Introduction
The cranium protects the brain and provides scaffolding for facial structures. During primate evolution, the orbits were enlarged and reoriented towards the front of the face. This, along with the gradual flattening of the face, allowed for improved binocular vision due to overlapping visual fields. Along with morphologic skull changes, facial mimetic musculature evolved in primates as a means of close-proximity non-vocal communication. It is in this context that the function of the eyelids to protect, lubricate, and cleanse the ocular surface, is most fully appreciated. This chapter will examine anatomy of the eyelids, orbits, and related facial structures.
Orbital anatomy and function
Orbit osteology
Derived from cranial neural crest cells, the bony orbit consists of seven individual bones combining into four walls that surround the globe, extraocular muscles, nerves, fat, and blood vessels. The orbital bones include the sphenoid (greater and lesser wings), frontal, ethmoid, maxillary, zygomatic, palatine, and lacrimal bones. Posteriorly approaching the apex, this four-sided pyramid becomes three-sided with the gradual merger of the medial wall and orbital floor after the floor is cut off by the inferior orbital fissure. The adult orbit has a volume of 25–30 mL, with the globe filling approximately 7 mL or 25% of the space. The orbit depth as measured from the center of the orbital margin to the apex is approximately 45 mm. The widest diameter of the orbit occurs 1 cm behind the orbital rim. The lateral walls of the orbit are oriented 90 degrees from one another and run approximately 40–45 mm, while the medial walls are parallel to one another ( Fig. 14.1 ). Due to this bony orientation, the eyes tend to diverge, and thus are tonically held in adduction by the medial rectus muscles to achieve ocular alignment.

The orbital rim provides a hard tissue shield to surround and protect the eye. It is a thick discontinuous spiral that begins medially at the anterior lacrimal (maxillary bone) crest and coils to end at the posterior lacrimal (lacrimal bone) crest ( Fig. 14.2 ). The lacrimal fossa, which contains the lacrimal excretory sac, lies between these two crests. Formed by the zygomatic bone and the zygomatic process of the frontal bone, the lateral orbital rim is the strongest and thickest portion of the rim. While the posteriorly directed concavity of the lateral orbital rim allows for a wide visual field, it also makes the eye prone to injury from objects approaching from a lateral direction. The superior orbital rim is formed by the frontal bone. Medially, the superior rim contains the supraorbital notch through which the supraorbital nerve and artery pass. In some individuals, a supraorbital foramen replaces the notch. The infraorbital nerve and artery exit through the infraorbital foramen approximately 4–6 mm below the inferior orbital rim medially.

The orbital roof is comprised of the orbital plate of the frontal bone along with a minor contribution from the lesser wing of the sphenoid posteriorly. The frontal bone is the strongest component of the craniofacial skeleton, withstanding between 800 and 2200 pounds of force before fracturing. This is equivalent to the force achieved in a frontal collision at 30 mph for an unrestrained adult passenger. Orbital roof “blow-in” fractures may be associated with a number of ocular and neurologic injuries including proptosis, ptosis, optic nerve contusion, orbital hematoma, as well as contusive and hemorrhagic injuries to the ipsilateral frontal and parietal lobes. Important bony landmarks of the orbital roof include the lacrimal gland fossa temporally and another small depression 3–5 mm behind the orbital rim anteromedially known as the trochlear fossa. Here resides the fibrocartilaginous trochlea through which the superior oblique tendon passes.
The frontal sinus begins to develop between 1 and 2 years of age through invagination of the frontal bone by frontoethmoidal air cells. The filling of air or pneumatization of the sinus begins at about 5–8 years old and continues into adulthood. Therefore, there is a relative absence of frontal sinusitis in very young children. Yet, orbital complications due to frontal sinusitis such as orbital cellulitis and abscess most often occur between the ages of 5 and 10 years. It has been hypothesized that direct extension through the relatively thin orbital roof in children or congenital bony dehiscences (gaps) posterior to the trochlear and supraorbital notch may account for these findings.
