The development of new imaging techniques coupled with new treatment algorithms has created new possibilities in treating temporal bone diseases. This article provides an overview of recent imaging innovations that can be applied to temporal bone diseases. Topics covered include the role of magnetic resonance (MR) diffusion-weighted imaging in cholesteatomas and skull base epidermoids, whole-body molecular imaging in paragangliomas of the jugular foramen, and MR arterial spin labeling perfusion for dural arteriovenous fistulas and arteriovenous malformations.
Key points
- •
High-resolution computed tomography is a fast and dependable method for assessing temporal bone anatomy and planning surgical approach in cases of cholesteatoma.
- •
Diffusion-weighted MRI is likely to decrease the number of second-look surgeries, decreasing patient morbidity and surgical costs.
- •
Contrast-enhanced computed tomography of the skull base, MRI of the skull base and neck, and catheter angiography and embolization in the preoperative period are recommended for evaluation and management of jugular foramen paragangliomas.
- •
Arterial spin labeling (ASL) is an emerging noninvasive MRI procedure that does not require gadolinium-based contrast administration and is a useful diagnostic test for dural arteriovenous fistulas (DAVFs) and small arteriovenous malformations (AVMs) less than 2 cm.
- •
The absence of venous signal on ASL is a helpful predictor of the presence or absence of DAVF or AVM in patients with pulsatile tinnitus and no obvious vascular malformation on routine imaging studies.
ASL | Arterial spin labeling |
AVMs | Arteriovenous malformations |
CBCT | Cone beam computed tomography |
CT | Computed tomography |
CTA | Computed tomography angiography |
DAVFs | Dural arteriovenous fistulas |
DOPA | Dihydroxyphenylalanine |
DOTATATE | Tetraazacyclododecane tetraacetic acid-octreotate |
DSA | Digital subtraction angiography |
DTPA | Diethylenetriaminepentaacetic acid |
DWI | Diffusion-weighted imaging |
EPI | Echo-planar imaging |
18 F-FDG | 18 F-fluorodeoxyglucose |
HRCT | High-resolution computed tomography |
IV | Intravenous |
MIBG | Metaiodobenzylguanidine |
MR | Magnetic resonance |
MRA | Magnetic resonance angiography |
MRV | Magnetic resonance venography |
NET | Neuroendocrine tumors |
PGL-1 | Paraganglioma syndrome 1 |
Introduction
Important advances in diagnostic imaging of the temporal bone have been made in the past decade. The development of new imaging techniques coupled with new treatment algorithms has created new possibilities in treating temporal bone diseases. This article provides an overview of recent imaging innovations that can be applied to temporal bone diseases; it does not provide a comprehensive review of temporal bone disorders and their imaging characteristics, because numerous excellent references already exist in textbooks and review articles.
Topics covered in this article include imaging techniques for evaluation of cholesteatoma and epidermoids, with emphasis on the role of magnetic resonance (MR) diffusion-weighted imaging (DWI); imaging techniques for evaluation of skull base neuroendocrine tumors, including paragangliomas, with emphasis on whole-body molecular imaging; and MR arterial spin labeling (ASL) perfusion for dural arteriovenous fistulas (DAVFs) and arteriovenous malformations (AVMs).
Imaging Techniques for Evaluation of Cholesteatoma and Epidermoids
Since its introduction in the early 1980s, high-resolution computed tomography (HRCT) of the temporal bone has been the gold standard for imaging cholesteatoma. HRCT now represents the preeminent modality for defining the bony anatomy of the temporal bone, as well as pathologic alterations in that anatomy caused by cholesteatoma. Although cholesteatoma is usually readily identified based on history and otoscopic examination, its presence and extent may not always be clear. This considerable unpredictability in size and extent of cholesteatoma can substantially affect surgical approach, expectations, and risk, as can the possible involvement of critical adjacent structures. Despite these strengths of HRCT, in postoperative ears, residual or recurrent cholesteatoma may be in areas concealed from direct inspection, leading to the necessity of second-look surgeries for complete evaluation, because HRCT cannot conclusively distinguish residual or recurrent disease from fluid and granulation tissue, which have similar density.
Because HRCT is limited in its ability to differentiate among soft tissue densities in the temporal bone, the addition of MRI, with its superior soft tissue contrast, has been valuable in the temporal bone. The recent development and refinements of diffusion-weighted MRI (DW-MRI) have contributed significantly in this regard, allowing accurate identification of the presence of small foci of keratin debris that would otherwise be impossible to differentiate from fluid, edematous mucosa, and/or granulation tissue on routine MR sequences and HRCT. In this way, the selective use of HRCT and DW-MRI can provide complementary information that can guide otologic surgeons in the management of cholesteatoma.
