INTRODUCTION
That primary open-angle glaucoma (POAG) and, especially, glaucoma occurring in childhood or associated with other developmental anomalies had familial characteristics has been known since the mid nineteenth century. Von Graefe described multiple families in which glaucoma had appeared in several generations. Family members of patients with POAG are more likely to have the disease than family members of those without glaucoma in the general population. A positive family history of glaucoma (especially a first-degree relative) is indeed a risk factor for POAG. Concordance of glaucoma is higher between monozygotic twins than dizygotic twins as would be expected if the disease was at least partially inherited.
Elevated intraocular pressure (IOP), reduced facility of outflow, increased pressure response to corticosteroids and cup-to-disc ratio all have hereditary tendencies. The Beaver Dam Eye Study demonstrated that elevated IOP has both polygenetic and environmental influences. That same study showed an even greater heritability of optic nerve parameters associated with POAG such as vertical cup-to-disc ratio than even IOP. Similar findings were reported by the Salisbury Eye Study with the heritability of IOP estimated at 0.29 and that of cup-to-disc ratio at 0.56. A recent analysis of the Blue Mountains Eye Study data from Australia showed that genetically influenced IOP variance accounted for about 18% of the glaucoma in that large population study. The studies of both Becker and Armaly found that the development of elevated IOP following 6 weeks of topical corticosteroid treatment did roughly fit an autosomal recessive type of inheritance pattern. However, the pattern of inheritance of the actual disease did not seem to fit any of the classical Mendelian forms of inheritance such as autosomal recessive or sex-linked recessive.
In a different condition, small eyes tend to run in families and angle-closure glaucoma is associated with small eyes. Certain developmental anomalies of the eye that are associated with glaucoma have been known for many decades to be familial in nature. Clearly, genetics plays an important role in many different forms of glaucoma. We still have a long way to go to sort out the effects of genetics versus environment but the serious study of these factors has begun. Science has given us tools that will continually accelerate this knowledge base.
The recent completion of the human genome project has offered a fascinating and hopeful glimpse into the future of medicine. Conceivably, in the not-too-distant future, we will have reclassified the glaucomas into more genetically appropriate conditions, we will be able to identify conditions like congenital glaucoma in utero as well as identify those patients at high risk for open- and closed-angle glaucoma while still in childhood or young adulthood so appropriate monitoring can be accomplished. Identifying the genes associated with various kinds of glaucoma also raises the possibility that we may be able to attach reparative genes to non-pathologic viruses or other vectors and actually prevent these diseases from occurring at all. An example of this kind of possibility has been reported with retinoblastoma; in a family where the father had bilateral retinoblastoma, as did the first born child (as well as pinealoma), the development of retinoblastoma in subsequent children was prevented by a technique called pre-implantation genetic diagnosis in which a single fertilized egg lacking the retinoblastoma gene was chosen for implantion in the uterus from several in-vitro inseminated oocytes. The resulting child did not have retinoblastoma (or any other tumor) with 6 months follow-up. Pharmacogenetics may also allow us to tailor our therapy of those diseases that slip through the genetic screens as well of those that are not genetically reparable to enable each patient to have the most effective and efficient therapy ( Box 20-1 ).
Better understanding of the pathogenesis of the glaucomas.
Newer and better classification based on cellular/molecular mechanisms.
Potential for genetic repair of mutations pre-implantation, in utero or early in life before glaucoma damage occurs.
Identification of potential or actual victims in utero or early in life so appropriate repair, monitoring and/or therapy can be initiated before visual loss.
Development of therapy targeted specifically at the molecular or cellular defect.
Using pharmacogenetics to develop and target individual therapy having the most efficacy and efficiency as well as least side effects.
As far as glaucoma is concerned, none of the promises have yet been realized; we have only just begun to travel down this road. What we have learned so far seems on the surface to be dull and includes an ever increasing list of glaucoma conditions associated with specific gene defects. Despite having found more than a dozen genes associated with one or another form of glaucoma, collectively these discoveries can explain less than 10% of the total cases of glaucoma. This chapter will try to outline what we have achieved so far. Because of improvements in methodology, the knowledge is coming at a faster and faster pace. While the subject matter may be only facts right now, what lies around the corner is exciting. Already, the ability to develop animal models of glaucoma using specific genetic defects (knockout rodents, etc.) has improved our understanding of some pathogenetic mechanisms in glaucoma. The chances are good that soon many of the promises noted above may be realized.
