This chapter covers the diagnosis and management of the causes of double vision and ocular misalignment. Although the bulk of the discussion concentrates on third, fourth, and sixth nerve palsies, other supranuclear, nuclear, neuromuscular junction, myopathic, and restrictive disorders are detailed or mentioned. The relevant anatomy of the ocular motor system is discussed first, followed by signs and symptoms in patients with diplopia, then the diagnosis and management. The differential diagnosis of the major entities that cause diplopia are then reviewed.
Several terms are used interchangeably throughout this chapter: oculomotor, third, and IIIrd nerve; trochlear, fourth, and IVth nerve; and abducens, sixth, and VIth nerve. Also, the term ocular motor nerves refers to the IIIrd, IVth, and VIth nerves together.
Anatomy
Overview
The three ocular motor cranial nerves (IIIrd, IVth, and VIth) innervate the six extraocular muscles of each eye ( Fig. 15.1 ), the major eyelid elevator, and the pupillary constrictor. The oculomotor (IIIrd) nerve activates the medial rectus (adduction), inferior rectus (depression), superior rectus (elevation), and inferior oblique (excyclotorsion and elevation) muscles as well as the pupillary sphincter muscle (constriction) and levator palpebrae of the upper lid. The trochlear (IVth) nerve supplies the superior oblique muscle, which intorts the eye and depresses it in adduction. The abducens (VIth) nerve innervates the lateral rectus muscle, which abducts the eye ( Fig. 15.2 ).


From Central Nervous System to the Orbit
Supranuclear control of III, IV, and VI . In a logical, hierarchical control of eye movements, the supranuclear centers in the cortex and brainstem direct the actions of the ocular motor nerves. For horizontal saccades (see Fig. 16.2 ), the frontal and cortical eye fields inhibit the contralateral omnipause neurons (OPN), thereby disinhibiting the paramedian pontine reticular formation (PPRF). Neurons of the PPRF, in turn, excite the adjacent VIth nerve nucleus, which, via the ascending medial longitudinal fasciculus (MLF), innervates the contralateral medial rectus subnucleus in the mesencephalon. Supranuclear centers for pursuit lie in the ipsilateral frontal and supplementary eye fields and V5 (see Fig. 16.3 ). Upgaze and downgaze saccades, also under voluntary control by the frontal and cortical eye fields, are initiated by neurons in the mesencephalic rostral interstitial nucleus of the medial longitudinal fasciculus (riMLF) and regulated by cells in the interstitial nucleus of Cajal (inC), areas which exert supranuclear control over the IIIrd and IVth nerve nuclei (see Fig. 16.18 ). The vestibular nuclei have direct connections with the IIIrd and IVth nerve nuclei, via the MLF. These and other supranuclear gaze centers are reviewed in detail in Chapter 16 .
Nuclear and fascicular structures . The oculomotor (IIIrd) nuclear complex lies in the midbrain, anterior to the cerebral aqueduct ( Fig. 15.3 ). Each muscle innervated by the third nerve is subserved by individual subnuclei. Unique features, however, include the central caudal subnucleus, which subserves both levator muscles; the superior rectus subnuclei, which each innervate the contralateral superior rectus muscle; and the Edinger–Westphal nuclei, which supply the preganglionic cholinergic neurons of the pupil. Fibers from the superior rectus subnuclei are thought to pass through the contralateral superior rectus subnucleus before joining the contralateral IIIrd nerve fascicle. Pupillary and eyelid function and related abnormalities are discussed in Chapters 13 and 14 , respectively. The paired oculomotor fascicles travel ventrally through the midbrain, each passing through the red nuclei and then the medial portion of the cerebral peduncles before exiting into the interpeduncular fossa (see Fig. 15.3 ). The neurons of each IIIrd nerve–innervated muscle are topographically organized within the fascicle ( Fig. 15.4 ).


The trochlear nucleus lies ventral to the aqueduct in the pontomesencephalic junction ( Fig. 15.5 ), caudal to the oculomotor complex. Fourth nerve axons decussate near the roof of the aqueduct and exit the brainstem dorsally just beneath the inferior colliculi, in an area called the anterior medullary vellum. Thus each trochlear nucleus innervates the contralateral superior oblique muscle.

The abducens nucleus lies immediately ventral to the genu of the facial nerve (facial colliculus in dorsal pons) ( Fig. 15.6 ). This nucleus contains cell bodies for the motor neurons which will innervate the ipsilateral VIth nerve and interneurons which will ascend within the MLF to innervate the contralateral medial rectus subnucleus (see Fig. 16.2 ). The fascicles travel ventrally, passing through the corticospinal tracts, before exiting anterolaterally at the pontomedullary junction.

It should be emphasized that except for the superior rectus subnucleus and the trochlear nuclei, the ocular motor nuclei innervate ipsilateral eye muscles.
Extraaxial structures . The three ocular motor nerves traverse the subarachnoid space at the skull base before reaching the cavernous sinus ( Fig. 15.7 ). The IIIrd nerve enters the cavernous sinus by piercing the dura in its most superior portion just lateral to the posterior clinoid process. The IVth nerve travels for about 3.5 cm from behind the brainstem, running under the free edge of the tentorium before piercing the dura to lie immediately inferior to the third nerve. The VIth nerve ascends the clivus then travels underneath the petroclinoidal (Grüber’s) ligament through Dorello’s canal to enter the cavernous sinus. The transition from petrous to intracavernous segments of the internal carotid artery occurs as the vessel passes the petrolingual ligament, which is the posteroinferior attachment of the lateral wall of the cavernous sinus. Rarely, there are two roots of the abducens nerve which exit the pons, or the main trunk splits into two. In such cases the roots eventually fuse into a single nerve within the cavernous sinus.

The cavernous sinuses are triangular-shaped interconnecting structures that flank the lateral sides of the sella turcica. The walls of each sinus are created by dura mater extending from the superior orbital fissure to the posterior wall of the sella known as the dorsum sella. Inferiorly, the cavernous sinus region and pituitary fossa are in close proximity to the sphenoid and maxillary sinuses while the optic chiasm lies superomedially (see Fig. 7.5 ). Each cavernous sinus contains a plexus of veins that drains the inferior and superior ophthalmic veins of the orbit and funnels posteriorly to the inferior and superior petrosal sinuses. Communications are established to the opposite cavernous sinus by channels that cross the midline. Inferiorly, connections are made to the pterygoid plexus that subsequently drain into the internal jugular vein while lateral anastomoses are made to the middle cerebral vein.
The S-shaped intracavernous carotid artery gives rise to multiple important branches, including (1) the meningohypophyseal trunk that supplies blood to the tentorium and inferior pituitary gland, (2) the inferior lateral trunk that nourishes the ocular motor nerves, and (3) the superior hypophyseal artery that provides blood to the rostral portion of the pituitary gland.
The third and fourth nerves and the first and second divisions of the trigeminal nerve (V1 and V2) lie along the lateral wall of the cavernous sinus, while the internal carotid artery, the sixth nerve, and third-order oculosympathetic fibers from the superior cervical ganglion lie more medially ( Fig. 15.8 ). In the anterior portion of the cavernous sinus, the IIIrd nerve anatomically separates into superior and inferior divisions. The superior division innervates the superior rectus and levator muscles, while the inferior division supplies the inferior rectus, inferior oblique, medial rectus, and pupillary sphincter muscles. Within the anterior cavernous sinus, the IVth nerve crosses above the third nerve just before both nerves enter the superior orbital fissure. Within the cavernous sinus network, the VIth nerve lies freely infralateral to the cavernous carotid artery and enters the superior orbital fissure as it courses anteriorly. The postganglionic sympathetic fibers travel up the carotid artery and briefly join the VIth nerve within the cavernous sinus. This anatomical relationship accounts for the occasional case of an abducens palsy and Horner syndrome associated with lesions of the cavernous sinus.

Knowledge of the anatomy of the trigeminal nerve (see Chapter 19 ) is critical for localizing lesions within the parasellar region. Since the mandibular branch of the trigeminal nerve travels through the foramen ovale, involvement of this branch indicates a process posterior to the cavernous sinus. More anteriorly, the maxillary division leaves the skull base at the level of the cavernous sinus via the foramen rotundum. The ophthalmic branch of the trigeminal, along with the IIIrd, IVth, and VIth nerves and the oculosympathetic fibers, leave the anterior cavernous sinus, and enter the orbit through the superior orbital fissure ( Fig. 15.9 ; see also Fig. 15.1 ).