The strength of HRCT is its capacity to image bone. A cholesteatoma appears as a soft tissue mass, usually occurring in pneumatized regions of the temporal bone. The normal aeration is lost and the surrounding bone often shows evidence of erosion with smooth or scalloped margins. Adjacent ossicles may be absent, eroded, or demineralized. The scutum is often eroded, revealing the pathway of ingrowth of epithelium from the pars flaccida into the epitympanum ( Fig. 1 ). HRCT is also useful in recognizing the geometry and location of adjacent vital structures. The bony labyrinth, facial nerve canal, tegmen, sigmoid plate, and carotid canal can all be well seen on HRCT. A careful study of the HRCT can also reveal anatomic variations that may affect surgery, such as dehiscence of the facial nerve canal. Similarly, loss of the normal bone overlying any of these structures may give a valuable warning of involvement by cholesteatoma.
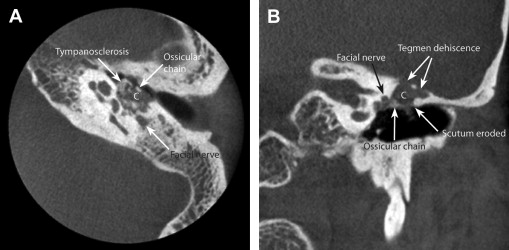
When ossicular or mastoid bony erosion is seen in association with a soft tissue mass, HRCT can distinguish cholesteatoma with specificity between 80% and 90%. In the postoperative period, HRCT has a high negative predictive value when it shows a well-aerated middle ear with no evidence of soft tissue densities. However, HRCT has proved unreliable in differentiating residual or recurrent cholesteatoma from granulation tissue, cholesterol granuloma, mucosal edema, fibrosis, scar tissue, or fluid. However, in patients who have undergone previous tympanomastoidectomy, the relevance of bony erosion is lost because it is difficult or impossible to differentiate surgical changes from pathologic bony destruction caused by cholesteatoma. In this setting, HRCT has a sensitivity of 43%, specificity of 42% to 51%, and a predictive value of 28% in detecting residual or recurrent cholesteatoma.
The introduction of in-office cone beam computed tomography (CBCT) imaging has made imaging for cholesteatoma more convenient and accessible. As a result of their favorable radiation safety profile and compact size, CBCT scanners can be assembled in clinic rooms with often minimal requirements for specialized shielding. In CBCT scanners, the x-ray beam forms a cone-shaped geometry between the imaging source (apex of the cone) and the detector (base of the cone). In contrast, conventional scanners have a fan-beam geometry. The radiation dose of these scans is reported to be 60% of a conventional computed tomography (CT) scanner when evaluating middle ear structures, but middle and inner ear bony structures are seen equally well in CBCT and conventional HRCT scanners. One disadvantage of in-office CBCT is the limited anatomic coverage, which means inner ear or more distal disorders in the mastoid may be missed. An additional CBCT disadvantage is the lack of any soft tissue contrast, and these scanners are typically used only to assess bony anatomy. A general disadvantage of both HRCT and CBCT is their use of ionizing radiation, and hence their intrinsic potential for inducing malignancy. Therefore, clinicians must always be judicious in their use, particularly in children who may be sensitive to cumulative radiation effects.
Introduction
Important advances in diagnostic imaging of the temporal bone have been made in the past decade. The development of new imaging techniques coupled with new treatment algorithms has created new possibilities in treating temporal bone diseases. This article provides an overview of recent imaging innovations that can be applied to temporal bone diseases; it does not provide a comprehensive review of temporal bone disorders and their imaging characteristics, because numerous excellent references already exist in textbooks and review articles.
Topics covered in this article include imaging techniques for evaluation of cholesteatoma and epidermoids, with emphasis on the role of magnetic resonance (MR) diffusion-weighted imaging (DWI); imaging techniques for evaluation of skull base neuroendocrine tumors, including paragangliomas, with emphasis on whole-body molecular imaging; and MR arterial spin labeling (ASL) perfusion for dural arteriovenous fistulas (DAVFs) and arteriovenous malformations (AVMs).