BASIC GENETICS
All of the human traits fit into 23 pairs of chromosomes. Each individual has 22 autosomal pairs and one sex pair which contains either two X chromosomes (female) or one X and one Y chromosome (male). Each of the 23 human chromosomes are made up of thousands of genes which in turn are arrayed along one molecule of DNA. Each chromosome pair contains one DNA molecule from each parent. The DNA is made up of nucleotides each of which is formed by varying sequences of four nucleic acid bases; a codon is a series of three nucleic acid base pairs. It is the sequence of the base pairs and codons ( Fig. 20-1 ) that determine the nature of the amino acid and protein synthesis directed by the DNA nucleotide, and it is the protein which determines a trait. There are over 30000 genes each of which has an average of 3000 base pairs. The chromosomes have anywhere from 20 to 200 million base pairs.

DNA is the template from which RNA is made and RNA governs protein synthesis inside the cell. Groups of DNA bases that are transcribed into messenger (m) RNA which results in protein synthesis are called exons and the DNA strands that form inactive mRNA in between the exons are called introns. When the mRNA gets into the cytoplasm or other parts of the cell where the protein synthesis related to that RNA takes place, the introns are stripped away and only the active mRNA is left to drive protein formation. The RNA builds proteins by adding amino acid sequences. The process is complicated and incompletely understood. However, if an amino acid sequence even of thousands is altered by as little as one amino acid, the abnormal protein produced may be inconsequential or, more likely, devastating to life or function.
Abnormalities of chromosomes come in several varieties. Some are gross enough to be visible with the microscope, such as extra copies of a chromosome, a missing part, a broken chromosome, or abnormal rejoining (translocation). For example, Down syndrome is caused by an extra copy of chromosome 21, so instead of two copies, there are three. This can be diagnosed by karyotype analysis. Most changes in DNA are at the molecular level and cannot be seen by just looking at the chromosome. These changes are called mutations and, as noted above, can involve just one amino acid base pair exchange which can produce profoundly life-altering changes or even fatality. Examples of conditions produced by mutations in a single amino acid sequence in a single gene include sickle cell anemia, cystic fibrosis, Marfan syndrome and Tay-Sachs disease.
GENETIC NOMENCLATURE
The Human Genome Organization (HUGO) designates the name (locus) of the gene. The first three letters indicate the broad category of disease state. For example, GLC indicates primary (rather than secondary) glaucoma. The 1 (2 or 3) following the first three letters further defines the type of glaucoma; for example, 1 is open angle, 2 is closed angle and 3 is congenital. Finally, in chronological order, each newly mapped gene is given a sequential letter; for example, a is for the first, b for the second gene identified, and so on ( Table 20-1 ). The name of the gene does not tell you anything about which chromosome or on what part of the chromosome the gene is located; the location of the gene is indicated by the term ‘map locus.’ The identified glaucoma genes are indicated in Table 20-2 along with a brief phenotype description, the locus, the age at onset, and the name of the gene as it relates to the protein it produces, if known. It is usually the abnormal protein produced by the mutated gene that is responsible for the structural or functional deficit in a genetic disorder.
