Nature of Incomitant Deviations
In some deviations, strabismus or heterophoria, the angle of the deviation varies as the patient moves the eyes to look in different parts of the field. Such deviations are incomitant. For most causes of incomitancy, there is a consistency in the way the angle changes: it increases in one particular direction of gaze each time the eyes are turned in that direction. Also, the angle will differ according to which eye is fixating (p. 250). Incomitant deviations are usually caused by abnormalities in the anatomy of the ocular motor apparatus or by one or more muscles being unable to function normally. These deviations can either be congenital, or may be acquired at any age:
- 1.
Congenital incomitancy is due to some developmental anomaly of the motor system, either in the anatomy or in the functioning of the muscles or the parts of the nervous system that serve them. This type of deviation gradually becomes more comitant over time but is very much less likely to respond to eye exercises than comitant (concomitant) deviations.
- 2.
Acquired incomitant deviations are caused by injury or disease of the ocular motor system. For example, they may be the result of a fracture of the skull, or of pathology affecting the muscles, nerves, or brain centres. Such conditions may be long-standing, static, and requiring no further medical attention, or can be due to recently acquired injury or active disease process. In the latter case, the patient needs referral for medical attention to the ocular condition or to the disease causing the anomaly.
The complete loss of action of a muscle is called a muscle paralysis . A partial loss is referred to as paresis . The term palsy is used generically to include both paralysis and paresis. In incomitant deviations of all kinds, treatment with spectacles or exercises is very limited in remedying the patient’s deviation. The first priority is to recognise those cases which require urgent medical attention.
A refractive correction may be required by the patient but is likely to have minimal effect on the deviation. Incomitancy is present in about 13% of cases of strabismus, but is rarer in heterophoria ( Flom, 1990 ). Prolonged occlusion in normal subjects may reveal small incomitancies ( Neikter, 1994a ). It may be that there is a continuum between the extremes of perfect comitancy and frank incomitancy, with most people having a slight anatomical incomitancy which is only revealed by prolonged periods of dissociation.
Before detailing the investigation and management of incomitancies, the normal actions of the extraocular muscles will be reviewed.
Actions of the Extraocular Muscles
The basis of muscle actions arises from their anatomy ( Evans, 2004a ). Fig. 17.1 is a scale plan view of the orbit. The eye is in the primary position, so the visual axis is parallel to the medial wall of the orbit. The centre of rotation of the eye is marked (C). Fig. 17.1A shows the centre of the attachment of the superior rectus muscle is medial to the plane containing the visual axis. This muscle’s attachment is neither symmetrical nor quite central, and its general line of pull is slightly nasal to the plane containing the centre of rotation. This means that it does not act vertically over the centre of rotation and this influences the secondary actions of this muscle. By reference to Fig. 17.1A , when the eye is looking straight ahead the primary action must be to elevate the eye, and the secondary actions are adduction and intorsion. The secondary actions will increase on adduction.

On abduction of the eye, the attachment of the muscle will move outwards, and the line of pull will be carried directly over the centre of rotation when the eye is abducted by about 25 degrees. In this position, two factors are obvious: the secondary actions can no longer occur, and the primary action will be at its greatest mechanical advantage. When the eye is abducted by 25 degrees, the superior rectus muscle will be a pure elevator and at its maximum power as an elevator ( Fig. 17.2 ).

A similar state of affairs applies to the depressor action of the inferior rectus muscle, as this lies very nearly in the same vertical plane as the superior rectus. When the eye is turned out by about 25 degrees, the inferior rectus has its strongest action as a depressor and has no secondary actions. The primary action of the superior rectus muscle opposes that of the inferior rectus: the muscles are antagonists.
The oblique muscles are illustrated in Fig. 17.1B . From its attachment to the eye, the superior oblique pulls towards the trochlea (T). The line of pull is also medial to the centre of rotation, and its actions in the primary position, intorsion, depression, and abduction follow from this one anatomical detail. Also, as the eye turns inwards its vertical action (depression) is increased and the other actions are very much reduced. If the eye were to be adducted by about 50 degrees, the line of pull would lie in the same plane as the centre of rotation. In this position, its power as a depressor would be maximum, and it would have no other actions.
From the point of view of clinical diagnosis, we can regard the inferior oblique muscle as lying in the same vertical plane as the superior oblique. Its actions can therefore be deduced in a similar way and the primary actions of the two muscles are opposite, so they are antagonists.
The single anatomical detail from which the muscle actions arise is that the two vertical planes containing the lines of pull of the vertically acting pairs of muscles cross medially to the centre of rotation of the eye. Once this is understood, not only can the primary and secondary actions of these muscles be deduced, but also incomitant deviation can be analysed.
Fig. 17.2 shows the interaction of the two elevator muscles: the superior rectus and the inferior oblique. The central diagram shows the eye turned upwards from the primary position. Its elevation is maintained by the combined actions of both these muscles. Their individual contributions to the maintenance of elevation (E) is shown in the vector construction above the central diagram. The diagrams on the left of the figure show the way these two muscles contribute to elevation when the eye is turned outwards (abducted), and those on the right show the contribution of each when the eye is turned inwards (adducted). In the vector construction ( Fig. 17.2 ), the sloping line SR shows how the power of the superior rectus to elevate the eye is at its maximum when the eye is abducted, and declines as the eye moves across the top of the motor field to the adducted position. The other sloping line IO indicates that the reverse is true of the oblique muscle: its elevating power is at a minimum when the eye is abducted and increases as the eye adducts. One muscle gradually takes over from the other as the eye moves across the top of the field. Fig. 17.3 shows a similar treatment of the depressor muscles (the inferior rectus and the superior oblique) as the eye moves across the lower motor field in the depressed (D) positions.