Orbit . Once in the orbit (see Fig. 15.1 ), the ocular motor nerves reach their respective extraocular muscles. The IIIrd and VIth nerves along with the optic nerve lie within the annulus of Zinn and muscle cone (see Fig. 15.9 ). Like the frontal and lacrimal nerves (branches of V1), the trochlear nerve passes above the annulus of Zinn. However, the orbital apex syndrome is usually considered to consist of optic, IIIrd, IVth, VIth, and V1 nerve dysfunction. In contrast to the more direct orientation and attachments of the lateral, inferior, and superior rectus and inferior oblique muscles, the superior oblique muscle tendon courses through a pulley attached to the superonasal orbital wall then attaches to the globe on its posterior and temporal aspect (see Fig. 15.1 ). This arrangement accounts for the down and in movement and intorsion of the eye produced by superior oblique contraction. Pupilloconstrictor fibers from the IIIrd nerve synapse in the ciliary ganglion, which lies within the lateral muscle cone (see Fig. 13.3 ) and issues postganglionic parasympathetic fibers that innervate the pupil. Other details regarding orbital anatomy, including that of the extraocular muscles, are discussed in Chapter 18 .
Blood supply of the extraaxial ocular motor nerves . The nutrient circulation to the IIIrd nerve arises from (1) small thalamomesencephalic perforators arising from the basilar and posterior cerebral arteries and the posterior circle of Willis, in the proximal subarachnoid space ; (2) as alluded to earlier, microscopic branches of the inferior lateral trunk of the intracavernous carotid within the cavernous sinus; and (3) recurrent branches of the ophthalmic artery intraorbitally. A watershed region of the IIIrd nerve may exist between the proximal and intracavernous portions. The cavernous and orbital blood supply of the IVth and VIth nerves is likely similar. The subarachnoid segment of the IVth nerve derives its blood supply from branches of the superior cerebellar artery. The subarachnoid segment of the VIth nerve is likely supplied by branches from the posterior cerebral and superior cerebellar arteries.
Symptoms
Patients with diplopia should be asked whether their double vision is (1) binocular; (2) horizontal or vertical; and (3) worse in left gaze, right gaze, upgaze, or downgaze, or distance or near.
- 1.
Binocular vs monocular double vision. This can be assessed by asking the patient, “Does the double vision go away when you cover either eye?” Neurologic ocular misalignment causes binocular diplopia which disappears with either eye covered, whereas ocular causes such as refractive error, for instance, lead to monocular double vision which persists in one eye with the other eye covered.
- 2.
Horizontal or vertical. Horizontal double vision suggests a misalignment caused by dysfunction of the medial or lateral rectus muscles. Vertical diplopia implies an abnormality of the vertically acting muscles such as the inferior rectus, superior rectus, inferior oblique, or superior oblique. Because the vertically acting muscles also tort the eye, their dysfunction can cause diplopia with tilted images.
- 3.
Directionality. In a patient with diplopia caused by extraocular muscle paralysis, the double vision will worsen when the patient attempts to look in the direction of action of the paretic muscle. For example, attempted leftward gaze worsens the horizontal diplopia due to weakness of the right medial rectus muscle. Thus, in some instances, history alone is often helpful in localizing the problem.
Some patients with ocular misalignment complain of blurry vision instead of frank diplopia, especially if the two images are close together or the person has intermittent fusion. Asking if the blurry vision resolves with one eye covered is helpful in this regard.
Individuals with ocular misalignment who have no diplopia usually have either (1) defective vision in one or both eyes due to refractive error, cataract, optic neuropathy, or maculopathy, for instance; (2) long-standing childhood strabismus with strabismic amblyopia (defined as loss of two Snellen lines of visual acuity in the bad eye compared with the good one) or suppression (the ability to ignore one of the images); (3) ptosis of one eyelid; or (4) a large angle of strabismus, making the second image less confusing and easy to ignore.
The examiner should investigate other historical details such as duration of onset, whether the patient has had the motility deficit since childhood; presence of pain; fatigability; other neurologic symptoms such as dysphagia or weakness; underlying illnesses such as hypertension, diabetes, cerebrovascular disease, cardiac atherosclerotic disease, or multiple sclerosis; and habits such as smoking or alcohol use.
Signs
The ocular motility examination is detailed in Chapter 2 . It should consist of testing for the following:
- 1.
Obvious misalignment, ptosis, or pupillary abnormalities, first by inspection.
- 2.
Ductions, which are movements of each eye separately.
- 3.
Vergences, which are coordinated movements of both eyes in opposite directions.
- 4.
Saccades and pursuit.
- 5.
Oculocephalic maneuver.
- 6.
Ocular misalignment, assessed qualitatively or quantitatively using cover–uncover, alternate cover, Maddox rod, or Krimsky or Hirschberg techniques. Often the motility disturbance will be obvious after inspection and testing of vergences. However, more subtle problems will require quantification of the misalignment using prism-alternative cover or Maddox rod techniques. Although there are many exceptions, acquired incomitant deviations (in which the ocular misalignment is different in different directions) are usually caused by a neurologic problem, while comitant deviations (same in every direction) are typically long-standing, often dating to childhood. Individuals with prior strabismus surgery may also have an incomitant deviation. Patients with shallow orbits may not bury their sclera fully in lateral gaze, giving the false impression that an abduction deficit exists. In such cases it is important to look for an esodeviation worse in lateral gaze which, if present, will indicate a true abduction deficit.
- 7.
Other clues such as head, face, and chin position (e.g., head tilt) or orbital signs such as proptosis or eyelid swelling.
- 8.
Forced ductions when restrictive abnormalities are suspected.
Monocular diplopia that resolves with a pinhole is likely due to refractive error, media opacity, or retinal abnormality such as an epiretinal membrane. Psychogenic disturbances should also be considered.
Approach
Using the clues from the history and examination, the examiner’s goals are to decide:
- 1.
Whether the diplopia or misalignment is a neurologic, ocular, or a childhood strabismus problem. If it is neurologic, then a lesion affecting the structures outlined in the anatomic pathways above, from supranuclear ones to muscle, may be implicated.
- 2.
Which extraocular muscles are involved.
- 3.
The cause. We suggest considering the most common causes of acquired misalignment by whether they typically cause horizontal or vertical diplopia ( Box 15.1 ).
Box 15.1
Horizontal vs Vertical Diplopia: Major Causes
Often the ophthalmoparesis and abnormal motility pattern is characteristic, and other historical features or accompanying neurologic findings aid in localization and diagnosis. A complete, isolated infranuclear third nerve palsy causes ipsilateral elevation, adduction, and depression weakness accompanied by abduction, hypodeviation, pupillary mydriasis, and ptosis. Patients with superior oblique paresis complain of vertical diplopia, often with a torsional component, and they have an ipsilateral hypertropia worse on contraversive (contralateral conjugate) horizontal gaze and ipsilateral head tilt. Patients with a lateral rectus palsy complain of binocular horizontal double vision worse on ipsiversive gaze and at distance. In many cases the limitation of abduction is evident, but, in more subtle instances, alternate cover or Maddox rod testing would confirm an esotropia largest on ipsiversive gaze. Small vertical deviations, consistent with normal hyperphorias, can be seen in sixth nerve palsies. Restrictive diplopia, due to orbital processes such as thyroid-associated ophthalmopathy, are associated with limited movements with normal saccadic velocity but positive forced duction testing. Proptosis and periorbital swelling are often present in such patients.
Another important consideration when determining the etiology of the diplopia is the age of the patient. In adults, diabetes, hypertension, trauma, and aneurysms are common causes of ocular motor palsies ( Table 15.1 ). In contrast, in most pediatric series, the most common causes of acquired IIIrd, IVth, and VIth nerve palsies are trauma and neoplasms. Vascular and aneurysmal causes of ocular motor palsies are uncommon in children.
Etiology | IIIrd (%) | IVth (%) | VIth (%) | Multiple (Any Combination of IIIrd, IVth, and VIth) (%) |
---|---|---|---|---|
Undetermined | 24 a | 32 a | 26 a | 12 |
Vascular | 20 b | 18 | 13 | 4 |
Head trauma | 15 | 29 b | 15 | 17 |
Aneurysm | 16 | 1 | 3 | 9 |
Neoplasm | 12 | 5 | 22 b | 35 a,b |
Other | 13 | 15 | 22 | 23 |
Treatment Overview
The ophthalmoparesis in some of the disorders described, such as ischemic and traumatic ocular motor palsies, will often resolve spontaneously. Other causes of diplopia, such as myasthenia gravis, or inflammatory, demyelinating, or neoplastic processes, will improve as the underlying condition is treated. In the meantime, patients with diplopia can wear an eyepatch over one eye or place opaque scotch tape on one lens of their eyeglasses to resolve the double vision. Some authors have advocated botulinum toxin injections into the antagonist muscle of the paretic muscle, even in supranuclear ocular motility disorders. For instance, in a left lateral rectus palsy, the left medial rectus palsy is injected with botulinum to decrease adduction tone. Prisms may be used to facilitate image alignment and are best for patients with relatively small (<15-prism diopter) comitant deviations. Temporary Fresnel paste-on prisms can be used initially, followed by ground-in prisms for permanent diplopia. Individuals with ophthalmoparesis who do not improve spontaneously, and whose examinations remain stable for at least 6 months, may be candidates for corrective eye muscle surgery.
Children with ocular motor palsies, especially those under age 8 years, have the potential for developing strabismic amblyopia. Third nerve palsies have the complicating feature of ptosis with the potential for deprivational amblyopia. These children, when able, should be referred to a pediatric ophthalmologist for patching or lid elevation. Children patched for symptomatic diplopia should have the patch alternated daily between eyes to prevent occlusion amblyopia.
Monocular Double Vision
Ophthalmic Causes of Monocular Double Vision
Most patients with monocular double vision have an optical problem, a macular abnormality, or a nonorganic symptom. Generally, the description of double vision that persists with one eye closed is different in each of these circumstances. Patients with optical causes of monocular double vision generally describe blurring of objects they are viewing and a “ghost image” or extra line. They usually do not see a discrete, second image but a lighter, partial second image that is attached to the main image and separates from it in one direction. The symptom almost always improves or disappears when the same object is viewed through a pinhole. Common causes of this type of double vision include cataracts, irregular astigmatism (particularly keratoconus), corneal scars, and tear film irregularities. If refraction with a rigid contact lens improves the double vision, the cause is likely to be an irregular corneal surface. Treatment of this type of double vision is etiology specific (i.e., refraction, rigid contact lens use, cataract extraction, or laser keratectomy).
Patients with macular causes of monocular double vision generally describe a break, bend, or distortion of the viewed edge leading to a double or distorted image. This type of monocular double vision generally does not improve with pinhole, and, in fact, vision is often worse through a pinhole in this setting. Common macular causes of monocular double vision include epiretinal membranes and retinal or choroidal folds. In addition, it should be noted that binocular diplopia can result from macular disease as well. In one mechanism, macular disease leads to significant image distortion or difference in image size compared with the other eye (aniseikonia). This image size or shape discrepancy prevents fusion of images despite normal alignment. Alternatively, double vision may result from macular displacement by vitreoretinal traction or epiretinal membrane, for instance.
Finally, patients with nonorganic or functional vision loss often complain of monocular double vision. In our experience this is an ill-defined symptom which cannot be further characterized by the patient and may affect one or both eyes. Double vision may be reported only after the examiner asks specifically about it when trying to characterize a complaint of “blurred vision.” In this setting, patients may also complain of triple or quadruple vision. The symptom does not improve with pinhole or any other maneuver on the part of the examiner. Nonorganic vision loss is discussed further in Chapter 11 .
Neurologic Causes of Monocular Double Vision
Rarely, acquired occipital lobe lesions or drugs may cause multiple images, akin to palinopsia. These types of visual illusions are discussed in Chapter 12 .
Supranuclear Causes of Diplopia, Including Vergence Disorders
This section reviews diplopia caused by supranuclear lesions: internuclear ophthalmoplegia (INO) and the major vergence disorders (abnormalities of moving the eyes in opposite directions), which are convergence spasm, convergence and divergence insufficiency, and pseudoabducens palsy, which cause horizontal diplopia, and skew deviation and monocular elevator palsy, which lead to vertical diplopia.
Internuclear Ophthalmoplegia
Disruption of the MLF in the pons or midbrain results in an INO typified by an ipsilateral adduction deficit and contralateral abducting nystagmus ( Figs. 15.10 and 15.11 , ). The side of the adduction deficit determines the side of the INO.




