Abstract
The aim of this in-vitro experimental study was to design a novel drug delivery system that may permit controlled release of N-acetylcysteine (NAC) following intratympanic administration. The system was composed of two different solutions that attained a hydrogel form within seconds after getting into contact with each other. The authors performed swelling, pH and temperature tests and analysis of controlled release of NAC from this novel controlled release system. For the structure and porosity analysis of the hydrogel, an environmental scanning electron microscope (SEM) was used. The diameter of designed hydrogel showed an increase when pH was increased. In addition, in comparison to acidic values, the pore diameter of the hydrogel increased significantly especially in physiological level. The increase in the pore diameter was also directly proportional to the increase in temperature. Spectrophotometric analysis showed that the amount of NAC released into the medium was statistically significant ( p = 0.038, t = − 2.18, 95% CI; DF: 27). SEM analysis of the samples revealed a smooth surface topography and numerous porous structures. The authors are of the opinion that the designed hydrogel may be used as an alternative method for intratympanic delivery of NAC for otoprotective purposes. The disadvantages of intratympanic injection of the drug in its liquid form, including leakage through eustachian tube, restraining the patient in an uncomfortable position, necessity for repetitive injections and dose dependent inflammation of the middle ear epithelium, may also be avoided. Further in vivo studies should be conducted to assess its tolerability and effectivity.
1
Introduction
Many antineoplastic agents, including cisplatin, were reported to cause inner ear damage characterized by irreversible and progressive bilateral high frequency hearing loss, and tinnitus . Cisplatin was found to induce atrophy of stria vascularis, collapse in Reissner’s membrane and damage in the outer hair cells and supporting cells of the organ of Corti . The ototoxicity of the drug is associated with its ability to exhaust the endogenous antioxidant system and trigger the apoptotic cascade via monohydrated cisplatin complex .
So far, a number of otoprotective pharmaceutics were administered via either systemic or intratympanic (IT) route in an attempt to prevent cisplatin induced ototoxicity . A number of sulfur containing antioxidants, including methionine, sodium thiosulfate, and N-acetyl-cysteine (NAC), are known to have high affinity to platinum species . Among these, NAC is a well known antioxidant and was reported to protect the inner ear from the toxic effects of cisplatin by inactivating reactive oxygen species and chelating both cisplatin and its monohydrated complex . However, many otoprotective drugs, including NAC, were found to fail to attain adequate otoprotective concentrations in the inner ear following systemic administration . Furthermore, systemic administration of such pharmaceutics was found to interfere with antitumor activity of cisplatin . For these reasons, administration of the otoprotective drugs into the middle ear cavity, where it may diffuse into the cochlear fluids through the round window membrane, became the preferred method for the prevention of cisplatin induced toxicity .
Although, IT injection of NAC was suggested to be a feasible and effective method, many limitations associated with this technique were described in the literature . The most frequently encountered limitation is rapid clearance of the injected solution from the middle ear cavity through the Eustachian tube. To prevent this loss, during IT injection, the patient is usually asked not to move, speak or swallow and stay in an uncomfortable supine position with the head turned 45 degrees to the opposite ear for about 15–30 minutes . Another major limitation of the method was reported to be pain and thickening of the round window membrane secondary to dose-dependent inflammation. IT administration of 20% of NAC solution could not be tolerated by the patients due to the pain associated with the inflammation in the middle ear mucosa. Furthermore, the inflammation was also found to be associated with a decrease in the diffusion rates of NAC into the inner ear fluids due to inflammatory thickening of the round window membrane . The foul sulfur odor of NAC and the necessity of repetitive injections to achieve the desired otoprotective effect (up to eight injections over a period of up to two weeks) were also reported to be the limiting factors that decrease the compliance of the patients during IT injection of NAC . Due to these limitations, following IT administration, NAC may not reach the desired otoprotective concentrations in the inner ear.
The aim of this research was to design a novel method for IT administration of NAC that would avoid the above-mentioned limitations. In this project, NAC was incorporated into a drug delivery system that is in liquid form at room temperature. Following IT injection, the two components of the drug delivery system loaded with NAC would come into contact with each other and polymerize promptly within the middle ear cavity to assume a ‘hydrogel’ form. The release rate of NAC from the hydrogel was analyzed in vitro. The details regarding; (i) the preparation of the hydrogel, (ii) swelling, pH and temperature tests, (iii) analysis of controlled release of NAC, and (iv) ultra-structural analysis of hydrogel were presented. The implications of our findings were also discussed under the scope of the existing literature.