The actions of the extraocular muscles in the primary position can be deduced from Figs. 17.1 and 17.2 and are given in Table 17.1 . It is stressed that the actions of each muscle will change as the eye moves away from the primary position. The cardinal diagnostic positions of gaze are important in interpretation of the ocular motility test (see later), but knowledge of the actions of the muscles in the primary position is required to interpret the results of the cover test carried out in the primary position. An easy way to remember the secondary and tertiary actions of the cyclovertical muscles is RadSin: recti adduct, superiors intort ( Hosking, 2001 ).
Muscle | Primary action | Secondary action | Tertiary action |
---|---|---|---|
Medial rectus | adduction | none | none |
Lateral rectus | abduction | none | none |
Superior rectus | elevation | intorsion | adduction |
Inferior rectus | depression | extorsion | adduction |
Superior oblique | intorsion | depression | abduction |
Inferior oblique | extorsion | elevation | abduction |
It has been suggested that fibroelastic sleeves, muscle pulleys , act as mechanical origins of the muscles and modify their actions ( Kono, Poukens, & Demer, 2002 ; Demer, 2006 ), although this has been disputed ( Jampel and Shi, 2006 ). In any event, a palsy or malfunction of one muscle will show as a failure of the eye to turn fully in the direction for which the muscle has the greatest mechanical advantage. For example, a palsy of the superior rectus muscle usually will be detected by the restricted movement when an attempt is made to elevate the eye when it is turned outwards ( Fig. 17.2 ).
It can also be noted that as the primary functions of the vertically acting muscles decrease, their secondary functions increase slightly. Thus, when the eye is turned down and inwards, the inferior rectus is pulling nearly at right-angles to the visual axis and plays little part in depression. However, its ability to adduct the eye is increased as is its ability to cyclorotate the eye (extorsion) ( Fig. 17.3 ).
The medial rectus muscle in each orbit is an adductor with little secondary function, and the lateral rectus is an abductor with little other function. These muscles are antagonists.
Muscle Pairs, Hering’s Law, and Sherrington’s Law
Within one eye, synergistic muscles move the eye in the same direction. For example, the superior rectus and inferior oblique are ipsilateral synergists for elevation (but they are not synergists for horizontal or torsional movements). Conversely, the pair of extraocular muscles that move the eye in opposite directions can be thought of as agonist/antagonist pairs . For example, the superior and inferior rectus muscles are antagonistic for vertical and torsional movements (but not for horizontal movements). Sherrington’s law of reciprocal innervation states that the contraction of a muscle is accompanied by simultaneous and proportional relaxation of its ipsilateral antagonist.
Sherrington’s law applies to the muscles of one eye. The movements of two eyes as a team are described by Hering’s law. Yoke muscles are pairs of muscles, consisting of one muscle from each eye, that produce simultaneous rotations of the eyes in either the same direction (conjugate movement) or opposite direction (disjugate movement). Hering’s law relates the innervation of a muscle in one eye (the agonist) to its yoked muscle in the other eye, the contralateral synergist. Normally, the agonist in one eye and its contralateral synergist move the eyes in the same direction (e.g., the right superior oblique is the contralateral synergist of the left inferior rectus). Hering’s law of equal innervation states that nerve impulses stimulating an agonist are equal to those stimulating its contralateral synergist.
Primary and Secondary Deviations
It was noted at the beginning of this chapter that if an incomitancy is present, the angle of deviation will differ according to which eye is fixating. This occurs because of Hering’s law of equal innervation and will be explained by the example in Fig. 17.4 . The top panel shows normal binocular fixation. ‘+’ signifies innervation to the lateral and medial recti muscles. In the second and third panels, the left lateral rectus has suffered a paresis. In the second panel the nonparetic (right) eye fixates, as is most commonly the case. There will be approximately equal innervation to the right lateral and medial recti, and therefore equal innervation to the left lateral and medial recti. Since the left lateral rectus is paretic, the left eye will be deviated inwards: the primary deviation .

In the bottom panel of Fig. 17.4 , the same left lateral rectus muscle is paretic but now the less common situation pertains, when the patient fixates with the paretic eye. Excessive innervation (+++) is required to the paretic left lateral rectus to maintain fixation in the primary position, and inhibition to the left medial rectus (−−−). Hering’s law means that the nonparetic right eye will also receive much greater innervation to the right medial rectus causing a very large secondary deviation , which is greater than the primary deviation that resulted when the nonparetic eye was fixating.
The difference between primary and secondary deviations has several clinical manifestations. During the cover test in the primary position, the size of the deviation when each eye is covered can be compared and if the deviation differs, this indicates the patient may have an incomitant deviation and can also indicate which eye has the under-acting muscle. Later in this chapter the use of the Maddox rod for comparing primary and secondary deviations will be discussed. The difference between primary and secondary deviations also explains why Hess and Lees screen plots are carried out twice, once with each eye fixating.
Classification of Incomitant Deviations
Incomitant deviations can be classified as neurogenic (a problem with the nervous supply), myogenic (a problem with the muscle), or mechanical (where a muscle is mechanically restricted).