Symptoms . Patients with INO may complain of horizontal diplopia, particularly when the adduction weakness is profound. Those patients with subtle paresis may have no symptoms or complain of blurred vision only with eccentric gaze or quick shifts in horizontal gaze. Since a skew deviation (discussed later) is frequently associated with lesions of the MLF, vertical diplopia may be another complaint. Oscillopsia may result from either the abduction nystagmus or an impaired vertical vestibular ocular reflex.
Signs . The hallmark finding of INO is impaired adduction of the eye (see Fig. 15.10 ). There is a wide range of medial rectus dysfunction in INO. Subtle cases may be evident only with fast eye movements from eccentric gaze to midline (“medial rectus float”). An optokinetic flag pulled along the horizontal plane may reveal slow adducting saccades in the affected eye during the fast phase of the nystagmus. The demonstration of intact convergence in INO establishes the supranuclear localization of the medial rectus weakness and usually signifies a lesion of the MLF within the pons ( ). In contrast, patients with MLF lesions close to the third nerve nucleus may have impaired convergence. However, some patients with INO have poor convergence because of the vertical misalignment produced by the associated skew deviation. Therefore, the absence of convergence may not be a totally reliable sign to localize the lesion in a discrete part of the MLF pathway.
The eye that is contralateral to the MLF lesion typically exhibits an abducting nystagmus in endgaze. Evidence suggests that this abducting nystagmus is an adaptive phenomenon in an attempt to increase the innervation to the weak adducting eye.
Since the otolith pathways project through the MLF, a skew deviation and ocular tilt reaction is commonly observed with an INO (see later discussion). Typically, the hypertropic eye is on the same side as the adduction weakness. A dissociated form of vertical torsional nystagmus may also be observed because of disrupted connections from the posterior semicircular canal. This results in a downbeating nystagmus in the eye ipsilateral to the MLF lesion and torsional nystagmus in the contralateral eye. Bilateral lesions of the MLF often produce additional eye findings, including impaired vertical pursuit and upbeat nystagmus in both eyes in upgaze. Severe bilateral INOs may also result in a large-angle exotropia in primary gaze in so-called “WEBINO” ( w all- e yed b ilateral INO ) ( ).
Pathophysiology . Axons for the MLF arise from interneurons intermingled within the abducens nucleus (see Fig. 15.11 ). These axons immediately cross the midline and ascend in the MLF. Lesions of the MLF produce impaired adduction of the ipsilateral eye. Since other important pathways travel in the MLF, a variety of other neuro-ophthalmologic signs may be found. The utricular pathway arising from the vestibular nucleus ascends in the MLF. Interruption of these vestibular connections results in an ipsilateral hypertropia. Vestibular information also travels to and from the inC. Interruption of these connections in the MLF, particularly when bilateral, will impair (1) vertical pursuit, (2) the vertical vestibular ocular reflex, and (3) vertical gaze–holding mechanisms.
Diagnosis . The diagnosis of INO is usually straightforward, especially when there is impaired medial rectus dysfunction in lateral gaze with normal convergence. One should carefully search for other orbital signs, since isolated medial rectus lesions may occur from local conditions such as extraocular muscle entrapment. Third nerve palsy should be considered, and ptosis, mydriasis, and vertical eye movement impairment should be carefully excluded. Exotropia resulting from strabismus can be distinguished by the presence of full extraocular movements in lateral gaze and normal saccades on gaze or optokinetic testing. Caution should be applied in this regard, however, because some patients with long-standing large-angle exotropia may develop adduction weakness, perhaps on a restrictive basis, which may mimic an INO. Myasthenia gravis can rarely mimic an INO (see Myasthenia Gravis ). In such patients there are often eyelid signs such as variable ptosis to suggest this diagnosis.
Differential diagnosis . A unilateral INO in an older patient usually results from a brainstem infarction, while bilateral INO in a young patient typically signifies a demyelinating process such as multiple sclerosis ( Fig. 15.12 ). INOs due to infarcts may result from small vessel (penetrator) occlusion or large vessel disease involving the posterior cerebral artery, superior cerebellar artery, or even the basilar artery. In both ischemic and demyelinating causes, the prognosis for spontaneous improvement over weeks is excellent.


