Ear, nose, and throat (ENT) medicine currently has several different systems at its disposal for minimally invasive treatments that apply high-frequency electric current to achieve a therapeutic effect. This chapter is intended to provide a survey of the technical fundamentals and tissue effects of such systems.
With the purpose of deepening the understanding of the use of high-frequency current for medical applications, an overview of the basics of high-frequency surgery is included in the second section.
1
System Overview
The therapy systems currently available on the market are distinguished in terms of their types of application, their therapy effects, and the technology of the equipment used.
1.1
Type of Application
Four types of applications of high-frequency energy can be distinguished:
- •
Submucous coagulation
- •
Superficial vaporization
- •
Submucous vaporization
- •
Electrotomy (electroexcision)
1.1.1
Submucous Coagulation
In the process of submucous coagulation, needle-shaped electrodes puncture the surface of the organ and are subsequently positioned inside it ( Fig. 42.1 ). When energy is applied, thermally induced coagulation builds up around the electrode, usually of ellipsoid shape, depending on the construction of the electrode. If positioning and energy dose are correct, the organ’s surface is conserved and the application is almost entirely free of pain. The body’s own decomposition and discharge of the necrotic tissue leads to a reduction in volume in the region treated.

1.1.2
Superficial Vaporization
The reduction in size of an organ (e.g. a tonsil) may also be accomplished by means of direct tissue ablation via vaporization (tissue vaporization) on the surface ( Fig. 42.2 ).

1.1.3
Submucous Vaporization
In the process of submucous vaporization, so-called channeling, channels are bored into the affected organ. For this purpose, a special bipolar electrode is necessary, at the tip of which a plasma ignition process takes place. When the tip comes into contact with tissue, the latter vaporizes immediately. A channel is generated by pushing the electrode forward in the tissue. More details on so-called plasma applications are to be found in the section “Plasma” Surgery.
1.1.4
Electrotomy
Electrotomy is also related to superficial vaporization methods. Contrary to plane vaporization at the surface, however, cuts are generated using fine needle–shaped electrodes with the objective of separating tissue ( Fig. 42.3 ).

1.2
Therapy Effect
Various therapy effects are derived from the four types of applications mentioned earlier: the delayed reduction in volume caused by the body’s own discharge of thermonecrotic tissue, the stiffening and tightening of a region of tissue by scar formation, and the removal of layers of tissue by superficial vaporization and the resection of parts of organs (e.g. uvula or tonsils) using electrotomy.
1.2.1
Volume Shrinkage by the Body’s Own Discharge of Coagulation Necrosis/Tissue Stabilization by Scar Formation After Coagulation Necrosis
Submucous coagulation shows two time-delayed effects: volume shrinkage caused by the body’s own discharge of the coagulated tissue and stabilization of a region of tissue by the scarring process.
After thermocoagulation, several phases take effect in the volume of tissue involved.
- •
Tissue coagulation becomes apparent immediately after its production by a lighter color resulting from the induced protein denaturation.
- •
After 1 day, a clearly defined lesion is evident, bordered by a hyperemic edge surrounding the destroyed tissue.
- •
About 10 days after treatment, the lesion is surrounded with connective tissue.
- •
After approximately 3 weeks, the dead muscle tissue has been replaced completely by connective tissue (collagen deposits). The scarring process is complete; the region of tissue involved has decreased in size and hardened.
1.2.2
Tissue Ablation by Means of Vaporization
Superficial ablation of tissue at the surface is used, for example, in tonsillotomy to achieve the partial resection of hyperplastic tonsils. In this case, the tissue is removed by vaporization in layers using a special arrangement of electrodes.
1.2.3
Tissue Resection by Means of Electrotomy
In the process of electrotomy, a high current density is generated at the point where the tissue is touched by means of electrodes of small surface area in the form of needles or slings. The tissue is heated to well above 100°C in a very short period, leading to vaporization of the cell fluid and bursting of the cells concerned. If the electrode is moved through the tissue, a cut is the result. Depending on the cutting power and speed of the cut, a more or less deep coagulation seam is produced at the edges of the cut, with the result that bleeding is reduced or can be avoided.
1.3
Equipment Technology
Radiofrequency systems can be fundamentally divided into monopolar and bipolar systems with regard to the equipment or applicator technology used. Further subdivision relates to the possibilities of therapy monitoring and power regulation.
1.3.1
Monopolar and Bipolar Application Technology
Monopolar Technology
Monopolar technology, where one of the two electrodes required to close the circuit is connected to the patient as a large-surface return path, is most commonly used in radiofrequency surgical applications to date. The actual working or active electrode is in the shape of a small-surface surgical instrument, for example, in the form of a needle, a lancet, or a ball. It is from the latter electrode that the radiofrequency alternating current from the generator is conducted into the patient, producing the desired surgical effect as a result of high current density at the point where the tissue is touched. The radiofrequency current disperses rapidly in the tissue and flows with lower current density through the body of the patient to the neutral electrode, whence it flows back to the generator ( Fig. 42.4 ).