2
Materials and methods
2.1
Materials
Polyvinyl alcohol (PVA) with an average molecular weight (Mv) of 78,400 g/mol was purchased from Vassar Brothers Medical Center (New York). Borax (Na 2 B 4 0 7 *10H 2 0) (di-sodium tetraborate decahydrate) was obtained from Merck (Darmstadt). Chitosan (Mv 140,000-220,000) was procured from Sigma-Aldrich Chemie GmbH (Steinheim) and NAC was supplied from IDOL Ilac Dolum Sanayi ve Tic. A.S. (Istanbul). A UV-spectrophotometer (UV Mini 1240-UV-V15 spectrophotometers, Shimadzu), a magnetic stirrer (Wisestir-wisd-MSH-20A), a pH meter (HANNA, 221 Microprocessor based, Mauritius), a digital caliper (Hangzhou Maxwell Tools Co., Ltd. Zhejiang) and an environmental scanning electron microscope (SEM) (ESEM, Quanta 250 FEG, Fei Company, Hillsboro, OR) were used for the design and analysis of the hydrogel.
2.2
Methods
This research project was conducted in Molecular Biology and Genetics Research Laboratory of Namik Kemal University. The drug delivery system was designed to be composed of two separate solutions in liquid at room temperature. The first solution was composed of PVA, chitosan and NAC and the second solution was composed of saturated borax solution ( Fig. 1 ). This property of the drug delivery system would make intratympanic injection of the solutions easy and possible in a double lumen needle. After coming into contact with each other at the tip of the needle in the middle ear cavity the solutions would become a hydrogel and attain a solid state, entrapping NAC for the controlled release of the drug in consecutive days. Following preparation of the hydrogel, swelling tests as well as degradation tests in different pH and temperature values were conducted. The amount and duration of release of NAC from the hydrogel were also assessed.
The primary objective of this study was to create a drug delivery system that enables controlled release and contains matrices with extremely small, nano-level porous structures. Moreover, the designed hydrogel should also have the characteristics such as biodegradability, volume stability, elasticity and appropriateness for the surgical technique. For this purpose, PVA was mildly cross-linked with microparticles of chitosan, a policathionic biopolymer, (poly-[β-(1,4)-2-amino-2-deoxy-β-D-glucopyranose]). After being passed through a sieve, 200 mg of chitosan was put in a beaker containing 9 ml hydrochloric acid (HCl). The mixture was stirred for 5 minutes and autoclaved at 121 °C for one hour. At the end of this period, the mixture was stirred again for a minute and NAC solution (100 mg/ml) was added into the mixture. Following this, it was stirred until it had a homogenous appearance. Using ultra pure water as a solvent, 9% PVA solution was prepared and placed on the magnetic stirrer. Then, the chitosan-HCl-NAC mixture was poured bit by bit into the PVA solution and hence the first component of the drug delivery system was prepared.
In another beaker, 22% saturated borax solution was prepared. Due to insufficient hydrophilic capacity of PVA, borax was chosen for cross network linking of the functional hydroxyl groups . The unoccupied hydroxyl groups would be able to react with saturated borax solution to achieve a stronger cross linking. 1 ml of PVA-chitosan-HCl-NAC solution and 3 ml of borax solution were taken from the beakers and injected into the well of a microplate simultaneously. The solution polymerized into a hydrogel form within seconds and the resultant hydrogel was transferred to electron microscope laboratory for ultra-structural analysis.
To assess the swelling ability, pH and temperature sensitivity of the hydrogel, as well as the rate of controlled release of NAC, borax solution prepared in a separate beaker was added over the nontoxic and hydrophilic PVA-chitosan-HCl-NAC solution in ratios of 3:1 and 1:1, respectively. The pH value of hydrogel was brought to 7.4 via a pH meter using 0.01 M HCl. Cylindrical pieces of hydrogel with an average diameter of 1 cm and weight of 1 g were formed in accordance with experiment groups. NAC loaded hydrogel samples were vacuum-dried at room temperature (22.4 °C) for 24 hours until they reached constant weighing. Care was given to mold the hydrogel samples gently in order to avoid creation of bubbles within the matrices. For the control group, hydrogel without NAC was prepared using the same protocol and all samples were marked by letter coding. These marked samples were given to the researcher who would perform the analysis and hence the researchers were blinded to the composition of the hydrogel.