The lateral wall is comprised of the zygomatic bone anteriorly and the greater wing of the sphenoid posteriorly. The vertically oriented zygomaticosphenoidal suture represents the thinnest portion of the lateral wall, and is a convenient breaking point during lateral orbitotomy. The posterior boundaries of the wall are marked by the superior and inferior orbital fissures. The frontosphenoidal suture forms the boundary between the lateral wall and orbital roof. About one-third of individuals have a meningeal foramen just superior to this suture which transmits the recurrent meningeal artery (a branch of the external carotid system) to form an anastomosis with the lacrimal artery (a branch of the internal carotid system). This collateral system has potential importance if the primary internal carotid vascular supply becomes compromised. An important bony prominence of the lateral wall is Whitnall’s lateral tubercle, a small rounded protuberance of the zygomatic bone 3–4 mm inside of the lateral orbital wall and approximately 11 mm below the frontozygomatic suture. ,
Fractures to the lateral wall may result from blunt trauma to the zygomatic bone and lateral orbital rim. Due to its multiple bony articulations, fractures of the zygoma may disrupt the wider anatomy and are often referred to as zygomaticomaxillary complex fractures or ZMC fractures ( Fig. 14.3 ). Clinical signs include lateral canthal dystopia (inferior displacement of the lateral canthal angle), cheek depression and trismus (difficulty opening the mouth owing to spasm of the masticatory muscles or impingement of the coronoid process of the mandible). Hypoesthesia may be noted at the lateral midface from disrupted branches of the zygomaticotemporal and zygomaticofacial neurovascular bundles (V1 branches traveling through the zygomatic bone), as well as in the distribution of the infraorbital nerve (V2 branch).

The orbital floor consists of the maxillary bone, the zygoma anterolaterally, and the palatine bone posteriorly. It is triangular in shape and extends from the maxillary-ethmoid buttress (the relatively dense bone strut at the union of the orbital floor and medial wall) to the inferior orbital fissure. The shortest of the walls, it travels posteriorly from the rim 35–40 mm and ends before the orbital apex at the pterygopalatine fossa. The infraorbital neurovascular bundle passes in the infraorbital groove and infraorbital canal of the orbital floor as it travels towards infraorbital foramen. The floor remains strong laterally, but thins posteromedially with expansion of the maxillary sinus. This thinned maxillary bone is where the floor usually fractures during trauma and is also a convenient site to initiate an inferior wall decompression. The infraorbital nerve is often contused during an orbital floor fracture but is rarely severed, thus, initial hypoesthesia in the V2 distribution will commonly resolve over several months’ time.
The medial wall consists of the maxillary, lacrimal and ethmoid bones, as well as the lesser wing of the sphenoid bone. The two medial walls are parallel to one another and extend 45–50 mm from the anterior lacrimal crest to the orbital apex. The lamina papyracea of the ethmoid bone is an extremely thin portion of bone that has a honeycombed structure. Giving support to the medial wall, the multiple bullae that form this structure develop secondarily to pneumatization of the ethmoid bone. This helps to explain why the medial wall fractures less often than the thicker orbital floor. Landmarks important to the orbital surgeon include the anterior and posterior ethmoidal foramina that reside at the frontoethmoidal suture and convey respective branches of the ophthalmic artery and nasociliary nerve. The anterior ethmoidal foramen is located approximately 24 mm posterior to the anterior lacrimal crest and the posterior ethmoidal foramen 36 mm posterior to the rim. The orbital foramen is located approximately 6 mm behind the posterior ethmoidal foramen. The frontoethmoidal suture may also be used surgically to approximate the level of the floor of the anterior cranial fossa.
Providing support for the inferomedial wall and helping to maintain globe position is the thickened region of bone known as the inferomedial strut. The strut is constructed using elements of multiple orbital and facial bones including the maxillary bone, ethmoid bone, and palatine bone posteriorly. , Preservation of the anterior portion of the inferomedial strut during orbital decompression has been shown to reduce the incidence of post-operative globe dystopia.
As previously mentioned, the lacrimal sac fossa lies between the anterior (maxillary bone) crest and posterior (lacrimal bone) crest of the orbital rim ( Fig. 14.4 ). The relative contribution of these two bones may vary. The lacrimal bone at the lacrimal sac fossa has a mean thickness of 106 µm, which allows easy penetration during dacryocystorhinostomy surgery (surgical procedure to restore the normal flow of tears into the nose from the lacrimal sac by bypassing the nasolacrimal duct). The maxillary bone is considerably denser than the lacrimal bone, and may require the surgeon to create a more posterior osteotomy during dacryocystorhinostomy. The nasolacrimal canal directs the nasolacrimal duct to the inferior meatus of the nose under the inferior turbinate.