Three cranial nerves control the movements of the extraocular muscles: the fourth nerve (IV; trochlear) controlling the superior oblique muscle, the sixth nerve (VI; abducens) controlling the lateral rectus, and the third nerve (III; oculomotor) controlling the other extraocular muscles. These nerves have nuclei, and neurogenic deviations can be supranuclear, nuclear, or infranuclear depending on whether the lesion occurs above, at, or below the level of the relevant nucleus. Nuclear palsies are rarely isolated due to the extensive size of the causative lesion in most cases, so the clinical findings are complicated by involvement of adjacent supranuclear eye movement control centres ( Ansons & Davis, 2001 ).
Fig. 17.5 illustrates the ocular cranial nerve pathways. The long pathway of the fourth and sixth nerves makes them prone to damage. The fourth nerve is particularly slender. Where the sixth nerve bends at the petrous temporal bone it is particularly prone to damage from compressive lesions from above following raised intracranial pressure, or from below such as following otitis media infection.

In myogenic palsies the primary problem affects the muscle itself rather than influencing its nerve supply or mechanically constricting the muscle. The most common example is myasthenia gravis.
Incomitancies that result from mechanical restriction are caused by elements within the orbit which either interfere with muscle contraction or otherwise prevent free movement of the globe. The restriction may be direct (e.g., tight or shortened muscle or tendon) or indirect (e.g., large retinal explant following retinal detachment surgery).
Incomitant deviations can also be classified as congenital or acquired. The main causes of acquired incomitant deviations are summarised in Table 17.2 . Myasthenia gravis is not strictly neurological in origin: it results from an anomaly at the neuromuscular junction.
Vascular | Neurological | Other |
---|---|---|
Diabetes | Tumour | Trauma |
Vascular hypertension | Multiple sclerosis | Thyroid eye disease |
Stroke | Migraine | Toxic |
Aneurysm | (Myasthenia gravis) | Iatrogenic |
Giant cell arteritis | Idiopathic |
Acquired neurogenic palsies can be a sign of life-threatening pathology or of trauma. Nearly all myogenic palsies are acquired. Mechanical incomitancies can be congenital (e.g., Duane syndrome) or acquired (e.g., blow-out fracture). Depending on the cause, acquired incomitancies may undergo spontaneous partial or complete recovery. So, surgical intervention is often postponed until consecutive Hess plots have been stable for at least 6 months.
Incomitant Deviations as a Cause of Diplopia
Moorfields Eye Hospital carried out a retrospective review of 171 patients presenting with diplopia ( Comer, Dawson, Plant, Acheson, & Lee, 2006 ). One in five patients originated from optometrists. Monocular diplopia accounted for 11.5%, caused by factors relating to refractive error, bifocal segment, ectropion, entropion, corneal lesions, cataract, retinal lesions, and migraine (rarely, monocular diplopia or polyopia can result from central nervous system disease; Miller, 2011 ). Of the 146 patients with binocular diplopia, cranial nerve palsies accounted for 67% and the results are summarised in Table 17.3 . Overall, 78.5% of cases had resolved after 12 months, with the best prognosis for incomitancies of a vascular origin.
Condition | % | Aetiology |
---|---|---|
Isolated VI nerve palsy | 31 |
|
Isolated IV nerve palsy | 25 |
|
Trauma | 11 | Occasionally: soft tissue trauma, orbital floor fracture |
Myogenic | 11 |
|
Isolated III nerve palsy | 8 |
|
Decompensating heterophoria | 3 | Rarely: esophoria, exophoria, hyperphoria |
Orbital | 2 | Rarely: metastases, cellulitis, dacryoadenitis |
Combined IV & VI nerve palsy | 1 | Rarely: herpes zoster, migraine |
Bilateral VI nerve palsy | 1 | Rarely: raised intracranial pressure |
A similar study from Scotland found 54% had an isolated nerve palsy (III, IV, or VI), 11% a mechanical cause, 10% dysfunction of higher control, 8% decompensation of existing phoria, 7% idiopathic, 5% monocular diplopia, and 5% another diagnosis. Fewer than 5% had serious underlying pathology that required immediate management.
A retrospective review of 300 hospital cases of vertical diplopia revealed the most common causes were congenital fourth nerve palsy (23%), thyroid eye disease (21%), and acquired fourth nerve palsy (9%) ( Tamhankar, Kim, Ying, & Volpe, 2011 ). Other causes included ocular surgery, orbital fracture, neurosurgery, childhood strabismus, skew deviation, third nerve palsy, myasthenia gravis, and decompensated hyperphoria. The authors concluded that in most cases of hypertropia, the aetiology can be ascertained by history and eye examination alone. Two-thirds of patients did not have an observable limitation of eye movements.
Several systemic medications, particularly for epilepsy, can cause diplopia ( Table 17.4 ), often from neurogenic palsies ( Alves, Miranda, Narciso, Mieiro, & Fonseca, 2015 ). When a patient reports recent onset diplopia, it is advisable to ask about any new medication that was started around the time of the onset of the diplopia.