Name of disease (e.g., glaucoma) | Variant of disease (e.g., primary open angle) | Order of discovery (e.g., first identified in open-angle glaucoma) |
---|---|---|
GLC | 1 | A |
Locus | Location or reference | Gene or protein name | Phenotype | Age of onset | Inheritance | Per cent of phenotype with gene |
---|---|---|---|---|---|---|
GLC1A | 1q23-q25 | TIGR/myocilin | JOAG/POAG | Juvenile/adult | Dominant | 3 |
GLC1B | 2cen-q13 | – | POAG | Adult | Dominant | Very low |
GLC1C | 3q21-24 | – | POAG | Adult | Dominant | Very low |
GLC1D | 8q23 | – | POAG | Adult | Dominant | Very low |
GLC1E | 10p15-14 | Optineurin | POAG/NTG | Adult | Dominant | <5 |
GLC1F | 7q35 | – | POAG | Adult | Dominant | Very low |
OPA1 | 3q28 | OPA1 | NTD | Adult | ? | ≈30% |
GLC3A | 2p21 | CYP1B1 | Congenital | Infant | Recessive | Majority |
GLC3B | 1p36 | – | Congenital | Infant | Recessive | |
NNOS | 11 | – | Angle closure/nanopthalmos | Young–older adult | Dominant | Majority |
RIEG1 | 4q25 | PITX2 | Rieger syndrome | Infant–childhood | Dominant | |
RIEG2 | 13q14 | FOXC1 | Rieger syndrome | Infant–childhood | Dominant | |
IRID1 | 6p25 | FKHL7 | Iridogoniodysgenesis | Infant–child | Dominant | |
7q35 | – | Pigment dispersion | Young adult | |||
NPS | 9q34 | LMX1B | Nail-patella syndrome | Young adult | Dominant | |
PAX6 | Aniridia | Congenital | ||||
LOXL1 | 15q21 | Lysyl oxidase | Exfoliation syndrome and glaucoma | Late adult | ? | 5–10% |
PRIMARY OPEN-ANGLE, NORMAL-TENSION, AND JUVENILE-ONSET OPEN-ANGLE GLAUCOMA
As noted above, POAG has long been known to have hereditary tendencies. This is seen most poignantly in the fact that people of black African ancestry tend to get glaucoma four times more frequently and at an earlier age; the result of this, plus environmental factors such as less access to medical care and, perhaps, less aggressive treatment, is that people of black African ancestry suffer blindness at 13 times the rate of Caucasians. Clearly, both genetic and environmental influences are at work here. Yet, in the late 1980s, heritability of open-angle glaucoma was estimated at 13% with 87% attributed to non-genetic causes, based on Finnish twin studies. Genetic studies are particularly difficult in a disease like POAG because it is diagnosed so late in life and is relatively prevalent. Even in those families with a clearly identifiable gene mutation associated with glaucoma, penetrance is incomplete, the age of onset varies among family members, and the severity of the glaucoma is different. This suggests that multiple genetic factors as well as some, as yet undefined, environmental factors play a role in this disease. Perhaps, one or more genes influence aqueous dynamics and another set of genes (or a gene) determines susceptibility to optic nerve damage.
TIGR/MYOCILIN
The study of genetics in open-angle glaucoma received a needed boost when the first gene (GLC1A) related to this condition was mapped in a large family with juvenile-onset open-angle glaucoma in 1993. The ice was broken, and over the next dozen years, six more loci of genes associated with open-angle or normal-pressure glaucoma were mapped. Point mutations in this gene can cause POAG. Polansky and co-workers identified the abnormal protein produced by mutations in this gene and subsequently were able to determine the exact molecular sequence of this gene, including its promoter, introns, and exons, which he called TIGR (trabecular meshwork-inducible glucocorticoid response). An independent investigation also identified this protein and its concomitant sequence and called it myocilin. The Human Genome Organization then designated MYOC as the official gene symbol for the TIGR/myocilin gene.
Myocilin is a protein with over 500 amino acids. There are two major domains of the molecule, one like myosin and the other olfactomedin – a main component of mucous layers. Olfactomedin is an old protein and can be found across the animal kingdom. In the human eye, the mRNA of myocilin is found not only in the trabecular meshwork but also in the retina, the choroid, ciliary body, aqueous humor, and iris. Myocilin is also found in bones, skeletal muscle, mammary gland, thyroid, and trachea, although in smaller amounts than in the anterior segment of the eye. No systemic disease states have been associated with myocilin mutations or deficiencies.
Several groups were then able to identify mutations in the MYOC gene as being associated with families with juvenile- and adult-onset POAG. It has been estimated that mutations in the MYOC gene account for about 3–5% of the cases of adult-onset open-angle glaucoma as well as a significant portion of familial juvenile-onset glaucoma. Some evidence exists to support the possibility that different mutations in the same gene may produce different aggressiveness of the disease.