The orbital apex
Many important neural and vascular structures pass through the orbit apex including cranial nerves II through VI, the origins of all the extraocular muscles except the inferior oblique, and arterial and venous blood supplies ( Fig. 14.5 and Box 14.1 ). Pathology in this crucial location of the orbit may lead to the orbital apex syndrome with characteristic hallmarks of visual loss from optic neuropathy and ophthalmoplegia.

- •
Passing through the optic foramen: optic nerve, ophthalmic artery, and sympathetic fibers
- •
Passing through the superior orbital fissure: oculomotor nerve, trochlear nerve, abducens nerve, the ophthalmic division of the trigeminal nerve (frontalis, lacrimal, and nasociliary nerves), sympathetic fibers, and the superior ophthalmic vein
- •
Passing through the annulus of Zinn: optic nerve, ophthalmic artery, oculomotor nerve, abducens nerve, nasociliary nerve, and sympathetic fibers
- •
Passing through the inferior orbital fissure: maxillary division of the trigeminal nerve, branches from the sphenopalatine ganglion, and the inferior ophthalmic vein
The optic foramen conveys the optic nerve and ophthalmic artery from the optic canal into the orbit. The canal is housed in the lesser wing of the sphenoid bone with a contribution from the inferomedial optic strut. It runs 8–10 mm in length and is 5–6 mm in diameter. The optic canal and foramen attain adult dimensions by 3 years and are symmetric in most persons. A canal/foramen that is larger in diameter by at least 1 mm than the contralateral side may be considered abnormal.
The superior orbital fissure is located just lateral to the optic canal. Approximately 20–22 mm in length, the fissure divides the lesser and greater wings of the sphenoid bone. The superior ophthalmic vein, as well as the lacrimal, trochlear, and frontal nerves, passes through the superolateral portion of the fissure outside the annulus of Zinn, a fibrous ring formed by the common origins of the rectus muscles. The annulus is further subdivided by the oculomotor foramen through which the superior and inferior divisions of the oculomotor nerve, the abducens nerve, the nasociliary nerve (a terminal sensory branch of the ophthalmic division of the trigeminal nerve), and sympathetic fibers all pass. , Also passing through the annulus of Zinn are the optic nerve and ophthalmic artery. As the extraocular rectus muscles proceed forward to their insertions on the globe, they form a conoid enclosure known as the intraconal space. This compartment is useful radiologically ( Fig. 14.6 ). Intraconal pathology of the fat, vessels, and optic nerve-sheath complex may be distinguished from extraconal pathology of the lacrimal gland, bony orbit, and remaining extraconal fat. ,

The inferior orbital fissure divides the greater wing of the sphenoid laterally from the maxillary bone of the orbital floor inferomedially. The fissure measures approximately 20 mm in length. It communicates with the pterygopalatine fossa which rests behind the maxillary sinus. Traveling through the fissure are the maxillary division of the trigeminal nerve, branches from the sphenopalatine ganglion, and the inferior ophthalmic vein. Branching away from the fissure and entering the infraorbital groove and canal are the infraorbital (V2) and the terminal branch of the internal maxillary artery.
Orbital soft tissues
Periorbital fascia
The periorbital fascia is a single interconnecting network emanating from the periosteal lining of the orbital walls, globe (Tenon’s capsule), and extraocular muscles ( Figs 14.7 & 14.8 ). There are also check ligament extensions from the extraocular muscle fascia that attach to the bony orbit as well as sheaths that extend between the rectus muscles. The periorbita is firmly attached at the suture lines, foramina, fissures, arcus marginalis, and the posterior lacrimal crest. Elsewhere, it is loosely attached to the bone which creates a potential space for accumulation of blood, pus, or tumor growth. It is most firmly attached along the arcus marginalis. The orbital septum is a thin, multilayered extension of the periorbita and is the anterior soft tissue boundary of the orbit. It functions as a physical barrier to pathogens and contributes to the normal posterior position of the orbital fat pads. In the upper eyelid, the fibrous lamellae of the orbital septum gradually blends with those of the levator aponeurosis on average 3.4 mm above the superior tarsal border (with a range of 2–5 mm). In the lower eyelid, the septum inserts onto the inferior border of the tarsus after joining with the inferior retractors 4–5 mm below the tarsus. Laterally the septum fuses with the lateral canthal tendon and attaches 2–3 mm posterior to the rim at the lateral orbital tubercle (Whitnall’s tubercle). Medially the septum splits and inserts onto the anterior and posterior lacrimal crest. Finally, it is anchored anteriorly to the orbicularis muscle by multiple fibrous attachments.