Imaging Techniques for Evaluation of Cholesteatoma and Epidermoids
Since its introduction in the early 1980s, high-resolution computed tomography (HRCT) of the temporal bone has been the gold standard for imaging cholesteatoma. HRCT now represents the preeminent modality for defining the bony anatomy of the temporal bone, as well as pathologic alterations in that anatomy caused by cholesteatoma. Although cholesteatoma is usually readily identified based on history and otoscopic examination, its presence and extent may not always be clear. This considerable unpredictability in size and extent of cholesteatoma can substantially affect surgical approach, expectations, and risk, as can the possible involvement of critical adjacent structures. Despite these strengths of HRCT, in postoperative ears, residual or recurrent cholesteatoma may be in areas concealed from direct inspection, leading to the necessity of second-look surgeries for complete evaluation, because HRCT cannot conclusively distinguish residual or recurrent disease from fluid and granulation tissue, which have similar density.
Because HRCT is limited in its ability to differentiate among soft tissue densities in the temporal bone, the addition of MRI, with its superior soft tissue contrast, has been valuable in the temporal bone. The recent development and refinements of diffusion-weighted MRI (DW-MRI) have contributed significantly in this regard, allowing accurate identification of the presence of small foci of keratin debris that would otherwise be impossible to differentiate from fluid, edematous mucosa, and/or granulation tissue on routine MR sequences and HRCT. In this way, the selective use of HRCT and DW-MRI can provide complementary information that can guide otologic surgeons in the management of cholesteatoma.
The strength of HRCT is its capacity to image bone. A cholesteatoma appears as a soft tissue mass, usually occurring in pneumatized regions of the temporal bone. The normal aeration is lost and the surrounding bone often shows evidence of erosion with smooth or scalloped margins. Adjacent ossicles may be absent, eroded, or demineralized. The scutum is often eroded, revealing the pathway of ingrowth of epithelium from the pars flaccida into the epitympanum ( Fig. 1 ). HRCT is also useful in recognizing the geometry and location of adjacent vital structures. The bony labyrinth, facial nerve canal, tegmen, sigmoid plate, and carotid canal can all be well seen on HRCT. A careful study of the HRCT can also reveal anatomic variations that may affect surgery, such as dehiscence of the facial nerve canal. Similarly, loss of the normal bone overlying any of these structures may give a valuable warning of involvement by cholesteatoma.
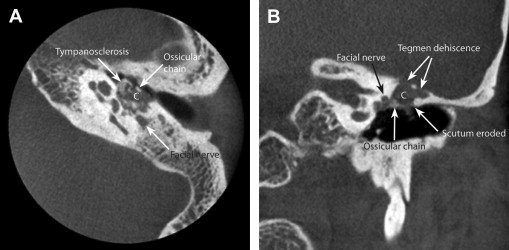
When ossicular or mastoid bony erosion is seen in association with a soft tissue mass, HRCT can distinguish cholesteatoma with specificity between 80% and 90%. In the postoperative period, HRCT has a high negative predictive value when it shows a well-aerated middle ear with no evidence of soft tissue densities. However, HRCT has proved unreliable in differentiating residual or recurrent cholesteatoma from granulation tissue, cholesterol granuloma, mucosal edema, fibrosis, scar tissue, or fluid. However, in patients who have undergone previous tympanomastoidectomy, the relevance of bony erosion is lost because it is difficult or impossible to differentiate surgical changes from pathologic bony destruction caused by cholesteatoma. In this setting, HRCT has a sensitivity of 43%, specificity of 42% to 51%, and a predictive value of 28% in detecting residual or recurrent cholesteatoma.
The introduction of in-office cone beam computed tomography (CBCT) imaging has made imaging for cholesteatoma more convenient and accessible. As a result of their favorable radiation safety profile and compact size, CBCT scanners can be assembled in clinic rooms with often minimal requirements for specialized shielding. In CBCT scanners, the x-ray beam forms a cone-shaped geometry between the imaging source (apex of the cone) and the detector (base of the cone). In contrast, conventional scanners have a fan-beam geometry. The radiation dose of these scans is reported to be 60% of a conventional computed tomography (CT) scanner when evaluating middle ear structures, but middle and inner ear bony structures are seen equally well in CBCT and conventional HRCT scanners. One disadvantage of in-office CBCT is the limited anatomic coverage, which means inner ear or more distal disorders in the mastoid may be missed. An additional CBCT disadvantage is the lack of any soft tissue contrast, and these scanners are typically used only to assess bony anatomy. A general disadvantage of both HRCT and CBCT is their use of ionizing radiation, and hence their intrinsic potential for inducing malignancy. Therefore, clinicians must always be judicious in their use, particularly in children who may be sensitive to cumulative radiation effects.