That myocilin plays an important role in trabecular meshwork function seems clear. Just how that role interacts with glaucoma and causes dysfunction is not clear. Polansky and his group thought that the myocilin gene governed the response of the trabecular meshwork cells to corticosteroid administration. Since a very large percentage of patients with glaucoma will respond to 6 weeks of topical steroid administration with a significant IOP rise, it seems logical to assume that a gene and its protein product that governs this function might have some relationship to the pathogenesis of glaucoma. Recombinant myocilin when injected into the anterior chamber decreases the outflow facility, whereas other similar proteins do not. If myocilin is overexpressed, trabecular meshwork cells lose adhesive and contractile properties.
Similar effects can be seen if myocilin is present in the extracellular environment. Myocilin has been found to bind to fibronectin in the extracellular matrix of human trabecular meshwork cells.
Liu and Vollrath’s work supports the idea that mutated myocilin causes accumulation of deleterious products in the endoplasmic reticulum of the trabecular cell and that these changes make the cell more susceptible to apoptosis. Carriers of myocilin mutations, particularly the Thr377Met mutation, will have reduced outflow facility by tonography, so proper myocilin activity is necessary for normal trabecular drainage. On the other hand, the trabecular meshwork malfunction in glaucoma does not seem to be due to the amount of myocilin since its absence or excess may not influence actual trabecular meshwork function. However, some evidence points to an increase in the function or change in quality of myocilin as opposed to amount. Mycocilin is found in abundance in the trabecular meshwork of eyes with late-onset open-angle glaucoma and with exfoliative glaucoma along with alpha B-crystallin, a stress protein. Myocilin also seems to be upregulated as a result of mechanical stretching of the trabecular meshwork. Tamm has postulated that mutated myocilin may assume an abnormal shape that either accumulates in the cell causing premature cell death or may physically block the trabecular meshwork, reducing aqueous outflow; direct evidence for this hypothesis has not been produced. Myocilin has been found in the myelinated portions of the optic nerve in humans and, in monkeys, is expressed by astrocytes. The significance of this finding is not clear.
Several mutations of MYOC have been mapped; each has its own amino acid change and location on the chromosome. The most common is the Gln368Stop mutation. At least two dozen other mutations have been identified in addition to the polymorphisms in the Mt1 promoter region described below. These include Gly434Ser, Asn450Asp, Val251Ala, Ile345Met, Ser393Asn, Phe369Leu, G1n368STOP, Val426Phe, Cys433Arg, and Tyr437His. Despite the different loci, the phenotypes of these mutations are rather similar and are characterized by families with early-onset, generally aggressive POAG with the exception of the Gln368STOP mutation. Using case-control methodology, Graul et al could not find any difference in age of onset or clinical course between those having the Gln368STOP mutation and those not having a MYOC mutation.
It is possible that the promoter region of MYOC may be abnormal in some patients. Polansky and co-workers have shown in a retrospective study that patients with advancing glaucoma are more likely to have polymorphisms in the promoter region (Mt1) of MYOC than patients whose glaucoma is stable. Others have failed to find this association. Whether this is due to differences in populations or techniques is not known at this time. A study by Mackey and co-workers in Tasmanian families with early-onset open-angle glaucoma showed that the specific Thr377Met mutation of the MYOC gene, although less common than G1n368STOP mutation, was associated with a younger age of onset, higher IOPs and higher likelihood of having had glaucoma drainage surgery.
The best inference from the often conflicting findings about the MYOC gene and its role in glaucoma is that abnormalities in this gene somehow negatively affect the function of the trabecular meshwork and lead to glaucoma in a significant number of patients with early-onset open angle-glaucoma and in a minority of patients with adult-onset open-angle glaucoma. It is therefore likely that MYOC mutations do play a significant role, at least in a subset of glaucoma patients, but need other enabling genetic and/or environmental factors to cause glaucoma. Further work will undoubtedly help explain the sometimes conflicting observations related to the myocilin gene.