Tenon’s capsule, or fascia bulbi, is a fibroelastic membrane that extends anteriorly from the dural sheath to encircle the globe and fuse with the conjunctiva just behind the limbus. Posteriorly it separates orbital fat from the globe and muscles. Openings within the fascia allow passage of the extraocular muscles which are in turn surrounded by their own muscular fascia. Anteriorly the muscular fascia thickens and connects with the orbital wall to form the check ligament of the extraocular muscles, preventing overaction of the muscle from which they extend. The strongest of these is the lateral check ligament that inserts on the lateral orbital tubercle, while the medial rectus check ligament inserts behind the posterior lacrimal crest.
Orbital fat
Orbital fat provides cushioned support for the globe and other intraocular structures. Eyelid fat pads are the anterior projections of the orbital fat. Changes in orbital fat appear to have a significant role in the development of thyroid-associated orbitopathy (TAO). Orbital fat and extraocular muscle volume expansion may result in soft tissue swelling, proptosis, lid retraction (with characteristic temporal flare), strabismus (usually due to muscle fibrosis), and compressive optic neuropathy. , Fibroblasts residing within the orbital connective/adipose tissue are believed to be the targets of an autoimmune attack consisting of primarily T lymphocytes, and there is ongoing research to isolate key autoantibodies. Patients with a hyperthyroid state are not the only patients affected with TAO. Euthyroid patients may also develop TAO. There appears to be a shift in the phenotypes of peripheral blood lymphocytes in euthyroid patients with TAO when compared to normal controls which may permit an orbital immune reaction.
Orbital nerves
Entering the orbit are the optic (cranial nerve II), oculomotor (cranial nerve III), trochlear (cranial nerve IV), abducens (cranial nerve VI), the first and second divisions of the trigeminal (cranial nerve V), parasympathetic, and sympathetic nerves. The trigeminal nerve will be discussed in the eyelid portion of this chapter while the extraocular muscles are discussed in Chapter 7 .
Composed of approximately 1.2 million nerve fibers, the optic nerve is an extension of the central nervous system. It has supportive neuroglial cells and is surrounded by the three layers of the meninges: the dura mater, arachnoid layer, and pia mater. Cerebrospinal fluid flows freely within the space between the arachnoid and pia. The nerve arises from the ganglion cell layer of the retina. These axons converge at the optic nerve head which is approximately 1.5 mm in diameter. As the nerve exits the globe at the lamina cribrosa and enters the orbit, its diameter increases to 3.5 mm due to the addition of the myelin sheath. The intraorbital portion of the nerve runs approximately 25 mm, although the distance from the globe to the optic foramen is 18 mm. This extra slack allows freedom of movement of the globe and a certain degree of proptosis. When excessively stretched, the nerve may exert tension on the globe – seen radiologically as “globe tenting” – which may result in vision loss due to compromise of the nerves’ vascular supply. The intracanalicular portion of the nerve is tethered within the bone, making it susceptible to damage from blunt trauma. , The intracranial portion of the optic nerve runs approximately 15 mm just medial to the internal carotid artery ending at the optic chiasm.
Motor innervation of the orbit involves the oculomotor (cranial nerve III), trochlear (cranial nerve IV), and abducens nerves (cranial nerve VI) ( Fig. 14.9 ). The oculomotor nerve innervates the superior, inferior, and medial recti, the inferior oblique, and the levator palpebrae superioris muscles. Additionally, parasympathetic fibers travel within the inferior division of the nerve. The nerve exits the brainstem medially and travels through the superolateral portion of the cavernous sinus while dividing into a superior and inferior division. Both branches enter the orbit through the oculomotor foramen within the annulus of Zinn. The superior branch enters the underside of the superior rectus, on its way to innervate the overlying levator palpebrae superioris muscle. The inferior branch of the oculomotor nerve travels beneath the optic nerve and supplies innervation to the medial and inferior rectus, as well as the inferior oblique.

