm Hg) | |||||
Baseline | 18.1 ± 5.8 | 16.2 ± 4.3 | 17.5 ± 4.8 | .217 b | |
Mean | 13.5 ± 2.1 | 12.7 ± 2.1 | 13.6 ± 2.3 | .061 b | |
SD | 2.2 ± 1.7 | 1.9 ± 0.7 | 2.1 ± 0.9 | .316 b | |
Visual field measurements | |||||
Number, n | 4.5 ± 0.9 | 4.2 ± 0.9 | 4.0 ± 0.9 | .080 b | |
Baseline MD (dB) | −3.2 ± 2.5 | −2.5 ± 2.9 | −2.4 ± 3.9 | .441 b | |
Baseline PSD (dB) | 4.8 ± 2.8 | 4.2 ± 3.4 | 3.7 ± 3.6 | .237 b | |
Baseline RNFL thickness (µm) | |||||
Global | 79.2 ± 13.4 | 82.7 ± 11.9 | 83.5 ± 15.2 | .269 b | |
Superotemporal | 109.0 ± 35.9 | 113.0 ± 30.8 | 108.7 ± 25.7 | 0.750 b | |
Temporal | 64.2 ± 17.7 | 75.7 ± 21.5 | 66.4 ± 12.9 | .005 b , d | 1 = 3 < 2 |
Inferotemporal | 87.7 ± 36.8 | 103.5 ± 35.3 | 111.1 ± 34.2 | .006 b , d | 1 < 3 |
Inferonasal | 85.0 ± 21.9 | 87.5 ± 24.9 | 94.7 ± 29.9 | .175 b | |
Nasal | 67.4 ± 14.8 | 64.1 ± 19.3 | 67.1 ± 14.8 | .553 b | |