However, a variety of lesions may be associated with INOs, including vascular malformations, tumors, head trauma, nutritional disorders including Wernicke’s disease, metabolic disorders such hepatic encephalopathy, structural abnormalities (Arnold–Chiari malformation), and degenerative conditions, particularly progressive supranuclear palsy.
In many patients with an INO, a lesion of the MLF in the midbrain or pons can be demonstrated on MRI, but for unclear reasons often the brainstem appears normal.
Variations . The combination of an INO with an ipsiversive conjugate palsy is a pontine “one-and-a-half” syndrome due to simultaneous ipsilateral involvement of the PPRF (or VIth nerve nucleus) and the MLF (see Chapter 16 , and Figs. 16.7 and 16.8 ). Damage to the MLF in addition to the corticospinal tracts results in Raymond-Cestan syndrome, characterized by an INO and contralateral hemiparesis. Rarely patients with impaired abduction will have adducting nystagmus. This has been sometimes referred to as a posterior INO of Lutz. This combination of eye signs most likely results from an abducens palsy with the adducting nystagmus representing an adaptive response.
Convergence Spasm
This condition, characterized by convergence, accommodation, bilateral pupillary miosis, and pseudomyopia, may mimic bilateral sixth nerve palsies ( ). The distinction is important because convergence spasm (or spasm of the near reflex) is almost always indicative of a functional disorder. Patients usually complain of double or blurry vision, and they often have other behaviors and symptoms which suggest a nonorganic etiology. The hallmarks are pupillary constriction on attempted abduction and a variable esotropia (reflecting variable patient effort). Other features include normal abducting saccades, intact abduction during the oculocephalic maneuver or testing of monocular ductions, and resolution of the myopia following cycloplegia. The miosis may resolve upon occlusion of the fellow eye by disrupting the binocular input necessary for convergence.
Rarely, spasm of the near reflex may be seen in disease states such as metabolic encephalopathy, Arnold–Chiari malformation, pituitary tumors, and trauma. Treatment of the condition consists of cycloplegic eye drops (e.g., atropine) to inhibit accommodation or weak minus lenses. Other possible treatment options include monocular occlusion, opacification of the medial third of the lenses, and botulinum toxin injection. However, patient reassurance that no organic disease exists is often the best approach. Convergence spasm should be distinguished from convergence retraction nystagmus or pseudoabducens palsy from pretectal lesions.
Convergence Insufficiency
Patients with convergence insufficiency describe horizontal diplopia at near, typically after a period of reading. In a practical definition, patients with this condition are unable to maintain the esodeviation required for near tasks. Once an object is brought to within 10 cm of the bridge of their nose, affected patients are unable to converge. Medial rectus function is normal when ocular ductions are tested, but patients typically have an exodeviation worse at near. A common sequela of head trauma, convergence insufficiency may also be seen in association with dorsal midbrain lesions (Parinaud syndrome; see Chapter 16 ), may be a decompensation of a long-standing exophoria, or may be idiopathic. The exact anatomical lesion which causes convergence insufficiency is unknown.
Convergence exercises such as “pencil push-ups,” in which the patient is asked to focus on the pencil eraser as it is repeatedly brought close to the eyes, and base-out prisms, which forces convergence, can be tried. Office-based vision therapy and orthoptics with home reinforcement are superior to home-based therapy. Strabismus surgery may be required for individuals who fail medical therapy. A prospective trial assessing the effect of office-based vergence and accommodative therapy versus placebo in children with convergence insufficiency is underway.
Divergence Insufficiency
This ocular motility deficit is characterized by an acquired horizontal diplopia at distance but not near, a comitant esophoria or esotropia at distance, near motor fusion at near, and full ocular ductions without evidence of a sixth nerve palsy. The abnormality has been attributed to a disturbance of a poorly defined supranuclear “divergence center” in the brainstem. Divergence insufficiency is typically benign, and affected patients are usually neurologically normal. Nonspecific small vessel ischemic disease is a relatively common finding in magnetic resonance imaging (MRI) studies in such instances and is of uncertain significance. While most patients with divergence insufficiency have no neurologic abnormalities, a subset of patients may have concomitant nystagmus, tremor, and ataxia or an underlying neurologic disorder such as Parkinson’s disease or a Chiari malformation.
Divergence insufficiency may be seen in patients with high myopia and is believed to be due to displacement of the lateral recti inferiorly and superior rectus nasally as the result of degeneration of the lateral rectus–superior rectus band that connects these muscles. These patients may have an abduction deficit and lateral incomitance that mimics a VIth nerve palsy.
Since small bilateral sixth nerve palsies may mimic divergence insufficiency, intracranial causes of subtle bilateral sixth nerve palsies due to elevated intracranial pressure and brainstem and skull-based lesions should be excluded with neuroimaging. Patients with pontine lesions associated with divergence insufficiency have been reported, and in two of these, discrete lesions in the unilateral tegmentum of the caudal pons were demonstrated, suggesting that involvement of the nucleus reticularis tegmenti pontis might be responsible for this ocular motor disorder.
Patients can be treated extremely effectively with base-out prisms or strabismus surgery.
Pseudoabducens Palsy
Pseudoabducens palsy is reviewed in detail in Chapter 16 because it often accompanies the dorsal midbrain syndrome or thalamic lesions. Although the lateral rectus appears to be weak, oculocephalic maneuvers demonstrate full horizontal ductions. The esotropia is usually variable and may represent excess convergence tone.
Skew Deviation
Skew deviation refers to a vertical misalignment of the eyes that commonly occurs from acute brainstem lesions which disrupt prenuclear vestibular pathways to the third and fourth nerve nuclei. Occasionally, skew deviation may also result from peripheral vestibular or cerebellar lesions.
Symptoms and signs . Patients may complain of binocular vertical diplopia, sometimes with a torsional component. The presence of other neurologic symptoms and signs often helps to distinguish skew deviation from other causes of vertical misalignment such as third and fourth nerve palsies. For example, a vertical deviation of the eyes with signs of pontomedullary dysfunction, such as ataxia, usually suggests a skew deviation is present.
Ocular-tilt reaction (OTR) . The combination of skew deviation with ocular torsion and a head tilt is known as the ocular-tilt reaction ( Fig. 15.13 ). This triad of signs is typically observed with lesions of the lateral pontomedullary junction ( Fig. 15.14 ) or the paramedian thalamic–mesencephalic region and results from dysfunction of the utricular pathways that begin in the labyrinths and terminate in the rostral brainstem. Although the exact neuroanatomic pathways that mediate the ocular-tilt reaction or its prime component, skew deviation, remain uncertain, the localizing value of skew deviation has evolved from clinical and experimental observation ( Table 15.2 ).








Lesion Location | Type of Skew | Associated Signs |
---|---|---|
Lateral pontomedullary | Contralateral hypertropia | Ipsilateral excyclotorsion; ipsilateral facial numbness; ipsilateral Horner; lateropulsion |
Midline cervicomedullary junction | Bilateral inferior rectus | Downbeat nystagmus |
Medial longitudinal fasciculus | Ipsilateral hypertropia | Internuclear ophthalmoplegia |
Rostral midbrain | Ipsilateral hypertropia | Vertical gaze palsy; conjugate cyclotorsion |
Pathophysiology . It appears that both the utricular and the semicircular canal pathways that regulate vertical gaze synapse in the vestibular nuclei and cross the midline to ascend in the MLF ( Fig. 15.15 ). There, the utricular pathway connects to the nuclei that activate the four vertically acting muscles, namely the superior rectus, superior oblique, inferior rectus, and inferior oblique. Because the superior rectus subnucleus controls the contralateral superior rectus, and the trochlear nucleus innervates the contralateral superior oblique muscle, a right utricular nerve lesion, for example, would result in a left hypertropia as a result of impaired action of the left inferior rectus muscle and the right superior rectus muscle. If the lesion occurs in the MLF, the hypertropia will be ipsilateral unless lateral pontomedullary structures are also affected, and in such a case the hypertropia may be contralateral to the INO.