The initial discovery of the MYOC mutations held the promise that relatives of glaucoma patients could be genetically screened early in life. The hope was that those at greatest risk would be identified so that closer monitoring and timely intervention would prevent vision loss. Towards that end, a commercial screening kit became available that screened for MYOC mutations, polymorphisms in the mt.1 promoter and, later, optineurin mutations. As the early promise that the major gene(s) for glaucoma had been identified began to fade, studies appeared that suggested that routine screening for these mutations could not be supported because of the low yield. For example, in the United Kingdom, a study of over 500 glaucoma patients found a mutation in MYOC in only 1.4% and the authors concluded that routine screening for MYOC mutations was not warranted. Similarly, in southern India, MYOC mutations were found in only 2% of patients with POAG. However, Americans of African ancestry have both a higher incidence of glaucoma than those of European ancestry and harbor MYOC mutations more frequently. There are other studies that show a lower incidence of MYOC mutations in black populations. MYOC mutations may account for as much as 5% of the POAG in France and Switzerland, up to 8% of familial glaucoma in Italy, and only 1% in Sweden. Despite evidence that POAG in a black population is inherited via a major co-dominant gene, no mutations in the MYOC gene were found in the Barbados Family Study of Open-Angle Glaucoma despite the high prevalence of glaucoma in this population. A recent study from India implicates MYOC gene mutations in primary congenital glaucoma.
OPTINEURIN
Great excitement accompanied the announcement of the identification of the GLC1E locus on chromosome 10 and its association with a large family with both normal-pressure and high-pressure glaucoma. Sarfarazi and colleagues had already localized this gene to chromosome 10p14. The gene was named optineurin (optic neuropathy-inducing protein), a name which was accepted by the HUGO committee and abbreviated to OPTN. Because optineurin is expressed in the retina and because it is associated with cellular apoptosis, it was thought that mutations in this gene could possibly explain why the optic nerves of patients with normal-pressure glaucoma are more susceptible to optic nerve deterioration. However, many of the patients in the large affected family had high-pressure glaucoma and there are conflicting reports of the importance of this gene to aqueous dynamics.
Polymorphisms in the OPTN gene were linked with both POAG in a large series of Japanese patients and with polymorphisms in the tumor necrosis factor gene. The polymorphisms in this series were associated with specific changes in the amino acid sequences and also seemed to be associated with polymorphisms in tumor necrosis factor-α. Perhaps, these latter sequence variations help modulate severity. Another group of investigators found that mutations in the OPTN gene accounted for approximately 15% of both the POAG and normal-tension glaucoma in a small group of Japanese patients. However, yet another study in Japan failed to find any optineurin polymorphisms in over 300 patients with POAG and normal-tension glaucoma. Although the Blue Mountains Eye Study in Australia found a higher prevalence of mutations of the OPTN gene in patients with high-tension glaucoma compared to non-glaucoma subjects, the association failed to reach statistical significance. None of their low-tension glaucoma patients had OPTN mutations. The same group found OPTN mutations in subjects without clinical evidence of glaucoma; these mutations were present in only 0.09% of the older, non-glaucomatous population. Another study in the US failed to show any mutations in adult-onset open-angle glaucoma. In a Chinese study, mutations in OPTN were found in patients with POAG but they were different mutations than those found in the Caucasian populations with normal-tension glaucoma. In a study of two English families with normal-tension glaucoma, the E50K mutation was associated with earlier onset, had more advanced disc cupping and had a higher likelihood of requiring surgery than patients with normal-tension glaucoma who did not carry the mutation. In conclusion, OPTN mutations are important for a small subset of POAG patients but, because the incidence is so low, it is not practical to conduct screening in general populations.
Clearly, racial differences (and perhaps environmental as well) exist as to what extent certain mutations account for different forms of glaucoma. In a Canadian study, mutations in OPTN were not associated with low-tension glaucoma but with juvenile-onset open-angle glaucoma. An American study found a high prevalence of optineurin variations in families with normal-tension glaucoma but estimated that the gene was responsible for only 0.1% of open-angle glaucoma in the USA. No mutations in OPTN were found in the Barbados Family Study of Open-Angle Glaucoma or in a Swedish group of POAG.
Vittitow and Borras suggested that optineurin served a protective function in the trabecular meshwork because OPTN is upregulated in tissue culture after 2–7 days of sustained elevated IOP as well as after prolonged dexamethasone treatment and by exposure to tumor necrosis factor-α. It would then stand to reason that absence of OPTN or abnormal OPTN would allow for more tissue damage under stress situations than in a normal eye.