Likelihood of diplopia | Drug | Main uses of drug |
---|---|---|
Very common (≥1 in 10) |
|
|
Common (between 1 in 10 and 1 in 100) |
|
|
Uncommon (between 1 in 100 and 1 in 1000) |
|
|
Rare (between 1 in 1000 and 1 in 10,000) |
|
|
Investigation
Most of the remainder of this chapter is concerned with the detection of incomitant deviations and the interpretation of their significance. Most patients in need of urgent medical attention have symptoms that lead them to consult medical practitioners in the first instance. It is, however, important to be able to detect incomitancy, as it does not respond well to eye exercises and occasionally cases of active pathology present themselves to community optometrists. The first indication of incomitancy may emerge during a routine eye examination, and additional tests may be required to confirm the diagnosis. The sections of the routine and appropriate additional tests are reviewed later.
History and Symptoms
Incomitant deviations due to recent injury or to active pathology nearly always have a sudden and dramatic onset of symptoms, sudden diplopia being the most usual. In long-standing deviations, the symptoms are seldom so disturbing to the patient, and of course they are usually reported as having been present for as long as the deviation. The following symptoms may be present:
- 1.
Diplopia is often present in incomitancy but may not be present in heterophoric incomitancy nor in long-standing strabismic incomitancy. The patient may be able to recognise the variation in the degree of doubling in different direction of gaze. There is usually a vertical element in the diplopia. In long-standing cases, it may be intermittent due to sensory adaptations. Two-thirds of patients who acquire strabismus following brain damage (usually stroke or trauma) do not experience diplopia ( Fowler et al., 1996 ).
- 2.
Asthenopia may be present ( Smith, 1979 ).
- 3.
Blurred vision may be present if the condition involves the third cranial nerve which also serves the ciliary muscle. For the same reason, the pupil reflexes may be abnormal. Some patients describe small degrees of diplopia as blur.
- 4.
Dizziness or vertigo may accompany incomitant heterophoria ( Rabbetts, 2007 ). Normally, a change in the pattern of innervation to the extraocular muscles is associated with a particular movement of the retinal image. Incomitancy results in an imbalance between innervation and retinal image movement and this can make the patient’s surroundings appear to move. If the paresis is mild, there may be an incomitant heterophoria rather than strabismus with diplopia. Hence, the symptoms of vertigo and dizziness may be reported.
- 5.
Other symptoms due to the disease causing the incomitant deviation may be present; for example, headache in intracranial conditions, neoplasms, vascular disturbances, etc. The diseases most likely to be associated with incomitant deviations are dealt with in a later section of this chapter, where their symptoms are also summarised.
- 6.
General health deterioration may also occur in accompanying metabolic disorders: loss of weight, changed appetite, general fatigue, loss of muscular ability, muscular tremor, breathlessness, etc.
- 7.
Injury to the head or orbital regions may be reported, and this could cause damage to the muscular apparatus, intracranial bruising, and damage or pressure from haemorrhage. This can be recent or be the explanation of a long-standing incomitant deviation. In some cases, the patient may not have thought the injury serious enough to seek medical advice at the time. Injury during birth sometimes causes lateral rectus palsy or superior oblique palsy. An operation for a previous strabismus can sometimes cause a degree of incomitancy.
- 8.
Previous comitant strabismus can result in an acquired incomitancy (see later).
Anomalous Head Postures and Facial Asymmetry
Acquired ocular torticollis is a type of anomalous head posture (AHP) that occurs in some patients with incomitant deviations, most commonly superior oblique paresis ( Nucci, Kushner, Serafino, & Orzalesi, 2005 ). In nearly every case, the purpose of the AHP is to reduce the effect of the incomitancy, so the AHP turns the head towards the field of action of the affected muscle. Generally speaking, if the underacting muscle is horizontally acting, then there will be a head turn; if it is a torsional muscle (obliques), then there will be a head tilt; and if it is a vertically acting muscle, there will be an elevation or depression of the head. The most commonly encountered AHPs are for lateral rectus palsies (right lateral rectus palsy: head turn to right), superior oblique pareses (right superior oblique paresis: top of head tilted to patient’s left), Duane syndrome (head turn), pattern deviations (elevation or depression), and Brown syndrome (may be turn, elevation, and tilt). Sometimes, a head tilt is adopted in a vertical incomitancy to level the diplopic images and so aid fusion. Common AHPs are detailed in Appendix 8 . Normal amounts of head tilt (up to 30 degrees) do not affect reading speed ( Firth, Machin, & Watkins, 2007 ).
The typical AHPs outlined earlier apply to the usual situation, when the purpose of the AHP is to reduce the effect of the incomitancy. Very rarely, an AHP may be adopted for the opposite reason: to exaggerate the effect of an incomitancy (von Noorden, 1996 ). For these rare cases, this can have two advantages: it can cause the deviation to break down and hence eliminate a symptomatic heterophoria, or it can cause diplopic images to move further apart making them easier to ignore. These cases are easy to detect because the patient will be strabismic when they view a straight-ahead object using their normal AHP. Another complication is that a compensatory head posture may be provoked not so much by the primary paralysis as by the modifications of other muscles induced by the paralysis (secondary sequelae).
An AHP that has been present for many years is frequently associated with facial asymmetry and this is found in more than 75% of patients with congenital palsy, typically from a congenital superior oblique palsy. The shallower side of the face is always on the side of the head tilt ( Plager, 1999 ). The presence of a facial asymmetry is such a strong sign of an early onset that it may preclude the need for neurological investigation ( Plager, 1999 ).
Because the usual purpose of an AHP is to preserve or enhance binocularity, the presence of an AHP suggests the patient has had binocularity at least at some time in the past. This improves the prognosis for treating sensory factors.