Skew deviation may also ensue following selective damage to the posterior or the anterior semicircular canal pathways. For example, injury to the right posterior semicircular canal projections would lead to a left hypertropia and relative right eye excyclotorsion from impaired innervation to the left inferior rectus and right superior oblique muscles, respectively. Although both eyes are torted toward the hypotropic eye, the lower eye is usually more excyclorotated than the higher eye is incyclorotated—hence the term relative excyclorotation. Bilateral damage to the crossing posterior semicircular pathway projection in the dorsal medulla could lead to an alternating hypertropia (i.e., right hypertropia in right gaze from impaired action of the right inferior rectus muscle, and a left hypertropia in left gaze from disturbed function of the left inferior rectus muscle). Patients who have skew deviation from interruption of the semicircular canal pathway often have associated vertical nystagmus. For instance, interruption of the posterior semicircular canal pathway leads to a skew deviation in association with downbeat nystagmus in one eye and a torsional nystagmus in the fellow eye.
Although brainstem strokes and tumors are the most common causes of skew deviation, this motility disturbance may be rarely observed as a false localizing sign of increased intracranial pressure including pseudotumor cerebri syndrome.
Diagnosis . The hypertropia of a skew deviation may be comitant (deviation is the same in all positions of gaze) or noncomitant (deviation varies with gaze position). When a skew deviation is noncomitant, it may be difficult to distinguish from an ocular motor palsy or extraocular muscle weakness. A fourth nerve palsy is the motility disorder that may be the most difficult to distinguish from a skew deviation since both conditions may be associated with a positive head-tilt or three-step test (see Fourth Nerve Palsies ). However, patients with skew deviation typically have binocular torsion as opposed to the excyclotorsion of just one eye seen in a fourth nerve palsy. Furthermore, in skew deviation the hypertropic eye is usually incyclorotated and the hypotropic eye excyclorotated, while the affected hypertropic eye in a fourth nerve palsy is excyclorotated, and in an inferior oblique palsy the affected hypotropic eye is incyclorotated. Torsion may be demonstrated by double Maddox rod testing ( Fig. 15.16 ), fundus photography (see Fig. 15.14 ), indirect ophthalmoscopy, or measurement of the subjective visual vertical. In addition, the supranuclear nature of the hyperdeviation associated with skew deviation may be evident during oculocephalic testing or forced eyelid closure (Bell’s phenomenon). Finally, the vertical deviation and torsion seen in skew deviation decreases when patients are supine compared with trochlear nerve palsy, in which they remain the same.

Midbrain lesions involving the INC may also produce skew deviation with the hypertropic eye ipsilateral to the lesion. A full OTR may also ensue with midbrain lesions producing a conjugate counter-roll of the eyes toward the contralateral shoulder ( Fig. 15.17 ). The head tilt will be contralateral to the lesion as well. Alternating skew with lateral gaze may also be associated with midbrain lesions.


Variations . Occasionally, irritative lesions of the midbrain will produce a paroxysmal skew deviation. These patients have a contralateral hypertropia, ipsilateral head tilt, and a conjugate counter-roll of the eyes toward the ipsilateral shoulder. One patient has been documented with skew deviation from ictal activity of the vestibular cortex.
Alternating skew deviation in the primary position is an unusual eye movement disorder that may be observed with midbrain lesions. The hypertropia may switch sides over several minutes in an aperiodic or periodic manner. Periodic alternating deviation may also coexist with periodic alternating nystagmus.
Acquired Supranuclear Monocular Elevator Palsy
Acquired supranuclear monocular, or “double elevator,” palsy is caused by a pretectal, supranuclear lesion. Both superior rectus and inferior oblique function of one eye are affected by a unilateral lesion in the rostral mesencephalon. Such a lesion would disrupt the efferent fibers from the riMLF) to the inferior oblique subnucleus and the contralateral superior rectus subnucleus, which innervates the superior rectus muscle contralateral to it (see Fig. 16.18 ). Thus a unilateral lesion produces a monocular elevation paresis. As the name of the condition implies, Bell’s phenomena and the oculocephalic responses are intact in the affected eye. If reflex eye movements are not preserved with a monocular elevator palsy, one should consider a fascicular third nerve palsy or a lesion along the distal path of the third nerve including the orbit.
Third Nerve Palsies
As indicated earlier, a complete, isolated infranuclear third nerve palsy causes ipsilateral elevation, adduction, and depression weakness accompanied by abduction, hypodeviation, pupillary mydriasis, and ptosis ( Fig. 15.18 , and ). The most feared cause of a third nerve palsy in adulthood is a posterior communicating artery (PCoA) aneurysm, which may compress the third nerve within the subarachnoid space. In adults, the most common identifiable cause of acquired third nerve palsy is presumed vascular insufficiency due to diabetes, hypertension, or atherosclerosis. Less common causes of isolated third nerve palsies include trauma, meningitis, stroke, pituitary apoplexy, and mass lesion at the base of the skull or cavernous sinus. In children the most common cause of acquired third nerve palsy is trauma. This section discusses other clinical features of third nerve palsies, then details their various etiologies and workup in adults and children.






Other Clinical Features of Third Nerve Palsies
Complete vs incomplete (or partial) . These terms refer to the extent of involvement of the eyelid and IIIrd nerve innervated extraocular muscles. These are nonlocalizing. Palsies of individual muscles can occur due to lesions at any level of the nerve.
Pupil involvement vs sparing . If the pupil is mydriatic and less reactive to light than the pupil of the fellow eye, the pupil is said to be “involved.” A normally reactive pupil which is the same size as the pupil of the fellow eye is “spared.” These features are nonlocalizing.
Inferior vs superior division . As mentioned earlier, the third nerve divides into two divisions in the anterior cavernous sinus, so divisional ocular paresis classically localizes to this region or to the posterior orbit. However, there are several case reports describing either a divisional palsy or single muscle involvement due to lesions anywhere along the course of oculomotor nerve, suggesting that topographic organization exists along the entire course of the nerve from midbrain to the orbit ( Fig. 15.19 ).




Aberrant regeneration . Patients with traumatic or chronic compressive IIIrd nerve palsies may develop aberrant regeneration, misdirection, or synkinesis. Common abnormal patterns include lid elevation during adduction or depression of the eye (pseudo-Graefe phenomenon) ( Fig. 15.20 ) or depression and constriction of the pupil during adduction but not to light (see Fig. 13.12 , ). Pupillary findings are discussed in more detail in Chapter 13 . Less common patterns include defective vertical eye movements with retraction of the globe upon attempted vertical deviations, adduction of the eye during attempted vertical movement, and transient synkinesis.



Primary (spontaneous) and secondary (acquired) aberrant regeneration are usually considered separately because of their etiologic implications. The synkinesis is the first manifestation of third nerve dysfunction in primary aberrant regeneration. This type, manifesting as a ptotic eyelid which elevates in adduction or depression of the eye, is usually associated with expanding lesions in or around the cavernous sinus such as intracavernous meningiomas, aneurysms, pituitary tumors, and other extracavernous masses. On the other hand, secondary aberrant regeneration occurs in the recovery phase several weeks after an acute third nerve palsy due to trauma, ophthalmoplegic migraine, pituitary apoplexy (see Fig. 13.12 ), or inflammation, for instance. Because aberrant regeneration after microvascular ischemic nerve palsies or midbrain infarction or hemorrhage is rare, in the absence of a history of trauma its presence should heighten suspicion for a compressive lesion.
In one purported mechanism, damaged, regenerating axons follow alternate pathways within the nerve sheath to innervate targets distinct from their original destination. Lid elevation in adduction would then be explained by misdirection of third nerve fibers for the medial rectus muscle incorrectly innervating the levator muscle. Similarly, miosis during adduction could be attributed to medial rectus fibers mistakenly innervating the pupillary sphincter. However, cases of spontaneous and transient synkinesis might argue against hardwired misdirection. Alternative explanations include (1) ephaptic transmission, a cross-talk between adjacent injured and intact nerve fibers, and (2) central synaptic reorganization within the oculomotor subnuclei.
Cyclic oculomotor spasms . In this eye movement disorder, a complete or third nerve palsy alternates with brief spasms of the third nerve innervated structures. Often heralded by eyelid twitches, the spastic phase is characterized by pupillary miosis, lid elevation, adduction, and depression but limited abduction of the eye ( Fig. 15.21 , ). The condition is usually unilateral, persists during sleep, and continues for the lifetime of the patient. Most cases are seen in congenital third nerve palsies, but rarely they may be acquired. Alternating phases of aberrant regeneration of the third nerve with an intermittent conduction block has been a proposed mechanism.


Etiologies of third nerve palsies and their accompanying clinical features are discussed by location in the following sections.
Intraaxial Lesions
Nuclear third nerve palsy . The classical unilateral lesion of the IIIrd nerve nucleus results in bilateral ptosis that is worse ipsilaterally; ipsilateral mydriasis; ipsilateral palsy of the medial rectus, inferior rectus, and inferior oblique muscles; and bilateral superior rectus palsies. However, bilateral ptosis with various combinations of oculomotor paresis have been described from nuclear IIIrd nerve lesions. Other cases of nuclear IIIrd nerve palsies have been described without levator involvement, with isolated inferior rectus palsy, and with isolated contralateral superior rectus palsy. Such patients without bilateral ptosis would be difficult to discern clinically from fascicular involvement only.
Fascicular third nerve palsy . Lesions of the midbrain tegmentum can affect the oculomotor nerve fascicles as they travel ventrally and are often associated with “crossed” neurologic signs. Involvement of the fascicle as it passes through the crossing dentatorubrothalamic fibers (from the superior cerebellar peduncle), just below and medial to the red nucleus, can produce an ipsilateral oculomotor palsy and contralateral ataxia (Claude’s syndrome) ( Fig. 15.22 ). A lesion of the cerebral peduncle results in ipsilateral oculomotor palsy and contralateral hemiparesis (Weber syndrome), and a larger process also involving the red nucleus can cause the same findings plus contralateral involuntary limb movements or tremor (Benedikt’s syndrome). Third nerve palsy accompanied by vertical gaze paresis, lid retraction, skew deviation, and convergence retraction saccades would be suggestive of dorsal midbrain process, as in the top of the basilar syndrome (see Chapter 16 ). In this setting, emboli to the posterior cerebral arteries may also result in a homonymous hemianopsia and visual “peduncular” hallucinations (see Chapter 12 ). Secondary diencephalic lesions may cause additional memory disturbances, behavioral changes, and altered consciousness.


