Endoscopic endonasal approaches have widely accepted techniques for managing benign and malignant processes along the entire ventral skull base with similar or better results compared with open procedures, but with lower rates of complication. Managing pathology affecting the skull base can be challenging because of complex anatomy and the proximity of critical neurovascular structures. Postoperative imaging can be challenging, because of surgical alterations of normal anatomy and the now common use of complex reconstruction techniques. Understanding the normal imaging appearance of skull base reconstruction is important for accurate postoperative interpretation and delineation between normal reconstructive tissue and recurrent neoplasm.
Key points
- •
The feasibility of endoscopic endonasal approaches, the potential surgical risk, and tumor resectability depend on critical anatomic structures in the surgical corridors that can be assessed by preoperative imaging studies.
- •
Computed tomography scan yields the best overall evaluation of the bony architecture of the skull base.
- •
MRI is the best imaging modality to evaluate the soft tissues and to demonstrate the location of a lesion and its relationship to adjacent neurovascular structures.
- •
Neoplastic recurrences typically occur at the interface of the flap recipient bed and reconstructive tissue and appear as new or growing infiltrative enhancing tissue similar to the resected tumor.
ASB | Anterior skull base |
CN | Cranial nerve |
CSB | Central skull base |
CSF | Cerebrospinal fluid |
CT | Computed tomography |
CTA | Computed tomography angiography |
DWI | Diffusion-weighted imaging |
EEA | Endoscopic endonasal approaches |
ESBR | Endoscopic skull base reconstructions |
FDG-PET | F18-fluorodeoxyglucose positron emission tomography |
FLAIR | Fluid-attenuated inversion recovery |
ICA | Internal carotid artery |
IOF | Inferior orbital fissure |
ITAC | Intestinal type adenocarcinomas |
MRA | Magnetic resonance angiography |
MRI | Magnetic resonance imaging |
NPL | Nasopalatine line |
NSF | Nasoseptal flap |
PPF | Pterygopalatine fossa |
SOF | Superior orbital fissure |
STIR | Short-tau inversion recovery |
T1WI | T1-weighted image |
T2WI | T2-weighted image |
Introduction
Although traditional open techniques still play an important role in skull base surgery, they continue to carry significant perioperative risks and morbidity with prolonged recovery times, in part because they require some degree of brain retraction and neurovascular manipulation to access the skull base. In an attempt to address some of the shortcomings of open skull base surgery, less invasive endoscopic endonasal approaches (EEAs) to the skull base have been developed and have become increasingly accepted and widely adopted.
In recent years, EEAs have become standard for the treatment of a variety of sinonasal and central skull base diseases. Important factors leading to the popularization of these techniques include an improved understanding of the anatomy of the skull base anatomy; imaging advances that allow easy acquisition and reconstruction of high-resolution, multiplanar images of the skull base; and the rapid development of imaging-based operative navigation systems. In modern practice, the preoperative radiographic evaluation is not only useful for diagnosis but is also invaluable for surgical mapping to ensure safe and optimal surgical outcomes. These techniques were initially developed for paranasal sinus surgery, but their indications have been gradually extended to include endoscopic resection of pituitary tumors, as well as lesions of the clivus, olfactory cleft, planum sphenoidale, petrous apex, and infratemporal fossa. The EEA provides access to almost all regions of the skull base situated anterior to the foramen magnum.
Principles of endoscopic endonasal skull base surgery involve selecting a surgical corridor with an optimal visual field, thus minimizing the need for neurovascular manipulation. If these preconditions are met, the outcomes after an EEA compare favorably with conventional techniques, while providing the advantages of a lack of external incisions or scars, decreased trauma to soft tissue and bone, less disturbance of craniofacial growth in pediatric patients, fewer complications, reduced risk of neurologic damage, improved postoperative quality of life, decreased lengths of hospital stays, and faster recovery times.