OTHER GENES IN OPEN-ANGLE GLAUCOMA
Families with open-angle glaucoma can have other mutations. For example, POAG in both an American and a Greek family maps to the GLC1C locus on chromosome 3. A study of 86 families with open-angle glaucoma implicated GLC1I as one of the most common mutations (17%) and was localized to chromosome 15 (15q11-13). The authors feel that one of the most important phenotypic markers is age at diagnosis and that early age at diagnosis can separate those with mutation-induced glaucoma from those with more multifactorial disease. If these data are borne out by other studies, the GLC1I gene would be the most common mutation identified so far for familial glaucoma. A multicenter group has identified a specific mutation in the WDR36 (GLC1G) locus mapped to chromosome 5 (5q22) as being a causative gene in about 6–7% of families with adult-onset glaucoma. Another large POAG family that maps to this locus did not have mutations in the WDR36 gene. The differences in genetic makeup of some families does indicate that the genetics of glaucoma are complex and no one genetic abnormality found thus far explains all similar phenotypes.
The OPA1 gene, which is responsible for dominant optic atrophy, has also been associated with normal-tension glaucoma in a Caucasian but not Korean population. Refining the screening technique for mutations, Powell and colleagues found a specific and hitherto unreported abnormality in the OPA1 gene in 28% of normal-tension glaucoma patients versus only 13% of controls. On the other hand, Aung and co-workers were unable to find any differences in phenotype between normal-tension glaucoma patients with and without the OPA1 mutation. A similar study in several Caucasian- and African-based populations was unable to find any association between polymorphisms in the OPA1 gene and POAG.
Genome-wide scans can be done in populations and do not depend on families with a known mode of Mendelian inheritance such as autosomal dominant. Such a scan was done on 180 pairs of siblings and linkages to chromosomes 2 (previously known), 14, 17 and 19 were found. A similar genome-wide scan in the Beaver Dam Eye Study identified two previously unidentified genetic loci for open-angle glaucoma on chromosome 6 and one on 13. The exact meaning of these findings remains to be elucidated at this time, but, obviously, multiple genes (as well as some environmental factors) seem to contribute to increased susceptibility to open-angle glaucoma.
Using a candidate gene approach, several potential POAG susceptibility genes have been identified. The renin–angiotensin system is present in the ciliary body and may play some role in the regulation of aqueous humor production. Polymorphisms in the angiotensin II receptor gene were found in Japanese POAG patients and some normal-tension glaucoma patients; those with the polymorphisms in the AGTR2 gene were more likely to have glaucoma and were likely to have worse visual fields than those without the polymorphism. On the other hand, polymorphisms in the gene for angiotensin converting enzyme were not associated with glaucoma in an English population. TAP1 and TAP2 genes play a role in the immunologic system; polymorphisms in these genes may be a risk factor for glaucoma. Excess synthesis of fibronectin has been found in tissue cultures of trabecular meshwork cells from patients with POAG. This opens a pathway of study to see if the excess fibronectin may play a role in reducing outflow facility in the glaucoma eye.
Sometimes, a promising pathway based on a sound hypothesis leads to a blind alley (as in any kind of medical research.) An example of this was the hypothesis that a mutation in apolipoprotein E, which can be found in several neurodegenerative disorders, might play a role in glaucoma which can also be considered a neurodegenerative disorder. The interest in this hypothesis was sparked by a report from Tasmania of an increased prevalence of normal-tension glaucoma in carriers of the epsilon4 apolipoprotein allele. However, subsequent studies elsewhere failed to confirm this and polymorphisms in apolipoprotein E could not be found in patients with either open-angle or normal-tension glaucoma. Another example is with pigmentary glaucoma. Recently, a DBA/2J mouse was found with iris atrophy and pigmentary dispersion. The mutation responsible for this appeared to be in the TYRP1 gene. Unfortunately, no mutations in the TYRP1 gene were found in humans with pigmentary glaucoma. On the other hand, at least in four families with pigmentary dispersion syndrome and pigmentary glaucoma, a mutation in a gene mapped to chromosome 7 (7q35-q36) has been found.
