Introduction
Normal red blood cell hemoglobin comprises four polypeptide globin chains, each associated with a central heme ring (ferroprotoporphyrin). The globin chains consist of two identical pairs of α and β polypeptide chains. The sickle-cell hemoglobinopathies are characterized by a genetic error in β-chain synthesis, which results in abnormal function of the hemoglobin molecule. In conditions of ischemia and metabolic stress, the imperfect globin chains induce pathological alterations in red blood cell morphology. The altered red blood cells are labeled “sickle cells” because of their crescent shape.
Systemic and ocular sequelae are well described. Interestingly, the severity of systemic symptoms does not typically correlate with the severity of ocular manifestations. The most severe systemic complications are observed in sickle SS disease, whereas severe ocular features are most commonly noted in patients with sickle SC or sickle thalassemia (S-Thal) disease. In general, vision-threatening sequelae of the sickle hemoglobinopathies are secondary to ischemia or peripheral retinal neovascularization.
Epidemiology and Pathogenesis
Hemoglobinopathies can be characterized by electrophoresis and by the genetic mutations that lead to abnormal amino acid substitutions in the β-globin chain. Normal adult hemoglobin with two normal α and β chains is termed hemoglobin A. Sickle hemoglobin, known as S, was initially described by Pauling and others in 1949 as a single point mutation that resulted in substitution of the amino acid valine for glutamic acid at the sixth position. The substitution of lysine for glutamic acid at this position results in the manufacture of hemoglobin C. The sickle hemoglobinopathies S and C are only two of many known mutations caused by qualitative errors in globin chain synthesis. Inadequate production of either normal or abnormal globin chains, a quantitative error in globin synthesis, is referred to as thalassemia or Thal.
Since these mutations are inherited, heterozygous or homozygous conditions exist. For example, normal hemoglobin comprises homozygous AA globin chains. Classic sickle cell anemia, or SS disease, usually arises when parents who have sickle trait (AS) disease each pass on their single abnormal S globin chain mutation. In the United States, African-Americans comprise the majority of patients afflicted by the hemoglobinopathies, with sickle trait (Hb AS) affecting approximately 8% and sickle cell disease (SCD) diagnosed in approximately 0.15%. If hemoglobin S is inherited from one parent and hemoglobin C from another, a double heterozygote form of hemoglobin is created known as sickle SC disease, which is nearly as prevalent as SS disease.
Normal hemoglobin confers pliability to the oval-shaped red blood cells, which allows them to pass easily through the microvasculature where they deliver oxygen. Sickle hemoglobins result in red blood cells with a crescentic, elongated shape, particularly under conditions of hypoxia or acidosis. This causes the blood cells to stack, which further exacerbates local ischemia. A vicious cycle of ischemia, red blood cell sickling, tissue hypoxia, and necrosis is set in motion.
Although sickle SS disease has the most severe systemic manifestations, hemoglobin SC and S-Thal have the most severe ocular manifestations with an earlier age of onset. The reasons for this discrepancy are likely multifactorial. The more severe anemia of SS disease is associated with lower red blood cell counts than those in hemoglobin SC and S-Thal disease. Relative anemia may decrease the viscosity of the remaining red blood cells, thereby causing less sludging in the circulation. Another theory posits that SS disease causes more frequent retinal infarction, whereas the less severe hemoglobinopathies such as SC disease cause more ischemia of the retinal tissues. The retinal ischemia may increase the angiogenic response of vascular endothelial growth factor and stimulate neovascularization as opposed to the retinal necrosis induced by infarction.
Since many of the problems are time dependent, the overall prevalence of ocular complications of sickle disease is unknown. In a large cohort, 6% (49/783) of SS patients had proliferative sickle retinopathy (PSR) on initial examination compared with 32% (172/533) of SC patients. Approximately 40%–43% of hemoglobin SC patients and 14%–20% of hemoglobin SS patients can be expected to develop PSR.
Ocular Manifestations
Nearly all ocular or periocular structures can be affected by the sickle hemoglobinopathies. Classic anterior segment findings include conjunctival “comma-shaped” capillaries, which represent intravascular sludging of sickling red blood cells ( Fig. 6.24.1 ). Sectorial iris atrophy represents areas of anterior uveal ischemia. A mild anterior chamber cell and flare reaction may be observed secondary to incompetence of the blood–ocular barrier. The anterior segment manifestations generally do not pose significant risks for vision loss.