In accordance with the theories similar to Flory–Rehner theory, swelling test was also performed to calculate the hydration level of the crosslinks of the swollen hydrogel in this research . To calculate swelling equilibrium of the hydrogel in ultra pure water, the hydrogel samples were divided into 10 mm long pieces with a width of 4.5 mm and a thickness of 1.5 mm. These samples were frozen in − 20 °C for 6 hours and kept in 37 ± 0.5 °C for the next 6 hours. The dried weights of the samples were weighed and recorded. Beakers, each containing 25 ml of ultra pure water, were prepared. All samples were immersed into these beakers. At the end of the first 20 minutes, the sample in the first beaker was taken off, dried with a blotting paper and weighed. At every 20 minutes, the same procedure was applied to the samples in the consecutive beakers and at the end of 240 minutes the last sample in the last beaker was taken off, dried and weighed.
After the swelling equilibrium, the pore diameters of the hydrogels were recorded. Following this, the diameters were measured and the averages of the obtained values were calculated in 25, 60 and 80 °C, respectively. Using different concentrations of HCl and NaOH, pH adjusted ultra pure water was prepared and the changes in the pore diameters of the hydrogel samples in response to different pH values were recorded. Dynamic weight swelling index was calculated with the formula:
Experiments conducted to assess the release rates were repeated at least three times in each occasion. In order to minimize analytical faults, each analysis was performed by the relevant researcher. As the control group, blank hydrogel samples were utilized. The researchers were blinded to the composition of the hydrogel. For assessing the diameters in hydrogel samples, a double walled constant temperature water bath was used. Changes in pore diameters were measured using the digital compass.
Release studies were done in phosphate buffered saline (PBS) (pH = 7.4). Changes in the absorbance values during release were determined using a UV-spectrophotometer within wave lengths of 280–424 (± 2) nm in 37.4 °C . Hydrogel samples with and without NAC were placed in beakers containing PBS solutions (0.1 N NaCl at pH = 7.4). In the following 16 days, the samples were left in the beakers and twice a day the PBS solutions were renewed. At each renewal, 1 ml of liquid was taken from the beakers, put in the Eppendorf tubes and transferred to glass cuvettes of UV-spectrophotometer. Spectrophotometric analyzes of PBS and contents of hydrogel without NAC were conducted and the spectrophotometer was blinded against them. Hence, only the amount of NAC in the medium could be measured by the spectrophotometer.
Absorbance values of molecules released by hydrogel samples with and without NAC within 16 days were spectrophotometrically measured and recorded. Absorbance values of PBS and empty hydrogel samples were subtracted from the absorbance values of NAC loaded hydrogel samples and the remaining values represented the amount of NAC released into the medium from the hydrogel samples. Absorbance-time graphs of NAC loaded hydrogels were drawn. Thereby, rates of controlled release were determined twice in 24 hours for 16 days.
During SEM analysis, FEG ion pumps were used for high vacuum. The images were recorded at a pressure of 100 Pa in ESEM vacuum mode, under magnifications of 1000 ×, 2000 × and 4000 ×, at resolution depths of 104, 207 and 414 μm (HFW), at an operating voltage of 5.00 kV and at a WD of 10.1 mm. Surface topography, characteristic network structure, and porosity of the hydrogel were investigated under environmental SEM. Size analysis and surface area measurements were also performed. Before the analysis, all samples were covered with 2.5% glutaraldehyde-cacodylate buffer solution .
Statistical analysis was performed using a two-tailed, unpaired Student t test. Values of p < 0.05 were considered to be significant. Quantitative data were presented as mean ± S.D.
2
Materials and methods
2.1
Materials
Polyvinyl alcohol (PVA) with an average molecular weight (Mv) of 78,400 g/mol was purchased from Vassar Brothers Medical Center (New York). Borax (Na 2 B 4 0 7 *10H 2 0) (di-sodium tetraborate decahydrate) was obtained from Merck (Darmstadt). Chitosan (Mv 140,000-220,000) was procured from Sigma-Aldrich Chemie GmbH (Steinheim) and NAC was supplied from IDOL Ilac Dolum Sanayi ve Tic. A.S. (Istanbul). A UV-spectrophotometer (UV Mini 1240-UV-V15 spectrophotometers, Shimadzu), a magnetic stirrer (Wisestir-wisd-MSH-20A), a pH meter (HANNA, 221 Microprocessor based, Mauritius), a digital caliper (Hangzhou Maxwell Tools Co., Ltd. Zhejiang) and an environmental scanning electron microscope (SEM) (ESEM, Quanta 250 FEG, Fei Company, Hillsboro, OR) were used for the design and analysis of the hydrogel.