A considerable number of complications may arise from the use of radiofrequency surgery, which are excluded when bipolar technology is applied.
Problem: Neutral Electrode.
Attachment of a neutral electrode to the patient is necessary when monopolar technology is used. In this context, attention must be paid to the certainty of low-contact resistance. The current density has to be evenly distributed over the entire surface of the neutral electrode. Perspiration, hairs, or fatty substances between the skin and the electrode surface may mean the current is unable to utilize the entire transfer surface, leading to current densities that are too high in certain places and that may in turn lead to burns.
Problem: Current Flow Through the Body Between Active and Neutral Electrode.
Thermal tissue damage may occur in parts of the body between the active and neutral electrodes where the cross-section available for current flow is small and electrical resistance high. The current flow is particularly problematic in the region of the head and neck: the greater part of the volume concerned here consists of bone as well as air-filled nasal and oral cavities or paranasal sinuses. In certain places only the thin mucous membrane layer remains as a conducting residual cross-section.
The application of monopolar high-frequency technology for patients with cardiac pacemakers or catheters may only be carried out in exceptional cases. The specialist literature contains different documented opinions and research results on the interaction between radiofrequency current and pacemakers.
Bipolar Technology
Although bipolar technology has been known for some considerable time, it was only in the mid-1980s that renewed efforts were made to press ahead and further develop bipolar radiofrequency technology for reasons of safety, both for coagulation and cutting purposes.
Bipolar application technology ( Fig. 42.5 ) is characterized by the fact that both electrodes, integrated in an application handset, are brought as close together as possible. The current only passes immediately between the two electrodes, meaning that secondary thermal damage to the patient, both internal and external, caused by leakage currents or marked changes in impedance (cross-sections with a high percentage of bone or fat and poorly conducting residual cross-sections) can be avoided. Because the attachment of a neutral electrode is not required in bipolar radiofrequency surgery and the current flow is restricted to the point of surgical intervention, the risks involved in monopolar technology as described earlier can principally be avoided ( Fig. 42.6 ).


1.3.2
Process Monitoring and Power Regulation
Superficial coagulation (e.g. using bipolar forceps to stop bleeding) can be directly monitored by the eye of the attending physician. Where submucous coagulation is concerned, however, the physician no longer has this possibility because no visual contact exists with the coagulation taking place underneath the tissue surface. In such a case, technical monitoring of therapy-relevant parameters via the power unit is all the more important.
The size of a submucous coagulation ( Fig. 42.7 ) depends on numerous parameters. Worth mentioning in this case are the electrode geometry, the power, and the application time. Tissue resistance, tissue temperature, or the energy input provide information on the tissue changes achieved.