A major criticism of endoscopic techniques is that they do not allow en bloc resection of tumors. However, the tumors are also often fragmented in the course of open surgery, and the most important aspect is not en bloc resection, but complete resection of the tumor margins. Tumors of the skull base often demonstrate exophytic growth into paranasal sinuses from a smaller pedicle. However, the endonasal approach often allows resection without damaging adjacent healthy tissues, which is not the case with conventional open surgery, in which the skin, bone, and sometimes dura mater are opened to provide access to the tumor, with a risk of tumor seeding.
The aims of this article were to review techniques for imaging the skull base, to depict the anatomy of the anterior and central skull base, to illustrate the most common cranial base pathologies that may be amenable to EEA, to briefly review the various endoscopic approaches in EEA and key presurgical anatomic considerations and contraindications to each of these, and to understand the expected postoperative imaging appearance of the common endoscopic skull base reconstructions (ESBR), recognizing the most frequent complications.
Introduction
Although traditional open techniques still play an important role in skull base surgery, they continue to carry significant perioperative risks and morbidity with prolonged recovery times, in part because they require some degree of brain retraction and neurovascular manipulation to access the skull base. In an attempt to address some of the shortcomings of open skull base surgery, less invasive endoscopic endonasal approaches (EEAs) to the skull base have been developed and have become increasingly accepted and widely adopted.
In recent years, EEAs have become standard for the treatment of a variety of sinonasal and central skull base diseases. Important factors leading to the popularization of these techniques include an improved understanding of the anatomy of the skull base anatomy; imaging advances that allow easy acquisition and reconstruction of high-resolution, multiplanar images of the skull base; and the rapid development of imaging-based operative navigation systems. In modern practice, the preoperative radiographic evaluation is not only useful for diagnosis but is also invaluable for surgical mapping to ensure safe and optimal surgical outcomes. These techniques were initially developed for paranasal sinus surgery, but their indications have been gradually extended to include endoscopic resection of pituitary tumors, as well as lesions of the clivus, olfactory cleft, planum sphenoidale, petrous apex, and infratemporal fossa. The EEA provides access to almost all regions of the skull base situated anterior to the foramen magnum.
Principles of endoscopic endonasal skull base surgery involve selecting a surgical corridor with an optimal visual field, thus minimizing the need for neurovascular manipulation. If these preconditions are met, the outcomes after an EEA compare favorably with conventional techniques, while providing the advantages of a lack of external incisions or scars, decreased trauma to soft tissue and bone, less disturbance of craniofacial growth in pediatric patients, fewer complications, reduced risk of neurologic damage, improved postoperative quality of life, decreased lengths of hospital stays, and faster recovery times.
A major criticism of endoscopic techniques is that they do not allow en bloc resection of tumors. However, the tumors are also often fragmented in the course of open surgery, and the most important aspect is not en bloc resection, but complete resection of the tumor margins. Tumors of the skull base often demonstrate exophytic growth into paranasal sinuses from a smaller pedicle. However, the endonasal approach often allows resection without damaging adjacent healthy tissues, which is not the case with conventional open surgery, in which the skin, bone, and sometimes dura mater are opened to provide access to the tumor, with a risk of tumor seeding.
The aims of this article were to review techniques for imaging the skull base, to depict the anatomy of the anterior and central skull base, to illustrate the most common cranial base pathologies that may be amenable to EEA, to briefly review the various endoscopic approaches in EEA and key presurgical anatomic considerations and contraindications to each of these, and to understand the expected postoperative imaging appearance of the common endoscopic skull base reconstructions (ESBR), recognizing the most frequent complications.
Imaging technique
Given the diversity of pathologic findings of the skull base, a combination of imaging studies may be required to assess a particular lesion fully. Computed tomography (CT) and MRI often play complementary roles in evaluation of the skull base pathology, and both may be considered essential for an initial radiologic workup.
High-resolution nonenhanced CT reconstructed with a bone algorithm yields the best overall evaluation of the bony architecture of the anterior and central skull base. Cortical margins, neuroforamina, trabecular bone, and tumoral or soft tissue calcifications can be evaluated with excellent detail.