MRI
Although MRI cannot provide a map of the bony geometric framework of the temporal bone for surgical planning, selected MRI techniques can provide valuable information regarding the presence, size, and approximate location of cholesteatoma that may not be available on HRCT imaging. MRI also has the advantage of not requiring exposure to radiation, although it does require longer acquisition times compared with HRCT, and the need for immobilization may make it difficult to obtain in young children.
On conventional MRI sequences, cholesteatomas and epidermoids appear dark on T1-weighted images, bright on T2-weighted images, and do not enhance with intravenous contrast unless acute infection results in rim enhancement. These signal characteristics render them difficult to distinguish from much of the other soft tissue present in a chronic ear condition unless they are large. One mechanism to circumvent this limitation has been the use of delayed-contrast techniques. Delayed-contrast MRI has been used to better detect recurrent cholesteatoma by taking advantage of the fact that other tissue, such as fibrosis or granulation tissue, often takes up more contrast given sufficient time, whereas cholesteatomas do not. In this technique, T1 images are obtained 30 to 45 minutes after intravenous (IV) paramagnetic contrast administration (gadolinium), which results in enhancement of inflammatory mucosa, granulation tissue, scar, or fibrosis. Absence of contrast enhancement in a lesion suggests cholesteatoma. De Foer and colleagues reported sensitivity and specificity for delayed-contrast MRI in detecting cholesteatoma as 56.7% and 67.6% respectively. Overall positive predictive value was 88% and negative predictive value was 27% in the population studied. Disadvantages of using delayed-contrast MRI are (1) the cost and potential morbidity associated with the need for IV contrast; (2) retained secretions, silicone/plastic (Silastic [Dow Corning, MI]) sheets, and calcified scars can mimic nonperfused cholesteatoma; (3) early acquisition of images may lead to false-positives; (4) this technique cannot detect cholesteatomas smaller than 3 mm; (5) it is difficult for scheduling purposes to keep an MR scanner available if immediate and delayed scans are both acquired; and (6) sedation or general anesthesia is required for children because of the prolonged time required for image acquisition. As a result of these limitations, delayed-contrast MRI for detecting residual or recurrent cholesteatoma has never caught on and is not routinely used in most practices.
However, over the last decade the use of diffusion-weighted sequences has provided considerable improvement in the diagnosis of cholesteatoma and skull base epidermoids, and this sequence is now considered an important component of the MRI assessment for both diseases. DWI relies on the principles of molecular diffusion or brownian motion. Molecular diffusion refers to the haphazard movement of water molecules, which is restricted in certain pathologic conditions, including in the presence of organized keratin debris as seen in both cholesteatoma and epidermoids. In regions where the diffusion of water is impeded or restricted, there is less dephasing of protons and more signal is retained, and hence the tissue with restricted diffusion is seen as bright on the diffusion-weighted image. The keratin debris associated with cholesteatomas and epidermoids restricts water diffusion, and this leads to a high signal intensity in this material on DWI compared with brain or other surrounding soft tissues. Granulation tissue, fibrosis, and mucosal edema are less restricting of water motion and do not lead to high signal on DWI.
Two broad categories of DWI algorithms can be used for initial evaluation of cholesteatoma and epidermoids, or detection of residual or recurrent cholesteatoma: echo-planar and non–echo-planar DW-MRI. The first algorithm developed was echo-planar DWI, and many articles have described its use in detecting cholesteatomas. Echo-planar imaging (EPI)–based methods are fast and reliable, but they produce considerable distortion at the skull base and temporal bone related to the numerous interfaces among air, bone, and soft tissue, and to the inhomogeneity of the magnetic field that results from these interfaces, as discussed in more detail later. Non-EPI DW methods are typically either single-shot turbo-spin echo sequences (half Fourier acquisition single-shot turbo-spin echo [HASTE; Siemens Systems, Germany]) or multishot turbo-spin echo sequences (periodically rotated overlapping parallel lines with enhanced reconstruction [PROPELLER]; BLADE [Siemens Systems, Germany]), and they are less subject to distortion at the skull base.