Fascicular oculomotor palsies may also be isolated, and either pupil involvement or sparing may occur. In incomplete oculomotor palsies, the pattern of ophthalmoplegia often reflects the topographical arrangement of the fibers to each extraocular muscle within the fascicle (see Fig. 15.4 ). Divisional pareses may occur, and, uncommonly, individual muscles may be affected.
The usual cause of a nuclear or fascicular oculomotor palsy is infarction in the territory of a mesencephalic paramedian penetrating vessel arising from the proximal posterior cerebral artery, but other etiologies include metastatic tumors, vascular malformations, abcesses, cysts, and multiple sclerosis.
Subarachnoid Processes
Uncal herniation . A third nerve palsy accompanied by altered mental status should suggest uncal herniation. Ipsilateral pupillary dilation may be the first sign of transtentorial uncal herniation before other signs of third nerve paresis develop. In this setting, mechanisms for third nerve dysfunction include direct compression by the herniating uncus beneath the tentorial edge ( Fig. 15.23 ), compression by the posterior cerebral artery or hippocampal gyrus, compression of the oculomotor complex in the midbrain, or displacement and kinking of the nerve over the clivus. As the syndrome progresses with a decrease in level of consciousness, the third nerve palsy may become complete along with signs of ipsilateral hemiplegia and extensor plantar response (due to contralateral compression of the cerebral peduncle—Kernohan’s notch syndrome). Further clinical progression may be monitored by examining the opposite pupil, which initially may be midposition with a depressed light reaction, then slightly miotic, then finally dilated. Transiently the pupils may acquire an oval shape. Uncommonly in transtentorial herniation, the opposite pupil is paradoxically the first to dilate.


Posterior communicating artery aneurysms . Within the subarachnoid space, PCoA aneurysms may compress the third nerve ( Fig. 15.24 ), almost always causing pupillary dilation. Aneurysms arising from the base of the PCoA as it emerges from the ICA are the second most common aneurysm in the anterior circle of Willis. In one study, 34% of patients with a PCoA developed a IIIrd nerve palsy. However, greater than 90% of patients with PCoA aneurysms and subarachnoid hemorrhage present with symptoms of a IIIrd nerve palsy before rupture. PCoA aneurysms and ICA aneurysms commonly project posterolaterally to compress the oculomotor nerve as it travels between the brainstem and cavernous sinus ( Fig. 15.25 ). Unruptured PCoA aneurysms may cause a third nerve palsy by mechanical compression or pulsatility. Larger aneurysms, particularly those larger than 5 mm, are more likely to cause a IIIrd nerve palsy.


The IIIrd nerve palsy resulting from aneurysmal compression is usually painful and complete. Because of the dorsomedial location of the pupillomotor fibers in the oculomotor nerve bundle, aneurysmal compression often involves the pupil early, even before complete ophthalmoparesis. However, pupillary sparing may occur, and, in one study, 14% of IIIrd nerve palsies due to posterior communicating aneurysms presented with normal pupils and partial motility deficits, but nevertheless pupillary mydriasis developed within days in most of those cases. Rare oculomotor presentations of PCoA aneurysms include isolated ptosis, ptosis and mydriasis, pupillary light-near dissociation, dorsal midbrain syndrome, pupil sparing and aberrant regeneration, and transient third nerve palsies. Visual loss may occur, and the pattern observed with PCoA aneurysms depends upon the anterior pathway structure which is compressed: optic nerve, chiasm, or tract.
Treatment. Treatment of symptomatic PCoA aneurysms should not be unduly delayed, as bleeding and/or rebleeding and death may occur when they are untreated. Treatment options consist of surgical clipping, endovascular embolization, and less often carotid occlusion. Advances in endovascular approaches, particularly coil embolization, have resulted in improved recovery rates of IIIrd nerve palsies and lower frequency of acute bleeding in the treatment of ruptured and unruptured aneurysms. However, a recent metaanalysis concluded that surgical clipping of PCoA aneurysms causing IIIrd nerve palsy achieved better recovery compared with endovascular coiling. Ultimately, the treatment strategy for patients with an aneurysmal IIIrd nerve palsy should be individualized, with technical factors and the patient’s underlying medical problems often determining the best approach.
After treatment of PCoA aneurysms, the recovery of the IIIrd nerve palsy is inconsistent and often slow. Diplopia, residual ptosis, and occasionally photophobia are the main complaints, and aberrant regeneration is often seen. Factors predicting a better prognosis for recovery of IIIrd nerve function include early treatment, incomplete palsies, younger age, absence of diabetes, and an unruptured aneurysm.
Basilar artery aneurysms . Aneurysms of the basilar artery, trunk and bifurcation, are the most common aneurysm in the posterior fossa (see Fig. 15.25 ). The most frequent presentation is subarachnoid hemorrhage, but cranial nerve pareses are frequent, IIIrd and VIth nerve palsies being most common. Patients with IIIrd or VIth nerve pareses may develop a concurrent crossed paralysis such as a Weber syndrome if the aneurysm injures the adjacent brainstem. Typically the IIIrd nerve palsies are pupil-involving, but pupil-sparing IIIrd nerve palsies in this setting have been reported.
Other neurologic and neuro-ophthalmic signs may develop from basilar aneurysms due to brainstem compression or concurrent thromboembolic disease. Giant saccular or fusiform aneurysms may compress the adjacent midbrain or pons. Hemiparesis or other corticospinal tract signs may or may not be evident depending on the duration of the compression. Thrombus within the basilar aneurysm may obstruct flow or embolize to nearby perforating vessels, particularly at the basilar bifurcation. Basilar artery insufficiency may result from midbasilar thrombosis. On rare occasions, giant fusiform aneurysms of the basilar artery may result in obstructive hydrocephalus due to aqueductal compression
Other aneurysms . Posterior cerebral artery (PCA) aneurysms may result in IIIrd nerve palsies by compressing the oculomotor nerve as it travels between the posterior cerebral artery and superior cerebellar artery. Giant PCA aneurysms can compress the optic radiations or visual cortex, producing a contralateral homonymous hemianopia.
Treatment. Therapy for posterior fossa aneurysms is either surgical or endovascular. Both treatments are complicated by significant morbidity and mortality due to occlusion of small perforating vessels or parent vessel thrombosis. For instance, clipping of basilar tip aneurysms may cause infarction in paramedian mesencephalic–thalamic perforating territories. Surgical therapy is additionally complicated by cranial nerve palsies secondary to traction. The IIIrd nerve palsy is most common, occurring in more than 60% of patients. Despite the significant risk, surgical treatment is often warranted due to the even greater morbidity and mortality associated with progressive brainstem dysfunction and posterior fossa subarachnoid hemorrhage (SAH). If vessel occlusion can be tolerated in the vertebral or basilar artery, then the endovascular approach is often the safest. In certain cases of basilar artery dolicoectasia, endovascular occlusion is the only feasible approach.
Vasculopathic third nerve palsies . Ischemic (vasculopathic) third nerve palsies, associated with hypertension, diabetes, advanced age, atherosclerosis, smoking, and hypercholesterolemia, are often preceded by orbital ache or pain and are characterized by pupillary sparing ( Fig. 15.26 ). In our experience, the pain can be rather severe, especially in younger patients. The pain may precede the onset of the palsy and can persist for weeks. The presence or absence of pain cannot be used to distinguish aneurysmal from vasculopathic third nerve palsies. The symptoms and signs may progress over several days.