Other visual causes of AHPs are a visual field loss and to move the visual axes into the null zone in congenital nystagmus ( Chapter 18 ). However, most (60%) cases of torticollis in children are nonvisual, mainly orthopaedic ( Nucci et al., 2005 ), but also sometimes from unilateral deafness, shyness, or just habit.
External Examination of the Eyes
General inspection may show an obvious strabismus. Scars or asymmetry of the orbital region may indicate previous injury. Some eye-signs of systemic disease may be seen in conditions which are sometimes accompanied by strabismus: exophthalmos, ciliary hyperaemia, ptosis, etc.
Eyelid Signs
Eyelid abnormalities may sometimes be useful in indicating the presence of an incomitant deviation. The width of the palpebral fissure should be noted:
- 1.
In the primary position when the right and left lid openings are compared. The width may be judged by the amount of the limbus visible through the lid openings. An abnormally wide fissure (Dalrymple’s sign of thyrotoxicosis) may be accompanied by hypophoria or hypotropia which increases on elevation of the eyes. Ptosis and diplopia which are both worse at the end of the day can be an early sign of myasthenia gravis. Ptosis can also be a sign of third nerve palsy. A hypotropic position of one eye may show a ‘pseudoptosis’; the lid is slightly lower as the eye is turned down.
- 2.
During the motility test, a lag of the lids on downward gaze (von Graefe’s sign) may be present in thyrotoxicosis. A change in lid fissure, when looking left or right, usually accompanies Duane retraction syndrome (p. 279).
Ophthalmoscopy and Fundus Photography
The internal examination of the eyes may also provide further evidence of pathology such as those present in vascular conditions or metabolic disease. Indirect ophthalmoscopy and fundus photography can be used to provide an objective measure of ocular torsion in which the relative positions of the fovea and optic disc are noted. Normally, the fovea is 0.3 disc diameters below a horizontal line extending through the geometric centre of the optic disc. A variation of more than 0.25 disc diameters between the two eyes indicates cyclodeviation (von Noorden, 1996 ). Visual field analysis can also be used in a similar way. A problem with these approaches is their sensitivity to improper head position ( Phillips and Hunter, 1999 ).
Ocular Motility Test
The examination of ocular motility is an essential part of the detection of incomitancy. The ocular motility test allows a subjective, and an objective, check that:
- 1.
both eyes move smoothly and follow the target;
- 2.
there is a corresponding lid movement accompanying the vertical eye movements; and
- 3.
there is no underaction or overaction of the movement of one eye in any direction of gaze.
The details of procedure for investigating the motility in routine examination are given in Chapter 2 . This chapter is mainly concerned with determining the significance of any anomaly and with any additional tests which may give further information. The site of a muscle palsy can be determined from an understanding of the actions of the extraocular muscles, as described at the beginning of this chapter.
In the motility test, the patient is asked to keep the head still and to follow, with the eyes, a pen torch as it is moved into the different parts of the visual motor field. The patient is asked to report any diplopia, although patients with an incomitancy may not report any diplopia, owing to sensory adaptations. Often, the most useful information that the motility test gives relates to the practitioner’s observation of the eye movements.
The fixation light is moved up and down in the median plane, so that lid movements and vertical eye movements (e.g., detecting gaze palsies) can be observed. In the method recommended by Boylan and Clement (1987) , the light is then moved across the field at three levels: at the top, at eye level, and in the lower part of the motor field. This is done with the patient following the light with both eyes, so one eye’s position can be judged relative to the other. A failure of one eye to follow the light in the top of the field indicates an anomaly of one of the elevators. To the patient’s right and top, the affected muscle is likely to be either the right superior rectus or the left inferior oblique. Failure of one eye to turn to the right or to the left at eye level is likely to show an anomaly of either medial recti muscles or either lateral recti, and failure in the lower field shows a problem with one of the depressor muscles ( Fig. 17.6 ). In these directions of gaze, each muscle has little or no secondary actions. Alternatively, some authors recommend that a ‘star’ technique is used where the pen torch is moved in the vertical, horizontal (at eye level), and four oblique positions ( Mallett, 1988a ).

It should be noted that Fig. 17.6 does not show muscle actions but shows the approximate directions in which the muscles have their greatest ability to move the eyes, excluding torsional movements. These diagnostic positions of gaze are very different from the actions of the extraocular muscles in the primary position ( Table 17.1 ). This is because the actions of each muscle will change as the eye moves. The cardinal diagnostic positions of gaze are important in interpretation of ocular motility results, but knowledge of the actions of the muscles in the primary position is required to interpret the results of the cover test carried out in the primary position.
The motility test is the only objective method available for standard clinical investigation of muscle paresis. Small deviations of one eye when it is in a tertiary position are not easy to detect. Observation of the corneal reflection of the fixation light will help, as will the symmetry of the lid and eye positions comparing dextroversion with laevoversion. Fortunately, from the detection point of view, underaction of a muscle is usually accompanied by an overaction in the paired synergic muscle which exaggerates the deviation.