As mentioned earlier, EPI DWI is subject to artifacts at the interfaces between tissues, especially when air or bone is adjacent to soft tissue. These magnetic susceptibility artifacts relate to local magnetic field inhomogeneities caused by tissues of markedly different composition; they can also occur in the vicinity of metallic foreign bodies, such as surgical clips or staples, or dental work. However, the mastoid and middle ear produce susceptibility artifacts caused by natural air-bone interfaces, and this causes image distortion. Multiple studies have shown the inability of EPI DWI to detect cholesteatomas smaller than 5 mm. Studies have also shown newer, non-EPI DWI methods to be superior to EPI DWI in detecting recurrent or residual cholesteatoma, and thus non-EPI DWI has become the standard for MRI imaging of cholesteatoma. Skull base epidermoids located in the cerebello-pontine angle (CPA) and petroclival junction, have less susceptibility artifacts.
Various studies, including a recent meta-analysis, have evaluated DW-MRI for the detection of residual and recurrent cholesteatomas. In the meta-analysis, the overall sensitivity of this imaging modality was 94% with a specificity of 94%. Most of the false-negatives reported were caused by cholesteatoma pearls less than 3 mm in size. False-positives reported in this study were caused by susceptibility artifacts, cholesterol granuloma, abscess, or bone powder; in some of these cases the image showed a true disorder, but this disorder was not necessarily cholesteatoma.
Although MRI can be helpful in imaging of cholesteatoma under specific circumstances, the cost of MRI is approximately double that of HRCT. Although clinicians should consider this additional economic impact, the benefits gained in selected patients by avoiding needless surgery, or by preventing a delay in diagnosis, can potentially justify its use on economic grounds.
Indications for imaging in cholesteatoma and epidermoids
Experts may disagree about the indications for imaging in cholesteatoma and about the extent to which it assists in treatment decisions. Some otologists routinely obtain imaging whenever cholesteatoma is seen or suspected, whereas others use imaging infrequently. Most agree that imaging is indicated in revision cases and those with intracranial or intratemporal complications. Surgeons should carefully consider the benefits they receive from imaging in their own practices, and they should regularly reevaluate imaging indications and referrals as they gain experience and perhaps modify their surgical techniques accordingly. Surgeons should also be diligent about reviewing imaging studies themselves, because even the best radiology report rarely conveys all the subtleties that may affect surgery.
For lesions located in the skull base, such as epidermoids, imaging is routinely obtained.
Preoperative Assessment
The benefits of being aware of potential challenges and of having a CT-based guide for surgical planning are particularly helpful in teaching settings, so that expectations for the case can be reviewed preoperatively. Similarly, HRCT can be helpful before revision surgery, especially when the surgeon did not perform the initial procedure. In revision cases, anatomy may be considerably altered, limiting the utility of normal surgical landmarks and presenting unexpected challenges.
HRCT can reveal specific patterns of pneumatization and aeration or variability in the position of the sigmoid sinus or tegmen, which may affect surgical access to the disorder. Is a mastoidectomy needed, or can the disease be adequately accessed via a transcanal approach? Is there likely to be adequate space to access disease with the canal wall left up, or is the mastoid sclerotic and contracted, warranting a canal-wall-down procedure? Erosion of the Fallopian canal may be suggested, as can exposure of the carotid artery or jugular bulb, and these findings are important alerts to potential hazards during dissection. Some labyrinthine fistulae are clinically silent, as are almost all facial nerve canal erosions, thus preoperative knowledge of these findings may alert the surgeon to areas that warrant extra intraoperative care and attention. Although the ossicles are difficult to assess completely, obvious ossicular abnormalities may predict the need for ossicular reconstruction. HRCT can also show unexpected and potentially unrelated anatomic variations such as anomalous facial nerve patterns.
Despite MRI’s superior ability to identify cholesteatoma and differentiate it from other soft tissues, it is seldom helpful in the preoperative setting in primary cases unless there is a question about the preoperative diagnosis of cholesteatoma; in these cases, DW-MRI can provide additional information when clinical information is limited or the otoscopic examination is inconclusive. However, most of the time the diagnosis is not in doubt and HRCT is superior in providing information on relevant anatomic geometry. DW-MRI becomes considerably more useful in assessing the potential for postoperative recurrence of disease. In such cases, cholesteatoma may appear in areas inaccessible to clinical otomicroscopy or in unexpected areas, including the mastoid cavity, deep to reconstructive materials, and growing around adjacent structures where the furthest extent of cholesteatoma may have been missed on the primary procedure ( Fig. 2 ). DW-MRI images must be interpreted in conjunction with other MRI sequences, because not all high-signal-intensity tissue on DW-MRI is cholesteatoma. In these cases, the use of other MRI sequences may be useful to predict an alternative diagnosis such as cholesterol granuloma, and to provide the surgeon and patient with expectations for treatment.