Posterior segment manifestations of sickle hemoglobinopathies may be observed in the vitreous body, optic disc, retina, and subretinal structures. Vitreous hemorrhage secondary to peripheral retinal neovascularization may develop. The optic disc may demonstrate sludging red blood cells within prepapillary retinal capillaries, which appear as small dark spots or vascular lines on the optic disc head. The “macular depression sign” represents atrophy and thinning of the inner retinal layers, which results in an oval depression of the bright central reflex and may be associated with a decrease in vision ( Fig. 6.24.2A ). Optical coherence tomography (OCT) has confirmed this finding and demonstrated sparing of the photoreceptors and retinal pigment epithelium ( Fig. 6.24.3 ). OCT angiography also showed a correlation between retinal thickness measurements and vascular density of the fovea and parafoveal regions. More debilitating macular ischemia can result in frank macular infarction with an enlarged foveal avascular zone secondary to multiple retinal arteriolar occlusions with an often insidious, progressive course. Fluorescein angiography reveals irregularity in or enlargement of the foveal avascular zone, with adjacent areas of retinal capillary nonperfusion and retinal vascular remodeling (see Fig. 6.24.2B ). Retinal arterial microaneurysms and cotton–wool spots may also be observed.




Retinal arteriolar sclerosis may be identified in areas in which there is diffuse capillary nonperfusion that reflects prior retinal vascular occlusion. Venous tortuosity is observed in as many as half of all patients who have SS disease and in one-third of patients who have SC disease but is not pathognomonic of sickle retinopathy. Angioid streaks are also described in association with SCD.
Other nonproliferative retinal findings are more characteristic of sickle hemoglobinopathies. Salmon-colored retinal hemorrhages are preretinal or superficial intraretinal hemorrhages that occur adjacent to a retinal arteriole and are often found in the equatorial retina ( Fig. 6.24.4 ). Histopathologically, they dissect into the vitreous cavity or into the subretinal space. The salmon hue is attributed to an evolution of color changes—the initial presentation is bright red. These hemorrhages result from the rupture of a medium-sized retinal arteriole due to ischemic vasculopathy. They usually resolve with few sequelae, although a retinoschisis cavity lined with iridescent refractile yellow particles may persist.

If the intraretinal hemorrhage dissects into the subneural retinal space and disturbs the retinal pigment epithelium, a black sunburst lesion may result ( Fig. 6.24.5 ). These dark, irregularly shaped, spiculated or stellate lesions are the result of retinal pigment epithelial hyperplasia and intraretinal migration. Since they are usually localized to the equatorial retina, the retinal pigment epithelium changes generally do not cause significant visual symptoms.

PSR was classified into five stages by Goldberg :
Stage I: Peripheral arteriolar occlusions.
Stage II: Arteriolar–venular anastomoses.
Stage III: Neovascular proliferation.
Stage IV: Vitreous hemorrhage.
Stage V: Retinal detachment.
This progression of PSR typically occurs in the third or fourth decade of life, but the youngest case of PSR was reported in an 8-year-old patient with SC disease. Peripheral retinal arteriolar occlusion can leave large areas of anterior retinal capillary nonperfusion, which is highlighted well by fluorescein angiography. Curiously, retinal venous occlusion is uncommon in patients who have SCD. Occluded arterioles initially appear dark red but subsequently evolve into “silver wire” vessels. Peripheral arteriolar–venular anastomoses evolve to shunt retinal arterial blood into retinal venules. These anastomoses can be best seen with fluorescein angiography at the junction of perfused and nonperfused retina, typically just peripheral to the equator.
Retinal neovascularization in a seafan configuration is the hallmark of PSR, extending from the border zone of perfused and nonperfused retina (see Fig. 6.24.5 , Fig. 6.24.6 ). Initially, a seafan is supplied by one major feeding retinal arteriole and one major draining retinal venule. Over time, an arborization of the neovascular complex occurs. Seafans most commonly are observed in patients who have SC disease or S-Thal disease and are rare in the other hemoglobinopathies. Fluorescein angiography demonstrates massive leakage of dye into the vitreous ( Fig. 6.24.7 ). The seafans represent a progressive proliferative retinopathy, which exposes the patient to the risks of vitreous hemorrhage and retinal detachment. Seafans may spontaneously involute, resulting in areas of grayish white fibrovascular tissues that often have residual perfused retinal vessels at their base. About 40%–50% of seafans may undergo some degree of autoinfarction during their course.



Vitreous hemorrhage is a common complication of retinal seafan formation. Patients with limited vitreous hemorrhage experience floaters, whereas those with dense vitreous hemorrhage have sudden severe vision loss. These hemorrhages may clear spontaneously with time or may persist to give ochre-colored vitreous membranes.