MRI is considered the best imaging study to evaluate the soft tissues of the skull base. MRI further details the location of a lesion and its relationship to adjacent neurovascular structures. In cases of a sinonasal tumor with skull base involvement, MRI distinguishes the neoplasm from inspissated secretion and defines the extranasal and intracranial extent of the tumor. The presence of fat in the soft tissues and in the marrow spaces is extremely useful in defining normal anatomy and in identifying areas of potential pathology, particularly on spin-echo T1-weighted images (T1WI), on which fat demonstrates high signal intensity. Short-tau inversion recovery (STIR) or fat-suppressed T2-weighted images (T2WI) are used to suppress signal related to fat and maximize T2 signal related to infection, inflammation, fluid, or neoplasm. These sequences are commonly performed in the axial and/or coronal planes.
Postcontrast gadolinium-enhanced images should be performed in at least 2 planes (usually the axial and coronal planes) and are essential for detecting disease of the suprahyoid soft tissues, the bone marrow, and the meninges, as well as perineural tumor spread. One or both of these enhanced sequences are typically performed with fat saturation. However, with fat-suppression techniques, susceptibility artifacts are common in the skull base because of field inhomogeneity and multiple air-bone–soft tissue interfaces. Table 1 outlines our MR protocol for imaging of the skull base. This protocol includes whole brain and dedicated skull base sequences and extends from the vertex to the hard palate.
Sequence | Plane | Slice Thickness, mm | Coverage |
---|---|---|---|
Precontrast | |||
EPI DWI | Axial | 5 | Entire brain |
Spin-echo T1W | Sagittal | 4 | Entire brain |
Fat-suppressed TSE T2 | Axial | 5 | Entire brain |
FLAIR | Axial | 5 | Entire brain |
Fat-suppressed TSE T2W | Axial | 4 | Paranasal sinuses and skull base |
TSE T1W | Axial | 4 | Paranasal sinuses and skull base |
3D T1 MPRAGE | Axial | 1 | Entire brain and skull base |
Postcontrast | |||
Fat-suppressed SE T1W | Axial | 4 | Paranasal sinuses and skull base |
Fat-suppressed SE T1W | Coronal | 3 | Tip of nose through brainstem |
3D T1 MPRAGE | Axial with additional sagittal and coronal reconstructions | 1 | Entire brain and skull base |
3D CISS | Axial | 0.7 | Paranasal sinuses and skull base |
Ultimately, imaging protocols may need to be individualized depending on the particular lesion or clinical question. Pituitary adenoma evaluation, for instance, often requires dynamic contrast-enhanced imaging, for detection of small lesions. Magnetic resonance angiography (MRA) or CT angiography (CTA) can be used to evaluate the vessels of the skull base and their relationships to resection targets. High-resolution heavily T2-weighted sequences are excellent at depicting cranial nerves (CNs) and vessels as they course through the basilar cisterns.
Other modalities may occasionally be used to evaluate the skull base. F18-fluorodeoxyglucose positron emission tomography (FDG-PET) can be used in the posttreatment follow-up of skull base tumors to differentiate posttreatment changes from persistent or recurrent neoplasm. No routine role has been found in the primary evaluation of patients presenting with skull base lesions, however. Conventional angiography may be necessary for preoperative embolization of highly vascular lesions to facilitate surgical resection and to reduce intraoperative hemorrhage.
Anatomy of anterior skull base
The floor of the anterior cranial fossa, often referred to as the anterior skull base (ASB), is formed by the roof of the nasal cavity and ethmoid sinuses, the roof of the orbits on either side of the ethmoid roofs, and the lesser wings of the sphenoid bone posteriorly.