Tissue Resistance Measurement
Tissue change can be determined by measuring the electrical resistance of the tissue (impedance). Protein denaturing during the coagulation process results in destruction of the cell membranes, which in turn induces an improvement of the electrical conductivity. The drying out process on reaching the boiling point, on the other hand, reduces the electrical conductivity of the tissue. These processes make it possible to monitor the coagulation process from start to finish. Tissue resistance provides direct information on the tissue change itself, in contrast to the temperature or energy input—physical parameters that induce the said tissue change.
Temperature Measurement
Tissue destruction is dependent on the tissue temperature and the time the tissue was exposed to this temperature (dose). Measurement of the tissue temperature permits an indirect assessment of the tissue destruction for this reason.
Determination of Energy Input
A correlation exists between energy input and coagulation volume in the process of submucous tissue coagulation. If a certain quantity of electrical energy is introduced into the tissue under treatment using a particular electrode geometry, a reproducible coagulation volume is the result. This only applies, however, as long as the marginal conditions of the coagulation process do not change. A change in the electrode position (distance from tissue surface or from larger vessels), in the temperature of the tissue, or in the blood perfusion rate has the result that a different coagulation volume is produced from a certain quantity of energy.
Measurement of the Application Time
If no possibility exists in a radiofrequency therapy system for the measurement of parameters accompanying the coagulation process, such as tissue impedance, temperature, or energy input, makeshift help may be provided by estimating the expected size of coagulation using time measurement. For this purpose, reference is made to experimentally determined data. A power level is selected and the spread of coagulation determined after certain time intervals for certain types of tissue. Where clinical applications are concerned, it is assumed that a particular combination of power and application time will always achieve the same effect in the tissue. Changes in the position of the electrodes affecting the coagulation result, tissue temperature, blood perfusion rate, and changes in performance associated with tissue impedance cannot be taken into account with this procedure.
Power Regulation
An optimal coagulation result can be achieved where radiofrequency power is adapted to the current condition of the tissue during the coagulation process. This way, overdosing or even carbonization can be avoided because a power input best suited to the desired result of the treatment is supplied as a function of each moment in time.
1.4
Therapy Systems Currently on the Market
1.4.1
Celon AG Medical Instruments
Application: submucous coagulation and electrotomy.
Technology: bipolar.
Power control therapy monitoring: impedance-dependent power regulation, automatic end of therapy on reaching target impedance, acoustic control of treatment status.
Other features: axial “in-line” electrode arrangement for submucous coagulation.
1.4.2
Gyrus ENT (Somnus)
Application: submucous coagulation.
Technology: monopolar.
Power control therapy monitoring: temperature-dependent power regulation, end of therapy on reaching targeted energy, optical control of temperature and energy input.
1.4.3
Coblator (Arthrocare)
Application: submucous vaporization (“channeling”)/coagulation and superficial vaporization.
Technology: bipolar.
Power control therapy monitoring: not present (manual time measurement).
Other features: procedures based on so-called plasma technology (sparking at the tip of the applicator caused by very high voltages).
1.4.4
Diverse Radiofrequency Surgical Equipment
Application: coagulation and cutting (excision).
Technology: monopolar, bipolar.
Power control therapy monitoring: not present (mainly manual time measurement).
Other features : conventional radiofrequency surgical equipment. Different working frequencies through to 4 MHz; direct contact between neutral electrode and tissue is unnecessary at this high frequency. The high-frequency current is also able to flow over so-called capacitive stretches (e.g. thin layers of air or clothing).
2
Basics of Radiofrequency Surgery
Reidenbach has defined radiofrequency surgery as follows : “radiofrequency surgery is understood as the application of radiofrequency energy for the purpose of changing or destroying tissue cells and of cutting through or removing tissue in combination with mechanical operation techniques.”
2.1
Historical Retrospective
The history of the development of radiofrequency surgery goes back as far as Hippocrates, a Greek physician of antiquity, who used a red-hot iron, the so-called ferrum candens, to arrest the flow of blood (hemostasis) during amputations around 400 bc .
In the middle of the 19th century, the so-called Paquelin burner was developed as a thermocauter (instrument for thermocautery) and the so-called galvanic cauter as an electrocauter. The first of these consisted of a metal pin heated to over 1000°C by a fuel/air mixture and used for the destruction of tumorous tissue. The electrocauter, on the other hand, made it possible to separate or slough off biological tissue by means of a knife or platinum sling raised to red-hot heat by direct current. Before the introduction of high-frequency technology at the beginning of the 20th century, such “cauterization” was the method usually applied in the field of surgery.
In 1891, the French physicist d’Arsonval reported on thermal effects induced in biological tissue by using alternating current at frequencies above 250 kHz without stimulation of muscles or nerves (so-called Faradic effect ). At the beginning of the 20th century, needle electrodes, which were inserted into the tissue (so-called electrodesiccation ), were used by Clark for the first time. Between 1907 and 1910, “coagulation” was developed as a method for tissue destruction, particularly against cancer, by Doyen, Czerny, and Nagelschmidt. In 1929, radiofrequency technology found its way into brain surgery with the development of suitable tube generators by Gulke and Heymann. At this time, a physicist, Bovie, and a surgeon, Cushing, were mainly responsible for the development of one of the first radiofrequency generators for the cutting and coagulation of tissue.
2.2
Physical and Technical Basics
Biological tissue comprises a system of different electrolytes, represented as different salt solutions in intracellular and extracellular space. These ions are responsible for electron transport and so for transcellular current flow in tissue. The cell membranes separate the electrolytes from each other, leading to their inhomogeneous distribution in the tissue. As a result of these dissociated salt solutions, depending on their individual concentrations, biological tissue becomes more or less electrically conductive. Where current flows through living biological tissue, three fundamental effects can be described: the Faradic effect, the electrolytic effect, and the thermal effect.
2.2.1
Faradic Effect
The Faradic effect is apparent using alternating currents of lower and middle frequency between 10 Hz and 10 kHz, which induce motor stimulation of muscle and nerve cells. Fig. 42.8 shows the stimulation threshold curve where current is plotted against frequency. It can be seen that the threshold current possesses a minimum between 50 and 100 Hz, meaning that very low currents are enough to cause serious damage to humans within the range of the usual power frequencies. At 50 Hz, even small currents may cause ventricular fibrillation.