Patients with active pathology usually have diplopia and this helps detection. Very small degrees of diplopia may be detected subjectively, which makes diagnosis more certain. During the motility test, therefore, the patient is asked to report any doubling and how this varies in different parts of the field. A diplopic image due to a paretic muscle is displaced in the same direction as the rotation which contraction of that muscle normally produces. The eye which sees the outermost diplopic image when the eyes look in the direction of maximum separation of the images is therefore the eye with the paretic muscle, and this eye can be identified by covering one eye. Subjective analysis of ocular motility can be assisted by using red and green diplopia goggles. A red goggle is worn before the right eye and a green one before the left. However, goggles prevent the eyes being observed.
It is useful to quantify the degree to which a deviation is incomitant, so any change can be monitored to assess if the condition is getting better or worse. This can be done by several methods and Appendix 8 is a worksheet for recording these results. This includes three variations of the motility test, including cover testing in peripheral gaze as described next. Incomitant deviations can be difficult to diagnose, and in incomitant cases, three versions of the motility test are often easiest to interpret if they are carried out separately ( Chapter 2 ).
Cover Test
In the primary position, the cover test can be used to compare the size of the deviation when the patient is fixating with either eye. The deviation is larger (secondary deviation) when the patient is fixating with their paretic eye than when they are fixating with the nonparetic eye (p. 250).
The cover test also can be used to measure the deviation in different positions of gaze during the motility test. The cover/uncover test, alternating cover test, or prism cover test can be used in peripheral gaze to provide further objective information on the motility test results. The alternating cover test is often easiest to interpret, but with just two to three alternate covers in each position of gaze to avoid causing greater dissociation than is necessary.
The Maddox ROD or Hand Frame
Primary and Secondary Deviations
The difference between primary and secondary deviations was explained earlier in this chapter. This phenomenon can be investigated ( Appendix 8 ) with a Maddox rod test in the primary position, with the rod first in front of the strabismic eye (measuring the primary deviation) and then in front of the nonstrabismic eye (measuring the secondary deviation). If the two readings are different, this suggests an incomitancy ( Borish, 1975 ). Unfortunately, the author has been unable to find any norms for determining what represents a significant difference between the two eyes with this test.
Deviation in Different Positions of Gaze
The Maddox rod can be used to measure the horizontal and vertical deviations in different directions of gaze ( Appendix 8 ). Using a pen torch for fixation, the patient’s head is kept still whilst measurements are taken in different parts of the field. It is important that the light is at a fixed distance and is moved to definite peripheral positions, so the test is repeatable. It is suggested that it is held at 50 cm from the eyes, and at the corners of a square formation in front of the patient, of 50 cm dimension.
Double Maddox Rod Test and Similar Approaches
In the double Maddox rod test, two Maddox rod lenses are placed, one in front of each eye, to measure cyclodeviation ( Phillips & Hunter, 1999 ). The method is described on p. 133 and illustrated in Fig. 9.1 . A significant cyclodeviation suggests the involvement of an oblique muscle.
The tilt of the retinal image is opposite to the tilt of the line as seen by the observer. So, if the patient reports that the line seen by their right eye is tilted with the outer end up, they have right ex-cyclodeviation, suggesting underaction of the right superior oblique. In summary, the line is perceived to be tilted in the direction in which the underacting muscle would rotate the eye .
Paresis of the superior oblique muscle can be very difficult to detect on motility testing ( Brazis, 1993 ) and Simons, Arnoldi, and Brown (1994) stated that the double Maddox rod test is the standard test for investigating a superior oblique paresis. Theoretically, the test can demonstrate which eye(s) manifest the paresis. However, von Noorden (1996) cautioned that an ex-cyclodeviation in superior oblique paresis may occur in the nonparetic eye in patients who habitually fixate with the paretic eye owing to a monocular sensorial adaptation to the cyclodeviation. Sensory adaptations (harmonious anomalous retinal correspondence (HARC) or sensory cyclofusion) and motor cyclofusion ( Phillips & Hunter, 1999 ) may explain why some patients with congenital superior oblique palsies have minimal subjective torsion with the double Maddox rod test ( Plager, 1999 ).
Simons et al. (1994) stressed that both eyes should view through the same colour Maddox rod, in which case the paretic eye is correctly diagnosed in 94% of cases. A comparison of five methods of measuring ocular torsional movements found that the double Maddox rod was reliable when used in the primary position ( Capdepon, Klainguti, Strickler, & van Melle, 1994 ), although Kraft, O’Reilly, Quigley, Allan, and Eustis (1993) found that the test was best at discriminating superior oblique palsies (normal/single/double pareses) in down-gaze.
A pair of Bagolini lenses can be used, with axes parallel, in an analogous way to the double Maddox rod test (von Noorden, 1996 ). When Bagolini lenses are used, the eyes are not dissociated, so the test is unlikely to work in strabismic cases where there is suppression and will be confounded in HARC. The result with this test may be similar to an assessment of the cyclodeviation with the Mallett fixation disparity test, which might be expected to have the same shortcomings with strabismic patients.
The Maddox wing test can be used to measure cyclodeviations and is straightforward to interpret. Very large cyclodeviations cannot be measured in this way. It is important to keep the patient’s head and the instrument level.
Rabbetts (1972) described a prototype instrument, the cyclophorometer, that used polarised filters to dissociate the eyes. A similar principle is used in the commercially available torsionometer, in which the patient views a red and a green line on card through red/green goggles ( Georgievski & Kowal, 1996 ). One of the lines is rotated until they are parallel, and the degree of torsion is recorded from the required rotation of the line. The test is less dissociating than the double Maddox rod test, but probably more dissociating than the Bagolini lens test. Even for patients with diplopia, where the eyes are effectively already dissociated, there is some variation (more than 5 degrees in 10% of patients) between different methods of measuring cyclodeviations (double Maddox rod, Maddox wing, synoptophore, torsionometer), although no particular test was responsible for the variation ( Georgievski & Kowal, 1996 ).