The midline cribriform plate, which forms the roof of the nasal cavity, is traversed by multiple olfactory nerves that extend from the olfactory mucosa to the olfactory bulbs. The cribriform plate is separated into right and left halves by the insertion of the nasal septum. On either side of the cribriform plate is the fovea ethmoidalis or ethmoid roof, which is formed by the medial extension of each orbital plate and is generally located more superiorly than the cribriform plate. A thin, usually vertically oriented bone plate (lateral lamella), connects the cribriform plate with each fovea ethmoidalis. In adults, the olfactory fossae are variable depressions on either side of the crista galli, which contain the olfactory bulbs; each fossa is bounded inferiorly by the cribriform plate and laterally by the lateral lamella ( Fig. 1 ). Because of the relative fragility of the bone of the cribriform plate and fovea ethmoidalis, they can be easily violated if care is not taken during EEA. The middle turbinate is delicately attached to the cribriform plate, so detachment of this structure at its insertion during EEA may tear the adjacent dura, resulting in cerebrospinal fluid (CSF) rhinorrhea. Although the thin cribriform plate is easily crossed by tumors, the orbital plates of the frontal bone, which are made of thick compact bone, constitute a barrier to tumor growth into the anterior cranial fossa. Therefore, it is not surprising that most tumors violating the anterior skull base arise from the sinonasal region.
The ethmoid cells are divided by the posterior attachment of the middle turbinate (the basal lamella) into an anterior group, which drains anteriorly into the ethmoid bulla and hiatus semilunaris, and a posterior group which drains into the sphenoethmoidal recess ( Fig. 2 ). The basal lamella extends superiorly within the ethmoid complex to reach the fovea ethmoidalis. The anterior ethmoid cells comprise the anterior two-thirds to three-quarters of the ethmoid complex. In the adult, the basal lamella is usually not a straight bony partition, but rather a curved structure almost inseparable from the other ethmoid septae, because it becomes remodeled by adjacent ethmoid cells as they develop. There is no basal lamella present along the anterior attachment of the middle turbinate. Instead, it is replaced by a medial lamella that attaches to the lateral cribriform plate. The posterior ethmoid cells are important because of their variable relationship with the sphenoid sinus and their intimate association with the optic nerve at the orbital apex.
The optic canal transmits the optic nerve and the ophthalmic artery. The anterior cranial base faces the frontal lobes with the gyri recti medially and the orbital gyri laterally, along with the branches of the anterior cerebral arteries medially and middle cerebral arteries laterally.
Another important anatomic consideration is the medial orbital wall. Most of its surface is exceedingly thin (lamina papyracea). This is formed by a small portion of the frontal process of the maxilla, the lacrimal bone, the ethmoid bone, and the body of the sphenoid. Anteriorly is the lacrimal groove for the lacrimal sac. The groove communicates below with the nasal cavity through the nasolacrimal canal, which is approximately 1 cm long and contains the nasolacrimal duct, which drains into the inferior meatus of the nasal cavity. Also in the medial wall are 2 canals for the anterior and posterior ethmoidal nerves and vessels. These canals are situated at the level of the floor of the anterior cranial fossa, as their lower margins are formed by the upper margin of the ethmoid bone and their upper margins are formed by the under surface of the frontal bone. The anterior ethmoidal foramen is located at the frontal-ethmoidal suture and transmits the anterior ethmoidal vessels and nerve. The posterior ethmoidal foramen transmits the posterior ethmoidal vessels and nerve. These arteries often need to be identified and ligated/coagulated during procedures in the anterior cranial base ( Fig. 3 ).
Another important vessel to consider is the orbitofrontal branch of the anterior cerebral artery, due to its proximity to the anterior cranial base and the increased risk of injury to the vessel during procedures extending into the anterior cranial fossa ( Fig. 4 ). Because of its small size, however, this vessel can be difficult to consistently visualize on noninvasive angiographic techniques like CTA and MRA.
Anatomy of the central skull base
The sphenoid bone is the foundation of the central skull base (CSB), which also contains the pituitary gland within the sella turcica and the parasellar cavernous sinuses. This bone and its foramina can be involved by primary pathologic processes of bone, extracranial disease that can extend intracranially, and intracranial disease that can extend extracranially.