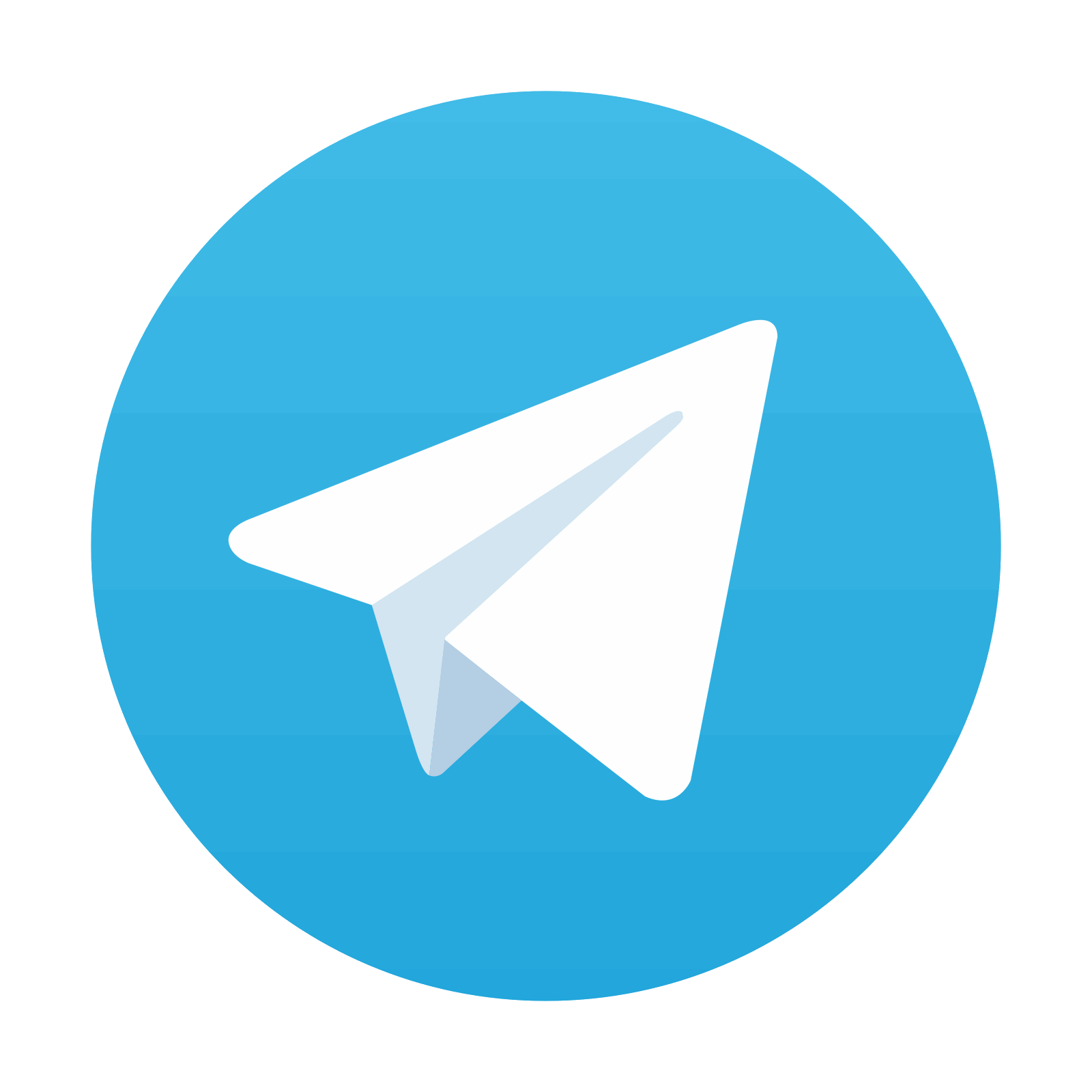
Stay updated, free articles. Join our Telegram channel

Full access? Get Clinical Tree
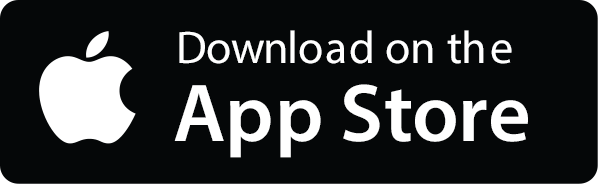
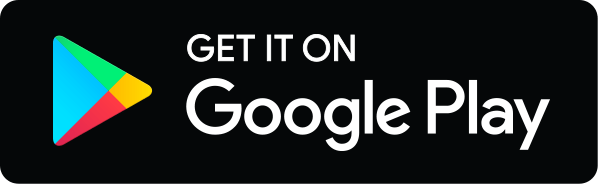