Most cases are thought to have a peripheral lesion. Presumably the third nerves develop ischemia and demyelination within the subarachnoid space or cavernous sinus, but there may also be a small infarction of the nerve within the mesencephalon. The pupil sparing in peripheral cases is attributed to the location of the pupillary fibers on the outside of the third nerve in areas less vulnerable to vascular insufficiency. However, some studies have suggested that minor pupillary involvement (less than 2 mm of anisocoria) may occur in as many as 38% of patients. The pupil is rarely dilated and unreactive. The pain may be due to ischemia of trigeminal fibers within or around the oculomotor nerve, but this has not been confirmed. Most patients with vasculopathic oculomotor palsies recover spontaneously within 8–12 weeks. Rarely, vasculopathic IIIrd nerve palsies may be seen in combination with IVth, VIth, and VIIth nerve palsies. Other responsible etiologies include vasculitis due to giant cell arteritis, systemic lupus erythematosus, and herpes zoster. There is no evidence that antiplatelet agents prevent ischemic oculomotor palsies.
Trauma. Third nerve palsies due to trauma are typically caused by severe blows to the head, often due to a motor vehicle accident. Traumatic third nerve palsies are more commonly due to frontal or parietal lobe traumatic injuries. The peripheral portion of the nerve may be damaged secondary to traction at the skull base or by an orbital or base of skull fracture. Alternatively, the nuclear complex or fascicle may be affected by brainstem shearing or hemorrhage. Alternative causes include penetrating orbital or intracranial trauma or iatrogenic trauma during removal of suprasellar or posterior fossa masses. Some oculomotor palsies from minor trauma are harbingers of an underlying aneurysm or mass lesion. The prognosis for recovery of function in most cases of traumatic IIIrd nerve palsies is very good, but often with evidence of aberrant regeneration.
Primary Tumors of the Oculomotor Nerves
Neurinomas and schwannomas. Stable or slowly progressive oculomotor palsies may be caused by tumors derived from the Schwann cells surrounding the extraaxial portion of the IIIrd nerve. Neurofibromatosis type 2 is a risk factor for such tumors. Neuroimaging typically reveals an enhancing, thickened IIIrd nerve in the subarachnoid space or cavernous sinus, but enlargement to several centimeters with mass effect can occur. Transient and recurrent oculomotor cranial nerve palsies have also been reported. The best management of these cases is uncertain. Because of their benign histology, complete removal is not necessary (though has been done ) unless the lesions become large enough to compress surrounding structures. It is unclear whether radiation therapy is useful.
Other neoplasms. Uncommon considerations include primary neoplasms of the oculomotor nerve such as malignant meningioma and primary glioblastoma multiforme.
Other causes of third nerve palsies . Base of skull neoplasms, infectious disorders, and inflammatory disorders are discussed in the section Miscellaneous Causes of Combined Third, Fourth, and Sixth Nerve Palsies. Less common causes include sinus mucoceles and carotid insufficiency, intraneural cavernous hemangioma, and radiation damage.
Diagnostic Evaluation of Adults With Acquired Third Nerve Palsies
Although controversial, we believe that all patients with acute third nerve palsies should have neuroimaging and directed laboratory evaluation. Alternative guidelines for observing some affected patients have been based upon the patient’s age, pupil involvement, and completeness of the motility deficit. However, in our experience conservative observation may miss an underlying responsible vascular process or mass lesion in 4.6% of cases. Even isolated, complete pupil-sparing third nerve palsies can be caused by small infarcts in the mesencephalon and compressive lesions, so we have lowered our threshold to scan patients with isolated ocular motor palsies early in their course.
In adults with acquired, isolated third nerve palsies, particularly those which are pupil-involving, an aneurysm at the junction of internal carotid artery and the PCoA should be excluded emergently. Isolated third nerve palsies may also result from an aneurysm at the apex of the basilar artery or at the junction between posterior cerebral and superior cerebellar artery. Immediate computed tomography (CT) or MRI together with noninvasive angiography should be performed. The sensitivity of CT angiography (CTA) in the detection of aneurysms >5 mm in diameter is 95% ; the sensitivity decreases somewhat for the detection of smaller aneurysms. The sensitivity of magnetic resonance angiography (MRA) is similar to CTA in the detection of aneurysms >5 mm, although this falls dramatically to 54% for those that are <5 mm. On the other hand, the spatial resolution of an MRI provides valuable information, including displacement of adjacent structures and presence of intraluminal thrombus. It has been suggested that MRA should be combined with MRI when ruling out aneurysm as a cause of the third nerve palsy. The expertise of the radiologist is an important factor in the detection of aneurysms by CTA or MRA. If MRI or CT and angiography are unrevealing and the index of suspicion is high, conventional angiography may be necessary to exclude an aneurysm.
Other conditions that can mimic isolated pupil-sparing third nerve palsies in adults include myasthenia gravis, thyroid eye disease, and giant cell arteritis (due to muscle ischemia). When these disorders are considered, an acetylcholine receptor antibody level, erythrocyte sedimentation rate, and C-reactive protein may be helpful.
We also have a low threshold for neuroimaging in patients with traumatic ocular motor palsies, even when isolated. These individuals may have a pseudoaneurysm or posteriorly draining cavernous sinus fistula (see later discussion). In the setting of minor trauma, an underlying aneurysm or mass lesion should be excluded. Extraocular muscle entrapment resulting from orbital wall fracture may mimic an oculomotor palsy.
Meningeal processes should also be considered in patients with oculomotor palsies, especially when they occur bilaterally or in combination, and when other cranial nerves or nerve roots are involved. MRI with gadolinium may disclose meningeal enhancement. In such instances, cerebrospinal fluid (CSF) examination, including cultures and cytology, should be performed to exclude acute bacterial and chronic fungal meningitis, tuberculosis, syphilis, Lyme disease, sarcoidosis, and carcinomatous or lymphomatous meningitis. In oculomotor palsies due to Guillain–Barré syndrome, the Fisher variant, or chronic inflammatory demyelinating polyneuropathy, the CSF protein should be elevated.
Course and Management of Third Nerve Palsy
Most microvascular palsies recover spontaneously within 3 months. Treatment of systemic predisposing factors such as metabolic control of diabetes and hyperlipidemia and control of hypertension is generally recommended in this instance. Traumatic and aneurysmal cases may not recover completely and may develop aberrant regeneration.
The management of patients with third nerve dysfunction poses several challenges, as it is the most complicated of all forms of paretic strabismus. This results from the potential involvement of four muscles and a complex pattern of extraocular muscle dysfunction. All decisions concerning the management of patients with third nerve palsy are divided into those which are recommended acutely and those recommended chronically when there is a fixed deficit. For acute management of diplopia from third nerve palsy, monocular occlusion and prisms can be used. In select cases botulinum toxin can be injected into the lateral rectus muscle, thereby reducing the horizontal deviation and alleviating diplopia (occasionally with the aid of vertical prisms). Photophobia secondary to persistent pupillary dilation can be ameliorated with pharmacologic miosis or tinted lenses.
Once the palsy has been determined to be chronic and measurements have been stable for at least 6 months (preferably 12 months), various surgical strategies can be used to attempt to achieve realignment in the primary position and occasionally a useful field of single binocular vision. In the setting of complete third nerve palsy, only realignment in the primary position can be hoped for. The surgeon must also consider the primary position ptosis. Many authorities feel that in the setting of complete ptosis, repair of the extraocular muscle alignment is not indicated. Furthermore, if eyelid elevation is defective and ptosis correction is attempted, the risk of corneal exposure is significant, particularly if supraduction is limited. Patients must be warned that, although there may be significant cosmetic benefit from realignment of the eyes, the potential for more disabling diplopia exists as the images are brought closer together. At this point an occlusive contact lens can be used to achieve a reasonable cosmetic and functional result.
Considerations in Children With Third Nerve Palsies
Third nerve palsies in childhood may be congenital or acquired ( Table 15.3 ). Almost every pediatric series has shown that the most common cause of an acquired third nerve palsy in this age group is trauma. Neoplasms, inflammatory disorders, and ophthalmoplegic migraine should also be considered. Isolated third nerve palsies due to posterior communicating aneurysms and vasculopathic causes are rare in children.
Miller 1977 (1951–1976) | Harley 1980 (1968–1979) | Keith 1987 (1974–1985) | Ing 1992 (1970–1991) | Kodsi 1992 (1966–1988) | Schumacher-Feero 1999 (1981–1996) | CHOP 2010 (1993–2006) | |
---|---|---|---|---|---|---|---|
Congenital | 13 | 15 | 8 | 8/(3) | Not included | 20 | 17/(2) |
Trauma | 6 | 4 | 7 | 20/(11) | 14 | 15 | 24/(13) |
Neoplasm | 3 | 3 | 1 | 1/(1) | 5 | 6 | 17/(6) |
Inflammatory/ infectious | 4 | 3 | 6 | 6/(1) | 1 | 3 | 9/(6) |
Migraine | 2 | 3 | 1 | 2 | 3 | 2 | |
Aneurysm | 2 | 0 | 0 | 0 | 0 | 1/(1) | |
Surgical | 0 | 0 | 0 | 0 | 3 | 7 | |
Idiopathic | 0 | 0 | 4 | 1 | 6 | 1 | 3 |
Other | 2 | 4 | 1 | 0 | 3 | 4 | 11/(2) a |
Total | 30 | 32 | 28 | 38/(16) | 35 | 49 | 91/(30) |
Congenital third nerve palsies . In Miller’s series of isolated third nerve palsies in pediatric patients, 43% were congenital in origin ( Fig. 15.27 ). Most had pupillary involvement, aberrant regeneration, and amblyopia, and some went on to develop the phenomenon of oculomotor paresis with cyclic spasms. Forty percent of third nerve palsies in Harley’s series were also congenital, but in other studies and in the Children’s Hospital of Philadelphia (CHOP) series, they were seen less frequently. Monocular elevator palsies were grouped as congenital third nerve palsies in some series.