Superonasal | 105.8 ± 26.1 | 102.6 ± 26.3 | 102.3 ± 33.1 | .807 b | |
βPPA +BM width (µm) | 230.4 ± 105.9 | 263.8 ± 144.1 | 174.6 ± 82.9 | .001 b , d | 1 = 2 > 3 |
βPPA −BM width (µm) | 211.1 ± 316.5 | 228.8 ± 209.8 | 36.1 ± 62.6 | <.001 b , d | 1 = 2 > 3 |
BMO area (mm 2 ) | 2.5 ± 0.7 | 2.4 ± 0.5 | 2.3 ± 0.4 | .109 b | |
Systolic BP (mm Hg) | 123.5 ± 16.2 | 117.8 ± 16.1 | 125.9 ± 17.0 | .086 b | |
Diastolic BP (mm Hg) | 73.4 ± 12.5 | 71.4 ± 12.2 | 72.4 ± 13.8 | .781 b | |
MOPP (mm Hg) | 47.1 ± 9.2 | 45.9 ± 8.0 | 47.1 ± 8.7 | .782 b | |
Disc hemorrhage, n (%) | 11 (23.4) | 5 (10.6) | 4 (8.5) | .082 c | |
Focal LC defect, n (%) | 20 (42.6) | 17 (36.2) | 12 (25.5) | .137 c |
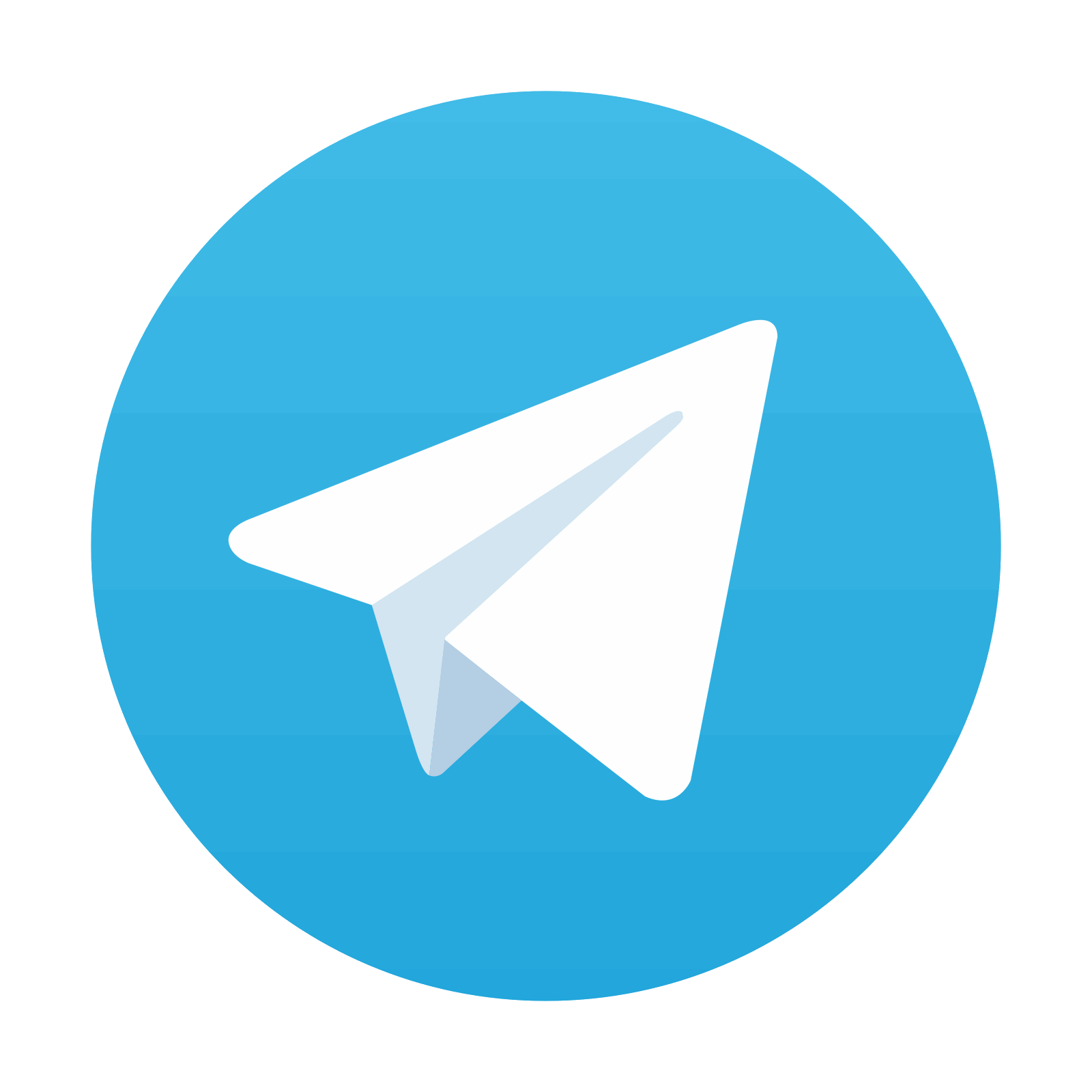
Stay updated, free articles. Join our Telegram channel

Full access? Get Clinical Tree
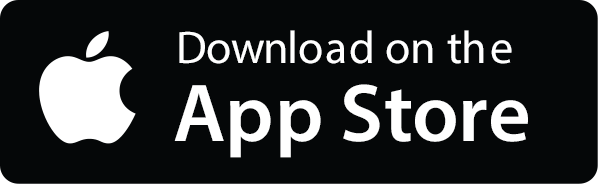
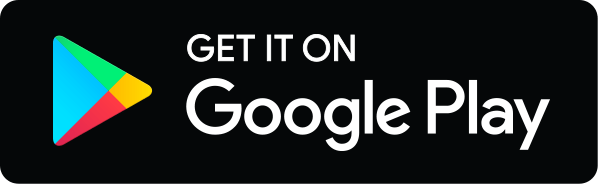