Screen Tests
Alternatives to the Hess screen, the Foster and Lancaster screens, employ similar principles to those described for the Hess screen. These methods provide the most thorough way of recording the degree of incomitancy and other information which will help assess progress of the condition. During all these tests, it is essential that the patient’s head does not move.
Screen tests are essentially dissociation tests that are carried out in different positions of gaze. The deviation is plotted in space, and chart plots can give a precise measure of the deviation in different positions of gaze. Another important feature of the test is that it is carried out first with one eye fixating and then the plot is repeated, but with the other eye fixating. This is to differentiate between the primary and secondary deviation (p. 250).
Hess Screen
Modern Hess screens are grey in colour, so that the light from two projector torches can be seen on the screen. The patient sits at a distance of 50 cm. The screen is divided into ‘squares’ representing 5-degree rotations of the eyes. As the screen is flat, tangential to the line of sight, the squares are distorted into a pincushion pattern. The practitioner holds the red torch and the patient wears red/green diplopia goggles. On the screen, a red bar image from this torch can be seen only by the eye with the red goggle before it. Thus, when the patient is asked to look at the red bar image on the screen, the eye will be positioned so the red image falls on the fovea of that eye. The patient holds a green torch and is asked to shine its bar image on the screen so that it appears to cross the red bar. This subjective cross will be formed when the green bar of light is in such a position that its image falls on the fovea, as the fovea in each eye are corresponding points and have the same visual direction. Therefore, the positions of the bar images on the screen mark the points of intersection of the visual axes with the screen. The degree of any deviation can be estimated from the 5-degree marking. The relative positions are plotted in nine positions of gaze. Then, the goggles are changed round so that the red goggle is in front of the other eye, and the plot is repeated with this eye fixating and the first one deviated. A copy of the plot is made on a paper chart with each eye fixating in turn.
Some versions are internally illuminated, so that an LED is illuminated in the appropriate position ( Fig. 17.7 ).

A computerised version of the Hess screen is available ( Fig. 17.7 and Appendix 12 ) which allows plots ( Fig. 17.10 ) to be obtained on standard desktop or laptop computers. Head position needs to be carefully monitored, and too close a working distance might reduce the ability of the test to detect subtle lateral rectus palsies. The absence of the peripheral points that are present on the standard Hess chart might be a disadvantage with rare cases of subtle mechanical limitations, although in the author’s experience this has not been a significant problem. A prototype of the test was described by Thomson, Desai, and Russell-Eggitt (1990) and a result recorded with the test is illustrated in Fig. 17.9 .
Lees Screen
The Lees screen is a pair of Hess screens mounted at right angles, the markings showing only when internally illuminated. A pair of mirrors mounted back to back bisects the angle between the screens ( Fig. 17.8 ). The patient initially faces the unilluminated screen and views the illuminated right screen with his or her right eye by reflection in the mirror. The patient is thus fixating with the right eye. The examiner uses a pointer to indicate a test position on the illuminated right screen and the patient uses a pointer to indicate the position to which the right pointer is projected on the left screen. The practitioner then presses a foot switch to briefly illuminate the left screen and plots the result relative to the correct position on a record chart ( Fig. 17.8 ). This procedure is repeated for various test positions, as with the Hess screen. The standard pointers have circular targets, but they can be modified to bar targets to aid the investigation of torsional palsies.

Interpretation of Hess or Lees Screen Plots
It is important with these techniques that the results are plotted correctly, with the fixating eye recorded as such. With the Hess screen, the fixating eye sees the image projected by the examiner. With the Lees screen, the fixating eye sees the image which is constantly illuminated.
General Points (Worksheet in Appendix 8 )
- 1.
All counting of squares must be from the centre of the plot (result recorded), not from the centre of the chart ( Fig. 17.9 ).
Fig. 17.9 Example plots from the PC Hess screen test. (A) right superior oblique muscle paresis; (B) mild left lateral rectus underaction; (C) recent paresis of left lateral rectus (caused by vascular hypertension); (D) same as (C) but partially resolved 1 month later (see also Appendix 13 and Digital Resource). - 2.
The test is, fundamentally, a dissociation test in different positions of gaze. When the central point is plotted, this is equivalent to a dissociation test in the primary position and the deviation should be revealed. If the deviation varies when different eyes are fixating, this suggests incomitancy.
- 3.
The test is based on foveal projection, so the position of the plots indicates the position of the eyes. For example, if the left eye’s plot is higher than the right, then there is left hypertropia.
- 4.
It follows from (3) that the smallest plot will be from the eye with the underacting muscle(s) ( Fig. 17.9 ). If it is difficult to tell which is the smaller plot, concentrate on a comparison of the height of the plot in vertical incomitancies and on the width in horizontal incomitancies.
- 5.
The paretic muscle(s) can be found by looking for the smallest distance from the centre of the plot.
- 6.
There should be an overaction of the contralateral synergist to the palsied muscle(s), and this will be very marked in a recent onset incomitancy. This overaction occurs because of Hering’s law of equal innervation.
- 7.
In a long-standing incomitancy, secondary sequelae usually will be apparent, as described later.