Studies in both monkeys and man have revealed that parasympathetic fibers, issued from cells of the pterygopalatine ganglion, pass into the orbit. Nilsson et al showed that parasympathetic fibers responsible for uveal vasodilation travel to the eye from the facial nerve via the pterygopalatine ganglion. As previously mentioned, a small bundle of parasympathetic fibers destined for the ciliary ganglion travel within the inferior division of the oculomotor nerve. Included within this parasympathetic bundle are pupillomotor fibers. During orbital floor repair, damage to these fibers may result in post-operative mydriasis.
The trochlear nerve is the only cranial nerve to exit dorsally from the midbrain; crossing the midline to emerge adjacent to the superior cerebellar peduncle. It travels within the wall of the cavernous sinus and enters the orbit via the superior orbital fissure outside of the annulus of Zinn. It is the only extraocular cranial nerve that travels extraconally within the orbit. It crosses medially over the origin of the superior rectus and levator palpebrae superioris, and enters the superior oblique muscle. The long intracranial course of the trochlear nerve makes it particularly vulnerable to closed head trauma.
The abducens nerve may exit between the pons and the medulla in one or multiple trunks. The nerve ascends through the subarachnoid space, then climbs along the bony clivus before passing through Dorello’s canal into the posterior cavernous sinus. Unlike the oculomotor and trochlear nerves, the abducens nerve is not located in the lateral wall of the cavernous sinus. Instead it travels within the body of the sinus with the internal carotid artery. It enters the orbit through the oculomotor foramen and inserts onto the inner surface of the lateral rectus at approximately one-third the distance from the muscle origin to its insertion.
Sympathetic fibers, originating in the superior cervical ganglion, travel along the internal carotid artery through the cavernous sinus, and into the orbit along the ophthalmic artery. Fibers have also been shown to travel with the abducens and ophthalmic (V1) division of the trigeminal nerve. While in the orbit, some of the sympathetic fibers pass through the ciliary ganglion without synapsing. Orbital sympathetics allow for pupillary dilation of the iris dilator muscle, function of the smooth muscle of the eyelid (Müller’s muscle), and vasoconstriction.
Vascular anatomy
Arterial supply
The arterial supply to the orbit is an anastomotic network with contributions from the internal and external carotid circulation. Most of the arterial supply of the eye and orbit is provided through the ophthalmic artery; the first intracranial branch of the internal carotid artery ( Fig. 14.10 ). The most important branch of the external carotid artery in regards to orbital supply is the internal maxillary artery.

The ophthalmic artery branches off from the internal carotid artery just before the latter artery exits the cavernous sinus. It travels with the optic nerve within the inferolateral portion of a common dural sheath, and gradually bends medially at which point branching of the artery occurs. , There is a great deal of variability in the order of branching vessels originating from the ophthalmic artery. In general, the first branch is the central retinal artery. Originating at the level of the orbital apex and eventually supplying the outer layers of the retina, the central retinal artery travels lateral or inferior to the optic nerve and penetrates the nerve at 5–16 mm posterior to the globe (average 10 mm). , Also arising near the medial bend of the ophthalmic artery are the lateral and medial posterior ciliary arteries. Constituted of typically two or three branches, these arteries give rise to 15–20 short posterior ciliary arteries as well as the two long posterior ciliary arteries. The short posterior ciliary arteries supply the optic nerve head and choroid, while the long posterior ciliary arteries travel within scleral canals anteriorly to supply the ciliary muscle, anterior choroid, and iris.