Retinal seafans may induce fibrovascular tissue on the surface of the retina that causes traction or rhegmatogenous retinal detachments ( Fig. 6.24.8 ). Circumferential seafan involvement can cause peripheral tractional retinal detachment. In the areas of thin, nonperfused retina, atrophic, stretched retinal holes can develop to create combined traction and rhegmatogenous retinal detachments.

Diagnosis
Most patients who present with the ophthalmic complications of sickle hemoglobinopathies are aware of their underlying red blood cell abnormality. Patients with SC and S-Thal disease who have minimal systemic manifestations can be completely unaware of their systemic diagnosis when the ocular complications flare. The characteristic history of hemoglobin SS patients—including multiple hospitalizations secondary to painful crises, chronic end-organ damage, multiple infections, and bony abnormalities—may not have been experienced with the other hemoglobinopathies.
Hemoglobin electrophoresis or DNA-based testing is necessary to characterize the abnormal globin chain type. A positive test for sickling, such as the solubility test (e.g., Sickledex), indicates the presence of hemoglobin S but does not distinguish between SS, AS, and double heterozygotes such as S-Thal and SC.
Diagnosis of the ophthalmic manifestations of the sickle hemoglobinopathies is enhanced by careful examination of the conjunctiva, iris, anterior chamber, optic disc, and macula. Indirect ophthalmoscopy and peripheral retinal contact lens biomicroscopy are helpful to delineate retinal hemorrhages and proliferative changes. Fluorescein angiography characterizes macular perfusion and peripheral retinal perfusion. Early seafan formation is highlighted well by fluorescein leakage. Ultra-wide field imaging may be useful to assess peripheral nonperfusion and the stage of retinopathy (see Fig. 6.24.7 ). Diagnostic ultrasonography can characterize posterior segment anatomy when a media opacity occurs, such as from vitreous hemorrhage or ochre membranes. OCT may demonstrate macular thinning with selective loss of the retinal ganglion cell and nerve fiber layer even in asymptomatic SCD patients. OCT angiography may show abnormalities in the parafoveal vascular system that is more common in patients with SC disease and proliferative retinopathy.
Differential Diagnosis
Other causes of macular ischemia and peripheral retinal neovascularization, vitreous hemorrhage, and neural retinal detachment are listed in Box 6.24.1 .
Other Causes of Macular Ischemia
- •
Diabetic retinopathy
- •
Retinal vascular obstruction
- •
Embolic phenomena such as talc retinopathy
Other Causes of Peripheral Retinal Neovascularization, Vitreous Hemorrhage, and Retinal Detachment
- •
Proliferative diabetic retinopathy
- •
Retinal vein obstruction
- •
The ocular ischemic syndrome
- •
Sarcoidosis
- •
Pars planitis
- •
Retinopathy of prematurity
- •
Familial exudative vitreoretinopathy
- •
Eales’ disease
Systemic Associations
Patients who have classic sickle SS disease manifest a variety of systemic abnormalities, which include anemia, bone marrow infarcts, bony sclerosis (e.g., vertebral “fishmouthing”), aseptic necrosis of the femoral head, ischemia to visceral organs, shortness of breath caused by pulmonary infarcts, and an increase in susceptibility to bacterial infections, particularly salmonellosis caused by reticuloendothelial cell dysfunction. Other hemoglobinopathies result in less severe systemic disease with a mild anemia as the only manifestation.
Treatment
Advances in treatment have reduced the morbidity and increased the life expectancy of patients with SCD over the last 40 years. Most notably, hydroxyurea therapy has been shown to reduce morbidity and mortality by boosting levels of fetal hemoglobin (HbF). In addition, stem cell transplantation in both children and adults with severe disease can reverse the sickle cell phenotype, and gene therapy is under active investigation.
For the ocular manifestations of SCD, treatment efforts focus on altering the course of PSR to reduce the chance of vitreous hemorrhage and retinal detachment. Cryotherapy, diathermy, xenon arc photocoagulation, and argon laser photocoagulation all have been employed to treat peripheral retinal neovascularization. Cryotherapy has been used to treat peripheral retinal neovascularization in the presence of vitreous hemorrhage when the neovascular proliferation is only partially visualized and when vitreous hemorrhage precludes treatment with laser photocoagulation. In recent years, cryotherapy has largely been abandoned because of the significant complication rate.