2.2
Methods
This research project was conducted in Molecular Biology and Genetics Research Laboratory of Namik Kemal University. The drug delivery system was designed to be composed of two separate solutions in liquid at room temperature. The first solution was composed of PVA, chitosan and NAC and the second solution was composed of saturated borax solution ( Fig. 1 ). This property of the drug delivery system would make intratympanic injection of the solutions easy and possible in a double lumen needle. After coming into contact with each other at the tip of the needle in the middle ear cavity the solutions would become a hydrogel and attain a solid state, entrapping NAC for the controlled release of the drug in consecutive days. Following preparation of the hydrogel, swelling tests as well as degradation tests in different pH and temperature values were conducted. The amount and duration of release of NAC from the hydrogel were also assessed.
The primary objective of this study was to create a drug delivery system that enables controlled release and contains matrices with extremely small, nano-level porous structures. Moreover, the designed hydrogel should also have the characteristics such as biodegradability, volume stability, elasticity and appropriateness for the surgical technique. For this purpose, PVA was mildly cross-linked with microparticles of chitosan, a policathionic biopolymer, (poly-[β-(1,4)-2-amino-2-deoxy-β-D-glucopyranose]). After being passed through a sieve, 200 mg of chitosan was put in a beaker containing 9 ml hydrochloric acid (HCl). The mixture was stirred for 5 minutes and autoclaved at 121 °C for one hour. At the end of this period, the mixture was stirred again for a minute and NAC solution (100 mg/ml) was added into the mixture. Following this, it was stirred until it had a homogenous appearance. Using ultra pure water as a solvent, 9% PVA solution was prepared and placed on the magnetic stirrer. Then, the chitosan-HCl-NAC mixture was poured bit by bit into the PVA solution and hence the first component of the drug delivery system was prepared.
In another beaker, 22% saturated borax solution was prepared. Due to insufficient hydrophilic capacity of PVA, borax was chosen for cross network linking of the functional hydroxyl groups . The unoccupied hydroxyl groups would be able to react with saturated borax solution to achieve a stronger cross linking. 1 ml of PVA-chitosan-HCl-NAC solution and 3 ml of borax solution were taken from the beakers and injected into the well of a microplate simultaneously. The solution polymerized into a hydrogel form within seconds and the resultant hydrogel was transferred to electron microscope laboratory for ultra-structural analysis.
To assess the swelling ability, pH and temperature sensitivity of the hydrogel, as well as the rate of controlled release of NAC, borax solution prepared in a separate beaker was added over the nontoxic and hydrophilic PVA-chitosan-HCl-NAC solution in ratios of 3:1 and 1:1, respectively. The pH value of hydrogel was brought to 7.4 via a pH meter using 0.01 M HCl. Cylindrical pieces of hydrogel with an average diameter of 1 cm and weight of 1 g were formed in accordance with experiment groups. NAC loaded hydrogel samples were vacuum-dried at room temperature (22.4 °C) for 24 hours until they reached constant weighing. Care was given to mold the hydrogel samples gently in order to avoid creation of bubbles within the matrices. For the control group, hydrogel without NAC was prepared using the same protocol and all samples were marked by letter coding. These marked samples were given to the researcher who would perform the analysis and hence the researchers were blinded to the composition of the hydrogel.
In accordance with the theories similar to Flory–Rehner theory, swelling test was also performed to calculate the hydration level of the crosslinks of the swollen hydrogel in this research . To calculate swelling equilibrium of the hydrogel in ultra pure water, the hydrogel samples were divided into 10 mm long pieces with a width of 4.5 mm and a thickness of 1.5 mm. These samples were frozen in − 20 °C for 6 hours and kept in 37 ± 0.5 °C for the next 6 hours. The dried weights of the samples were weighed and recorded. Beakers, each containing 25 ml of ultra pure water, were prepared. All samples were immersed into these beakers. At the end of the first 20 minutes, the sample in the first beaker was taken off, dried with a blotting paper and weighed. At every 20 minutes, the same procedure was applied to the samples in the consecutive beakers and at the end of 240 minutes the last sample in the last beaker was taken off, dried and weighed.