Other intraorbital structures supplied by the ophthalmic artery include the extraocular muscles and lacrimal gland. There are two main muscular branches which supply the extraocular muscles: the medial and lateral branches. The medial muscular branch supplies the medial rectus, inferior rectus, and inferior oblique muscles, while the lateral branch supplies the levator palpebrae superioris, superior rectus, superior oblique, and lateral rectus muscles. The vessels run along the muscle belly or medial surface of the muscle. Each rectus muscle artery branches into two anterior ciliary arteries, except for the lateral rectus muscular artery, which terminates into one anterior ciliary artery. The anterior ciliary arteries anastomose with the long posterior ciliary arteries to supply the globe. Simultaneous surgery on three rectus muscles or two muscles in patients with poor vascular supply may result in anterior segment ischemia, characterized by corneal edema and a mild anterior uveitis. The lacrimal artery travels superolaterally in the orbit to reach the posterior surface of the lacrimal gland. It also gives off the zygomaticotemporal, zygomaticofacial, and the lateral palpebral arterial branches. The latter branch supplies the lateral portion of the arcades of the upper and lower eyelids. Portions of the lacrimal artery anastomose with the middle meningeal artery through branches of the recurrent meningeal artery and temporal arteries.
The posterior and anterior ethmoidal arteries course medially from the ophthalmic artery. While traveling at the level of the frontoethmoidal suture, they exit the orbit through the posterior ethmoid foramen and anterior ethmoid foramen respectively. They supply the ethmoid and frontal sinus mucosa as well as the frontal dura.
The supraorbital artery comes off from a more distal portion of the ophthalmic, exits through the supraorbital foramen or notch, and supplies mainly the eyebrow, forehead, and the medial portion of the eyelids. The anterior continuation of the ophthalmic artery is the nasofrontal artery. Just posterior to the trochlea, the nasofrontal artery divides into the supratrochlear artery which supplies the medial forehead and scalp, and the dorsal nasal artery. The dorsal nasal artery and its branches, the medial palpebral arteries, supply the medial eyelids and nose.
The two terminal branches of the external carotid artery are the maxillary and superficial temporal arteries. The maxillary artery branches off from the external carotid artery just behind the neck of the mandible an average of 25.7 mm (range, 24.86–27.47 mm) below the condyle. It passes deep to the mandible and enters the pterygopalatine fossa through the infratemporal fossa. Among its branches are the middle meningeal and infraorbital arteries. The middle meningeal artery, which has been previously discussed, may anastomose with the lacrimal artery via the recurrent meningeal artery. Rarely, it may provide the majority of orbital blood in patients with ipsilateral carotid stenosis or congenital atresia (lack of patency) of the ophthalmic artery. Branches from the infraorbital artery supply the inferior rectus and inferior oblique muscles, the lacrimal sac, orbital fat, upper cheek, and lower eyelid. The superficial temporal artery supplies the forehead and may anastomose with the supraorbital and supratrochlear arteries of the internal carotid circulation.
Venous drainage
Venous drainage of the orbit consists of a valveless anastomotic system with multiple tributaries that drain anteriorly to the facial veins or posteriorly into the cavernous sinus or pterygoid plexus. The major venous branches of the orbit are the superior and inferior ophthalmic veins. The superior ophthalmic vein is formed at the confluence of the angular, supraorbital, and supratrochlear veins. It travels posteriorly along the roof of the orbit, before diving into the muscle cone where it picks up venous drainage from the globe through the superior vortex and ciliary veins. The superior ophthalmic vein then drains into the cavernous sinus after passing through the superior orbital fissure. The inferior orbital vein is responsible for venous drainage of the inferior orbit. It collects venous blood from the inferior extraocular muscles and inferior vortex veins, and drains into both the cavernous sinus and pterygoid plexus. Some of the venous blood from the surrounding periorbita drains through a system of anterior facial veins. These typically drain directly into the external jugular vein.
Orbital lymphatic drainage
The goal to identify the orbital lymphatic drainage system has been pursued in both human and animal studies. Traditionally thought to be absent in the orbit, the more recent use of selective lymphatic markers has identified lymphatics in at least some areas of the human orbit. , Using CD-34 and D2-40 monoclonal antibodies, specific for blood vessel endothelium and a protein on lymphatic endothelium respectively, lymphatics were confirmed in human lacrimal gland and optic nerve dura. Further studies should help to clarify and expand these findings.