Various methods of laser photocoagulation effectively induce regression of peripheral neovascularization. Feeder-vessel laser photocoagulation requires intensive treatment with high energy but is more likely to be complicated by chorioretinal or choriovitreal neovascularization, retinal detachment, and vitreous hemorrhage. Scatter laser photocoagulation of areas that surround seafan proliferation and associated areas of ischemic retina can induce lesion regression. The rationale is to ablate areas of ischemic retina that are stimulating neovascular proliferation. This hypothesis is supported by studies demonstrating hypoxia-inducible factor 1α and vascular endothelial growth factor (VEGF) were strongly expressed in retinal cells within avascular (nonperfused) retina in patients with proliferative sickle cell retinopathy. Scatter laser photocoagulation can be performed as a localized treatment confined to areas anterior to the patent neovascular fronds or in a 360° peripheral, circumferential retinal scatter technique to the anterior retina. The rationale of the 360° scatter treatment is to induce regression of existing peripheral retinal neovascularization and to prevent the formation of any future neovascularization. These techniques can be performed with light to moderate intensity burns approximately 500 µm in diameter placed approximately one burn width apart. Scatter laser photocoagulation, either localized or circumferential, is the treatment of choice to prevent complications secondary to peripheral seafan proliferation.
Vitreous hemorrhage secondary to PSR may be followed for up to 6 months to await spontaneous clearing. If areas of retinal neovascularization can be identified through the vitreous hemorrhage, the associated anterior retina should be treated with scatter laser photocoagulation. When the retina is not well visualized, it is important to follow these eyes with ultrasonography, although a minority of patients progress to retinal detachment.
Anti-vascular endothelial growth factor (VEGF) agents have gained increasing roles in the management of many retinal vascular diseases, but their utility in SCD has not been fully investigated. Case reports have described the regression of retinal neovascularization and resolution of vitreous hemorrhage following intravitreal injection of bevacizumab or ranibizumab. However, there are no large-scale studies to assess the long-term effects and incidence of complications.
Surgery on patients who have sickle hemoglobinopathies is fraught with ocular and systemic pitfalls. Systemically, a preoperative workup to assess the severity of anemia, hemoglobin electrophoretic status, and overall systemic condition is critical. Intraoperative and postoperative systemic complications include thromboembolic events such as pulmonary or cerebral embolism. Prophylactic exchange transfusions to increase levels of hemoglobin A may reduce the rate of anterior segment ischemia and optic nerve and macular infarcts. However, studies suggest that exchange transfusions are not needed with modern vitreoretinal surgical techniques.
Adequate hydration and supplemental oxygen often are administered during the perioperative periods. If possible, surgery should be performed under monitored local anesthesia. Vasoconstrictive agents, such as epinephrine in the anesthetic block and pupillary dilating agents, should be avoided. Many advocate lowered intraocular pressure (IOP) to both maximize intraocular perfusion and avoid hemoconcentration. Single doses of carbonic anhydrase inhibitors or intravenous mannitol may be administered to lower IOP but should not be used repetitively.
Anterior segment ischemia does not usually occur if care is taken to avoid elevations of IOP and if the anterior ciliary circulation is not violated. The possibility of anterior segment ischemia is reduced by avoiding treatments in the horizontal meridians, which harbor the long posterior ciliary arteries and avoiding transsection of the horizontal and vertical rectus muscles. With current vitrectomy instrumentation, fluctuations in IOP during vitrectomy surgery are less common. An ultrasound to elucidate the intraocular anatomy is important in cases of vitreous hemorrhage. The peripheral vitreous may be firmly attached to shallow areas of traction retinal detachment at the sites of fibrovascular proliferation and may not be well visualized intraoperatively.
In cases of combined traction-rhegmatogenous retinal detachment, the surgical goals include the release of areas of traction that elevate the retina as well as the removal of fibrovascular proliferation. Once the retina is flat, scatter endolaser photocoagulation is performed. Postoperatively, the patient’s IOP must be monitored closely, particularly if intraocular gas is used.
Patients with sickle hemoglobinopathies who develop postoperative or posttraumatic hyphema are at risk for posterior segment ischemia or infarction even at mildly elevated IOPs. Low oxygen tension and high ascorbic acid levels in the anterior chamber promote sickling of red blood cells, which then can obstruct the trabecular meshwork and lead to a greater elevation of IOP. Aggressive lowering of IOP to less than 25 mm Hg is indicated, with some authors recommending early paracentesis to reduce the IOP in acute situations.