After the swelling equilibrium, the pore diameters of the hydrogels were recorded. Following this, the diameters were measured and the averages of the obtained values were calculated in 25, 60 and 80 °C, respectively. Using different concentrations of HCl and NaOH, pH adjusted ultra pure water was prepared and the changes in the pore diameters of the hydrogel samples in response to different pH values were recorded. Dynamic weight swelling index was calculated with the formula:
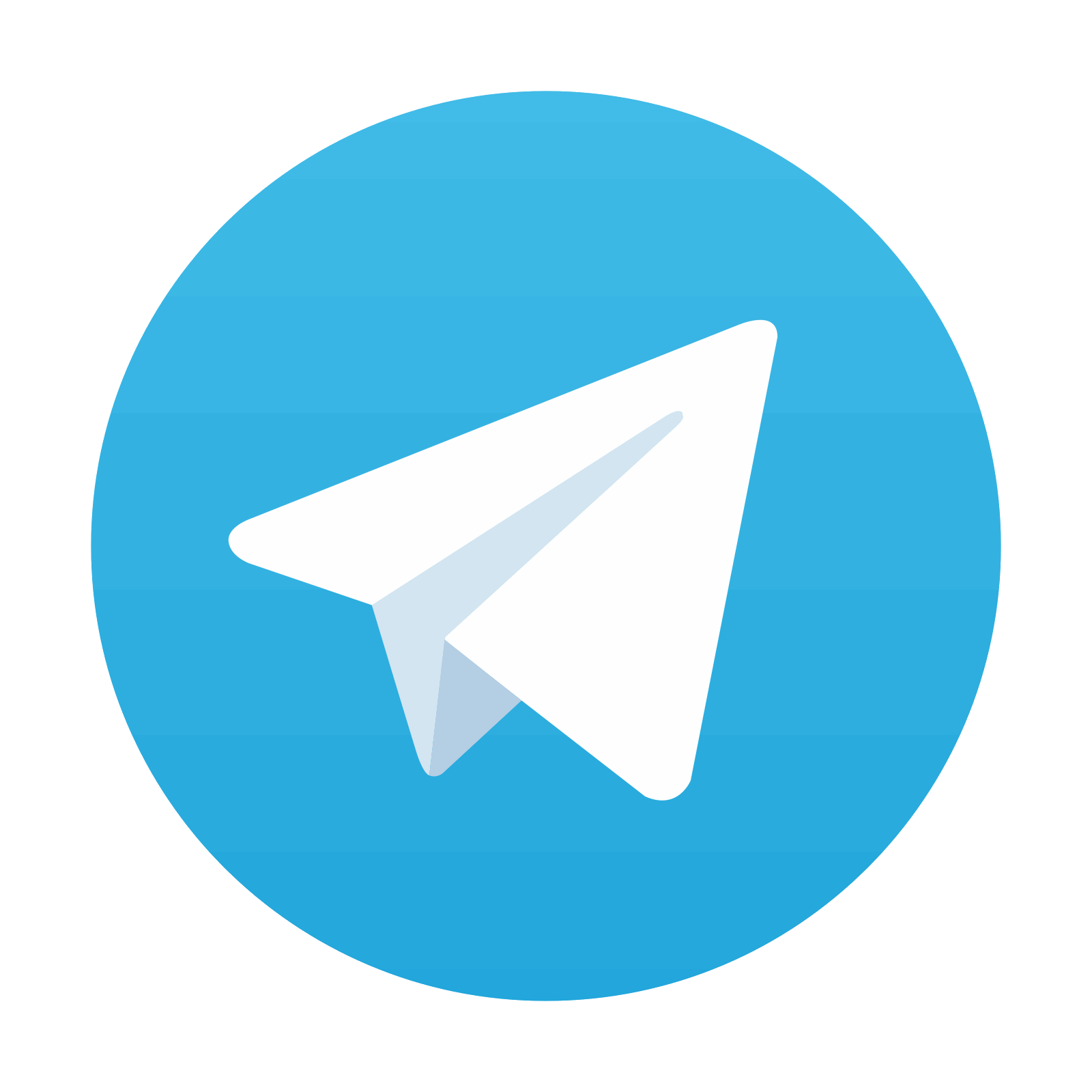
Stay updated, free articles. Join our Telegram channel

Full access? Get Clinical Tree
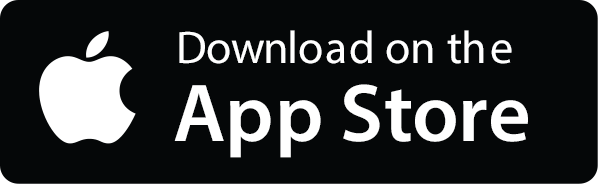
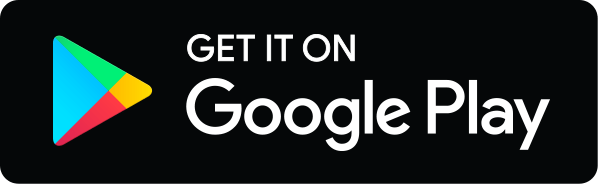