Nernst has defined the “physiological stimulation” of alternating currents as a function of the electric current I and the frequency f with the equation:
Phys . stim . = I f
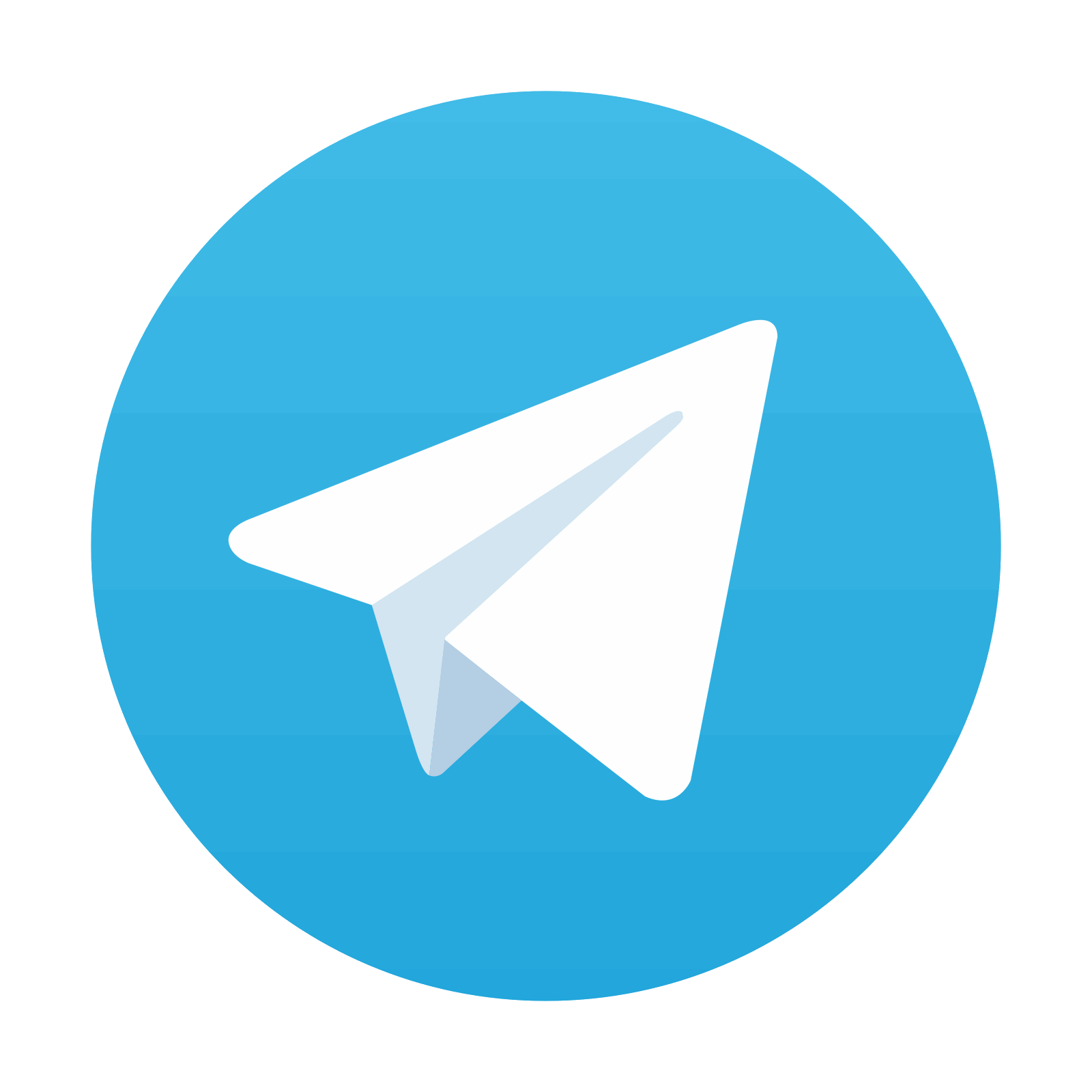
Stay updated, free articles. Join our Telegram channel

Full access? Get Clinical Tree
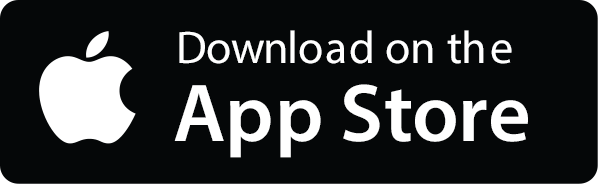
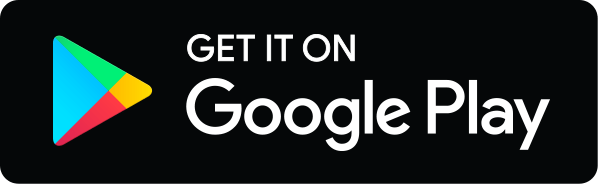