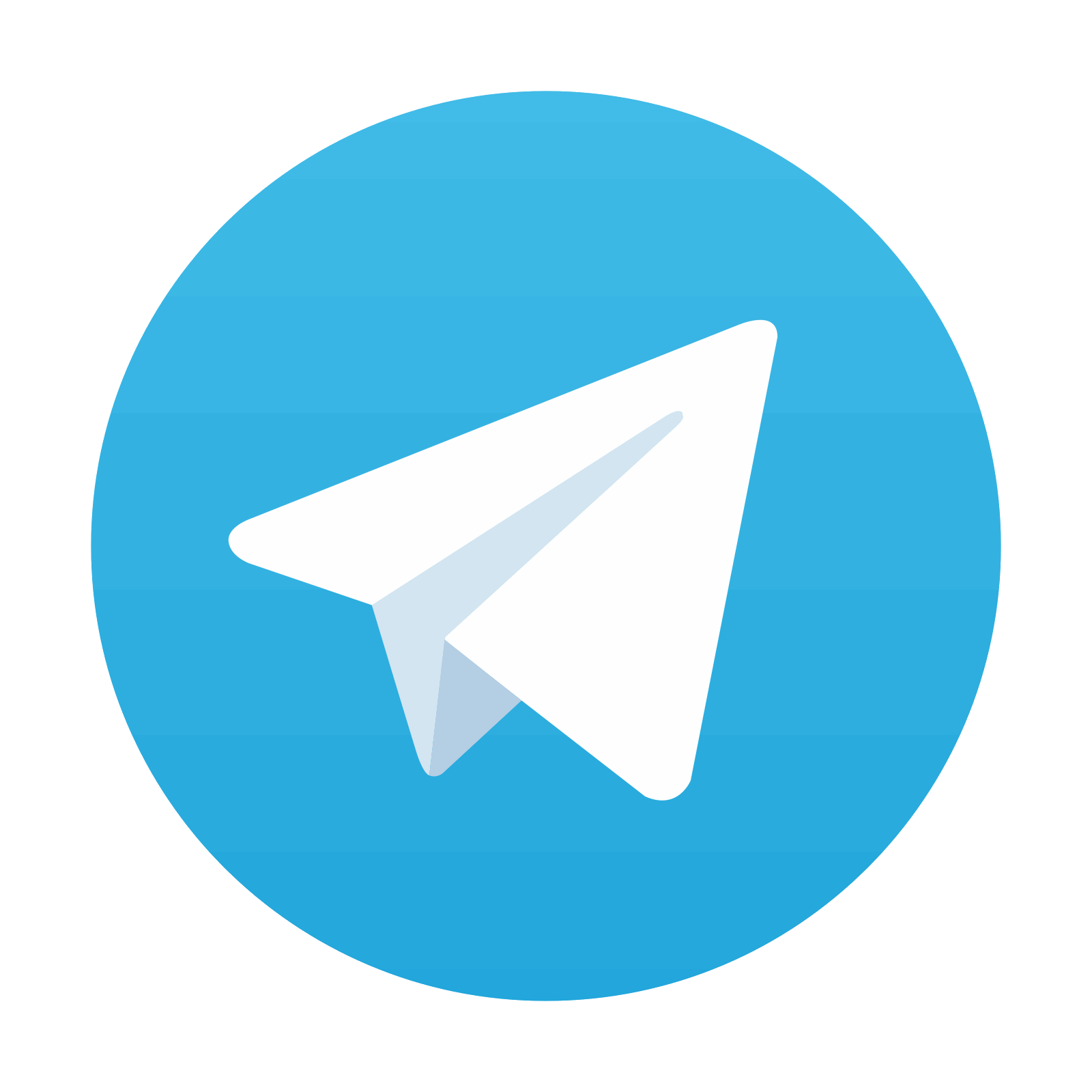
Stay updated, free articles. Join our Telegram channel

Full access? Get Clinical Tree
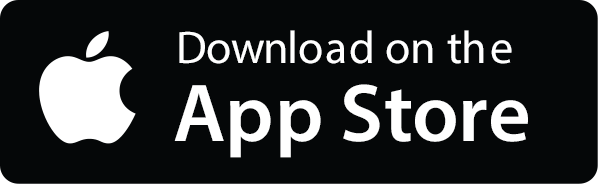
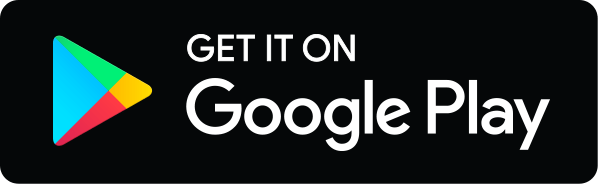