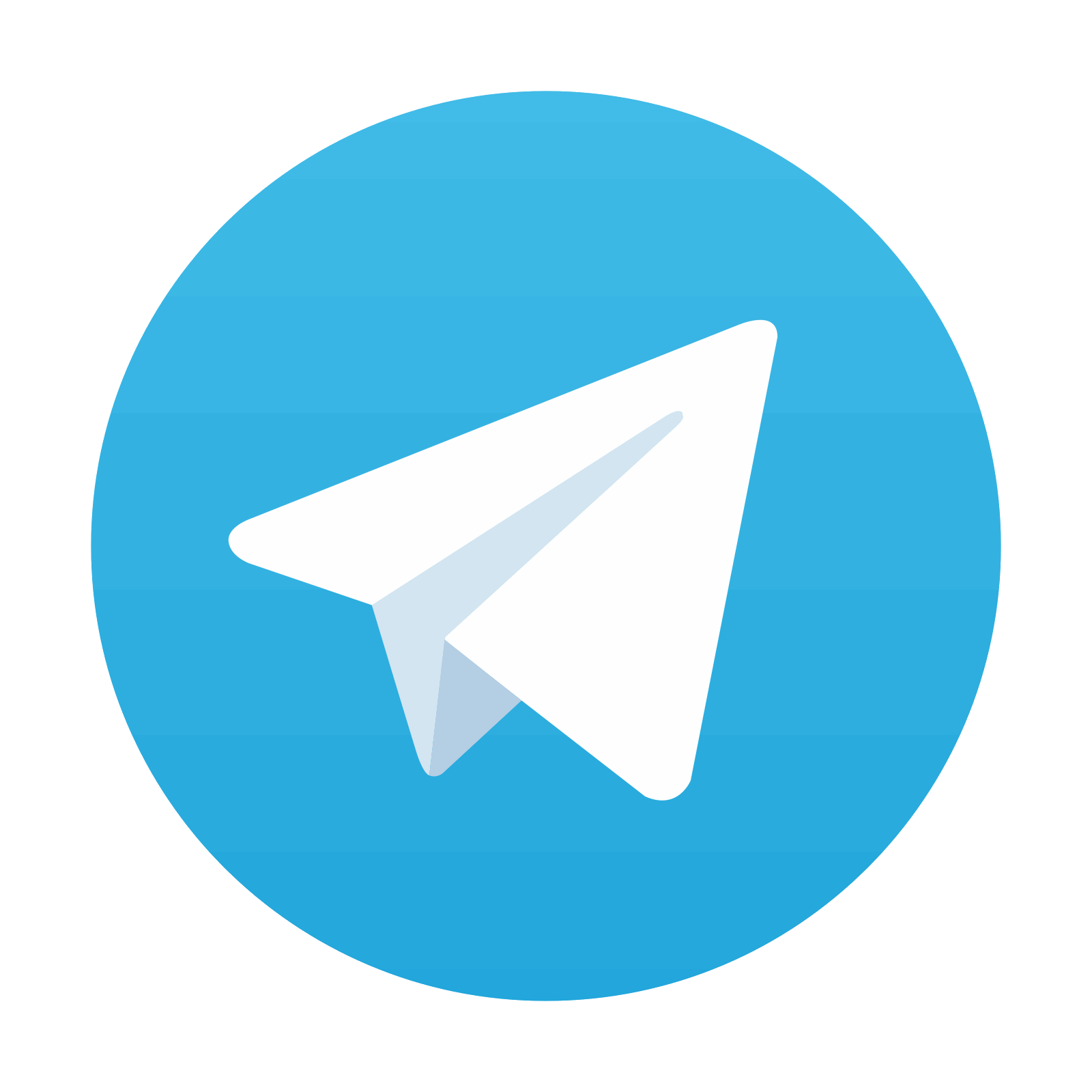
Stay updated, free articles. Join our Telegram channel

Full access? Get Clinical Tree
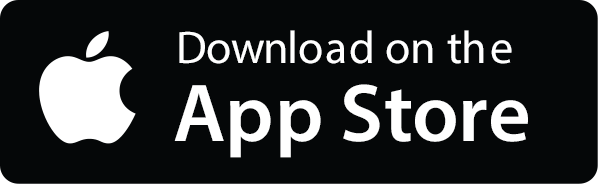
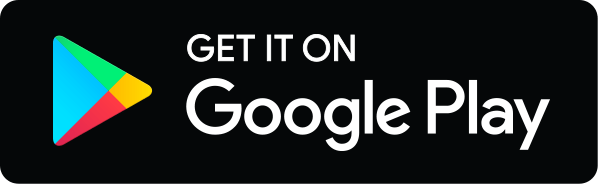