The shape of the sphenoid bone resembles that of a bird with wings outstretched. The central sphenoid body is a roughly cuboidal structure that houses the sphenoid sinuses, and the superior surface of the sphenoid body contributes to the floor of the anterior cranial fossa. Specifically, just posterior to the ethmoid bone, the ethmoid process of the sphenoid bone forms the flat region known as the planum sphenoidale, which represents the anterior portion of the roof of the sphenoid sinuses. The planum lies between the lesser sphenoid wings and separates the cribriform area anteriorly from the chiasmatic sulcus of the sella posteriorly ( Fig. 5 ). The greater wings of the sphenoid sweep laterally from the sphenoid body, reaching and contributing to the lateral surface of the calvarium. The lesser wings form the superior margin of the superior orbital fissure (SOF). The anterior clinoid processes define the posterior margins of each lesser wing. The pterygoid processes and their medial and lateral plates drop inferiorly from the junction of the body of sphenoid bone and the greater wing. The sphenoid bone is separated from the petrous apex of the temporal bone on either side by foramen lacerum.
Many of the important neurovascular foramina of the skull base lay in this central compartment, including the intracranial opening of the SOF, which is traversed by CNs III, IV, VI, and V1 and the superior ophthalmic vein on their way from the cavernous sinus to the orbit; foramen rotundum, which gives passage to CN V2 between the cavernous sinus and the pterygopalatine fossa (PPF); the vidian canal, which contains the vidian nerve and artery extending from foramen lacerum to the high PPF; foramen ovale, which conveys V3 to the masticator space; and foramen spinosum, which is crossed by the middle meningeal artery ( Figs. 6–8 ).
The PPF is a primarily fat-filled space that tapers inferiorly into a common bony palatine canal that carries the palatine nerves. This canal ultimately divides into separate greater and lesser palatine canals, transmitting their respective nerves to the posterior hard palate. Superiorly, the PPF is contiguous with the inferior orbital fissure (IOF). The maxillary nerve continues from the PPF through the IOF, becoming the infraorbital nerve. Laterally, the PPF communicates with the infratemporal fossa through the pterygomaxillary fissure. Medially, the PPF is contiguous with the submucosa of the posterior nasal cavity through a gap or notch in the perpendicular plate of the palatine bone, referred to as the sphenopalatine foramen (see Figs. 6 and 7 ; Fig. 9 ).
The body of the sphenoid bone also houses the sella turcica, which is located behind the planum sphenoidale and is bounded anteriorly by the tuberculum sellae and posteriorly by the dorsum sella. The posterior surface of the dorsum is continuous with the posterior surface of the body of the sphenoid and basiocciput, which collectively form the clivus. The sella turcica is covered superficially by a thin layer of dura, the diaphragma sellae, which stretches across the top of the sella and is perforated centrally by the pituitary stalk (see Figs. 5–7 ).
The pituitary gland, also called the hypophysis, is a reddish-gray, bean-shaped gland with 2 distinct parts or “lobes,” which differ in embryologic origin, structure, and function. These parts are the anterior pituitary (adenohypophysis) and the posterior pituitary (neurohypophysis). The overall height of the pituitary gland on coronal T1WI varies with both age and gender. In prepubescent children, a height of 6 mm or less is normal; in young girls of menstrual age, 9 mm is the upper limit of normal; and in pregnant women, the pituitary gland can measure 12 mm. The upper limit of normal in adult men and postmenopausal women is 8 mm. The neurohypophysis usually is of higher signal intensity on T1WI (the so-called posterior pituitary “bright spot”) caused by the presence of neurosecretory granules. Of note, the neurohypophysis does not contain lipid and therefore does not suppress with fat-suppression techniques. The pituitary gland does not have a blood-brain barrier, so it normally enhances rapidly and intensely following contrast administration ( Fig. 10 ).