- 8.
Sloping fields are indicative of an A- or V-pattern, not a cyclodeviation (although this may be present as well).
- 9.
In mechanical incomitancies, secondary sequelae (see later) are not likely to be present and the deviation in the primary position does not reflect the extent of the defect.
Localisation Disturbances
The localisation of objects in space is determined visually by a combination of two mechanisms: retinal localisation, and the motor system’s directional mechanism. The position of the image on the retina determines the direction in which it is perceived: its visual localisation. Because the eyes and head move, the brain must also take these movements into account in localising an object with respect to the egocentre. Eccentric fixation can cause a disturbance of the retinal system when the fixation point moves away from the centre of localisation at the fovea: past pointing occurs ( Chapter 13 ).
The motor localisation system uses two types of information to register eye position. One of these is feedback from the extraocular muscles themselves (inflow), which is called muscle proprioreception. The other is information which is copied within the brain from the centres that control movement to those that monitor eye position ( efferent copy : outflow). Proprioreception is a back-up to efferent copy ( Bridgeman & Stark, 1991 ). If the eyes do not move correctly in response to the nerve impulses sent to the muscles, as in paretic strabismus, then the motor localisation system will be disturbed.
The past pointing test may be used to demonstrate these motor disturbances. The test is applied monocularly to each eye in turn, as described in Chapter 13 . Past pointing will be demonstrated in the eye having the affected muscle and in the field of action of this muscle. The degree of past pointing will increase as the eye turns further into the direction of its action and will not occur in the opposite direction of gaze. This test can be made more effective by holding a card horizontally (for horizontal deviations) at the level of the patient’s chin, so that it occludes the patient’s pointing hand from view while the target appears above the card. Past pointing only occurs with paresis of recent onset and lessens as the patient adapts to the deviation. Past pointing can also occur in eccentric fixation (p. 184), where it is typically of a lesser degree than in incomitant deviations of recent onset.
Testing the Vestibular System
In supranuclear lesions the vestibular ocular reflex (VOR) will be maintained, but in those caused by infranuclear lesions it will not. The VOR is phylogenetically the oldest slow eye movement system and, because it does not require visual feedback, it has a short latency. Horizontal VOR is well-developed at birth; the vertical VOR develops a little later.
The VOR can be tested to investigate whether a gaze palsy is supranuclear or infranuclear, and to investigate poor abduction in an infant. The two most common ways of testing the VOR are as follows. In the Doll’s head test, the patient is asked to fixate an object while the examiner rapidly rotates the patient’s head, first in the horizontal and then in the vertical plane. A normally functioning VOR will result in eye movements equal and opposite to head movements. In the spinning baby test, the infant is held upright by the practitioner sitting on a rotating chair. The practitioner rotates themselves and the baby through 180 degrees or 360 degrees, observing the patient’s eyes. The eyes make a conjugate movement in the direction opposite (compensatory) to that of the body rotation and then, after about 30 degrees, they make a fast movement to the primary position, the cycle repeating. Once the rotation is stopped there may be some post-rotational nystagmus, but only for a few seconds in a sighted infant. An older infant may maintain fixation on the examiner’s face, in which case the test can only be done in the dark with eye movement recording equipment.
Algorithms for Aiding the Diagnosis of Vertical Muscle Pareses
The diagnosis of the paretic muscle(s) is fairly straightforward in horizontal deviations, but is more complicated in cyclovertical deviations ( Spector, 1993 ). Three algorithms (e.g., three-steps tests) have been developed to help practitioners detect the paretic muscle(s) in cyclovertical deviations, and these will now be described. The principles behind the various tests in these methods can be understood from the earlier sections of this chapter.
The Lindblom 70-cm Rod Method
A very simple method of qualitatively investigating cyclovertical incomitancies is to use a 70-cm rod held horizontally, 1 m in front of the patient ( Lindblom, Westheimer, & Hoyt, 1997 ). If a 70-cm rod cannot be found, a 50-cm ruler will suffice ( Table 17.5 ). The patient is asked to describe whether the rod is single or double and, if double, whether tilted (indicating faulty oblique muscle(s)) or parallel (indicating faulty vertical recti muscle(s)). If tilted, the patient’s perception typically resembles an arrow (e.g., ∠) which points towards the side of the eye with the paretic muscle. The muscle can be further identified by investigating the effect of up- and down-gaze on the patient’s perception. If the patient reports a shape resembling an ×, it suggests there may be a double oblique paresis, usually both superior obliques. This approach is summarised in Table 17.5 , and is included in the worksheet in Appendix 8 .

Full access? Get Clinical Tree
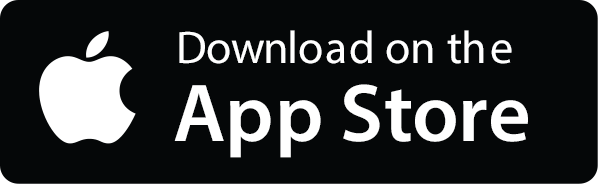
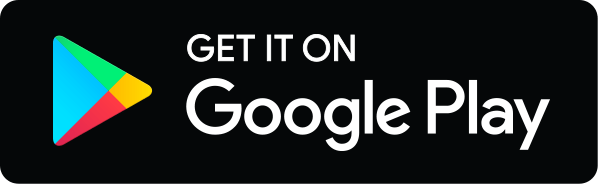