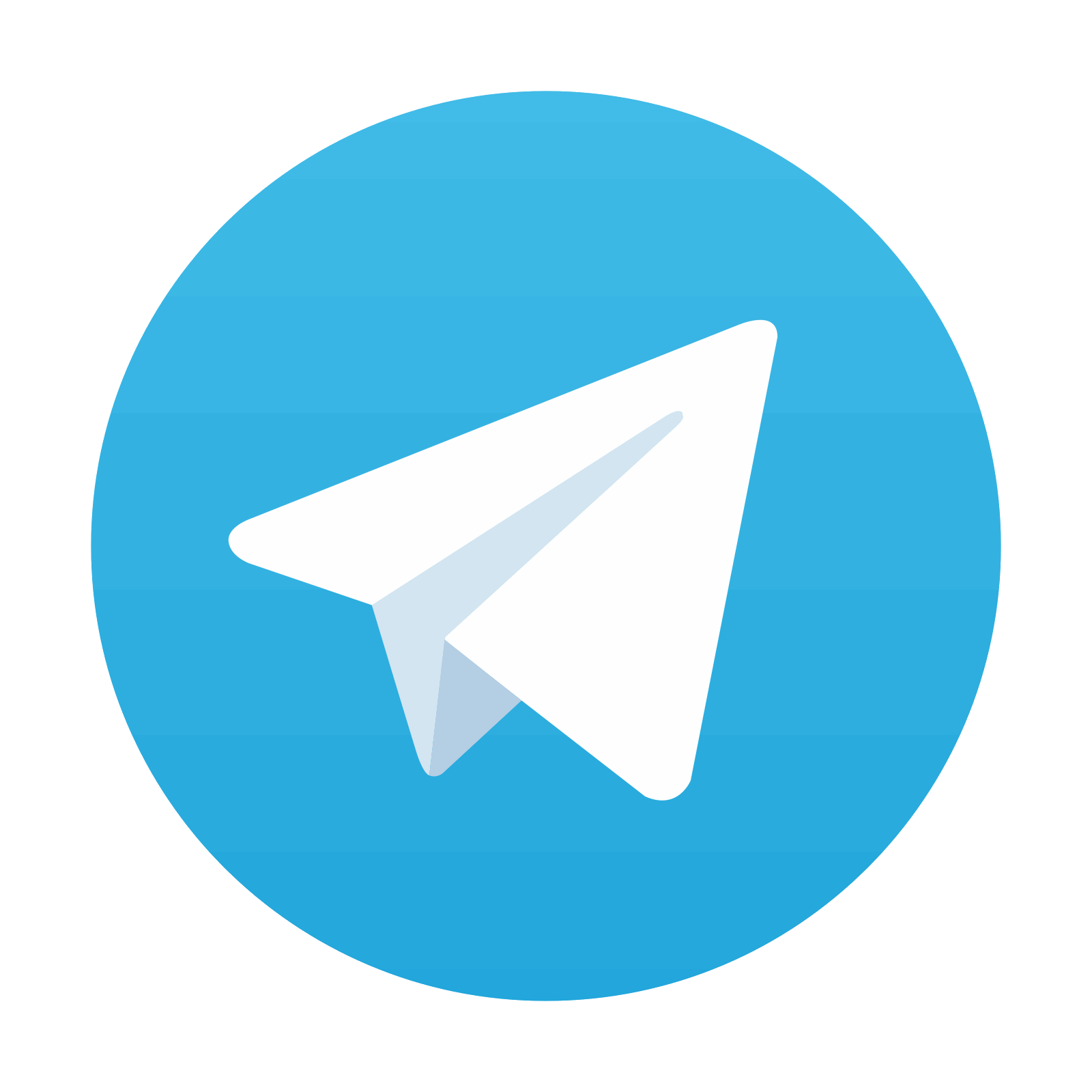
Stay updated, free articles. Join our Telegram channel

Full access? Get Clinical Tree
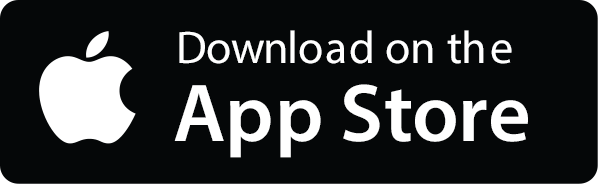
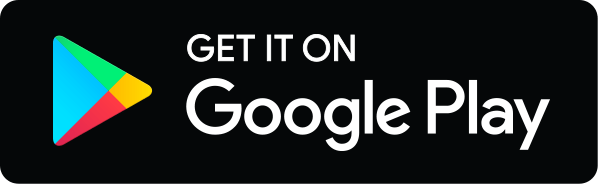