Before the advent of modern neuroimaging, in most cases the responsible lesion was felt to be peripheral, due to birth trauma (skull molding) for instance, as suggested by the isolated nature of the third nerve palsy and the high frequency of aberrant regeneration. However, Balkan and Hoyt reported 10 patients with congenital third nerve palsies, of whom 4 had focal neurologic abnormalities (e.g., hemiparesis), and 2 had developmental delay. They and subsequent authors suggested that in some instances, congenital third nerve palsies may result from brainstem injury or reflect more widespread neurologic damage. Indeed, various autopsy studies have demonstrated aplasia of the ocular motor nuclei or malformation of the midbrain, reflective of an in utero insult to the central nervous system. MRI can readily confirm midbrain damage in some cases.
Miscellaneous Common Acquired Etiologies
Inflammatory (including infections) . Pneumococcal and Haemophilus influenzae meningitis have been associated with third nerve palsies in children. In addition, in the CHOP series an isolated third nerve palsy was caused by tuberculous meningitis, and combined palsies were seen in association with Lyme meningitis and Miller Fisher syndrome. A parainfectious third nerve palsy in a young child with cisternal nerve enhancement on MRI has been reported. Rarely Tolosa–Hunt syndrome occurs in childhood and can mimic ophthalmoplegic migraine (see later section).
Neoplasms . Posterior fossa tumors occur more frequently in children than in adults. However, these neoplasms tend to affect the pons and medulla, causing sixth nerve palsies more commonly (discussed later). When tumor-related third nerve palsies occur, lesions affecting the midbrain (e.g., astrocytomas or higher grade gliomas), orbit, orbital apex (e.g., rhabdomyosarcomas ), and leptomeninges may be implicated. Schwannomas, neurinomas, and meningiomas of the oculomotor nerve may also be seen in childhood, especially in patients with neurofibromatosis type II ( Fig. 15.28 ).

Ophthalmoplegic migraine . Ophthalmoplegic migraine is a rare neurologic disorder occurring in children associated with recurrent bouts of headache accompanied by ophthalmoplegia ( Fig. 15.29 ). The third cranial nerve is commonly affected, followed by the VIth and rarely the IVth nerve. The third nerve palsy in ophthalmoplegic migraine is usually pupil-involving, and complete resolution over weeks is the rule. However, aberrant regeneration and persistent deficits may be seen in half the patients. We have a seen a child who became progressively more exotropic with each attack, which occurred yearly for 3 years.



The disorder is uncommon and occurred in only 0.1% of children with migraine seen over an 18-year period in one institution, and in only 8 of 5000 migraineurs in another study. The first attack usually occurs before the child is 10 years of age, sometimes in infancy, and uncommonly after age 20 years. In a review of published cases, the mean age at the time of first attack was 8 years (range 1–74 years), and two-thirds of affected patients were females. Ipsilateral headache or periorbital pain usually precedes the ophthalmoparesis, sometimes by days, and young children often exhibit irritability and skin pallor in addition to nausea, vomiting, photophobia, and phonophobia. Attacks are usually separated by months or years, occur almost always in the same eye, and involve the same ocular motor nerve each time.
The diagnosis is one of exclusion, following neuroimaging, lumbar puncture (LP), and blood studies, but is suggested by a personal or family history of migraine. Once the individual has had several recurrent, self-limited third nerve palsies over a period of years, with normal investigations and normal health between events, the diagnosis is more obvious. Most cases are associated with enhancement of the oculomotor nerve on MRI ( Fig. 15.30 ). Whether these cases might also be considered a variant of Tolosa–Hunt syndrome is unclear. Nevertheless, the International Headache Society (IHS) now classifies ophthalmoplegic migraine under “cranial neuropathies” rather than migraine.

Etiology. Compression of the ocular motor nerves against the lateral wall of the cavernous sinus by an edematous or dilated cavernous carotid and third nerve ischemia have been proposed as mechanisms. However, given the associated enhancement of the third nerve found frequently on MRI, ophthalmoplegic migraine is now thought to be a recurrent inflammatory cranial neuropathy.
Treatment. Patients have been treated with ergotamines, corticosteroids, and propranolol, for instance. However, no abortive medication has actually been proven beneficial, as most patients’ oculomotor palsies spontaneously resolve. Prophylaxis may not be ideal in young children in whom the attacks may be separated by months or years. There are too few cases to study any drug’s efficacy formally. One approach is to treat the headache symptomatically, allow the motility deficit to improve spontaneously, and avoid prophylactic therapy.
Miscellaneous Uncommon Acquired Etiologies
Aneurysms . Intracranial aneurysms are uncommon in childhood; thus isolated third nerve palsies due to posterior communicating aneurysms are extraordinarily infrequent in this age group as well. In the pediatric series only two such patients, one aged 16 years and one aged 17 years, were reported by Miller. Younger patients have been the subject of single case reports by Gabianelli et al. (14 years old), Wolin and Saunders (11 years old), Mehkri et al. (10 years old), Branley (7 years old), and Ebner (6 years old). The first two had CT studies that missed the posterior communicating aneurysm, which was later confirmed on conventional angiography.
Third nerve palsies due to other intracranial aneurysms are even more rare. These include those due to giant cavernous carotid aneurysms and aneurysms of the congenital distal basilar artery, superior cerebellar artery, posterior cerebral artery, and internal carotid artery.
Diabetes . Diabetic cranial mononeuropathies are very rare in children. Grunt et al. reported three such patients, but each had features which were atypical. A 12-year-old boy had a purported sixth nerve palsy, but an ophthalmologist diagnosed hyperopia with accommodative esotropia, and this coincided with temporary hyperglycemia. The “left internal strabismus” in this patient improved when the refractive error and insulin requirement resolved. The second patient was a 13-year-old child with a complete, pupil-sparing third nerve palsy, which did not resolve fully after 21 months of follow-up. The third patient was an 11-year old with a partial, pupil-involving third nerve palsy which lasted 16 months until strabismus surgery was performed.
Diagnostic evaluation in children with third nerve palsies . One suggested approach is outlined in Box 15.2 . In children with congenital third nerve palsies present since birth, a careful neurologic examination should be performed, looking for other associated neurologic abnormalities. Then an MRI of the brain should be ordered to exclude congenital brain malformations.
- 1.
Congenital
- a.
Careful neurologic examination
- b.
MRI of the brain
- a.
- 2.
Acquired
- a.
Exclude history of trauma or migraine
- b.
MRI and MRA
- c.
Consider lumbar puncture, if the neuroimaging is negative
- d.
If MRI and LP negative, consider ophthalmoplegic migraine
- e.
If MRI and LP negative in a patient 10 years of age or older, consider conventional angiography
- a.
LP, Lumbar puncture; MRI, magnetic resonance imaging; MRA, magnetic resonance angiography.
When the third nerve palsy is acquired, a history of trauma or migraine should be excluded. In all acquired cases, MRI and CTA or MRA should be performed, looking for mesencephalic, cavernous sinus, or orbital lesions and to exclude an aneurysm. We do not believe that children with acquired, isolated third nerve palsies can be watched conservatively. If the neuroimaging is negative and the child is ill-appearing or has a fever or stiff neck, a LP should be performed to exclude meningitis. If the MRI and LP are negative, a diagnosis of ophthalmoplegic migraine can be entertained. Finally, if the MRI and LP are negative in a patient 10 years of age or older, practitioners should consider conventional angiography to exclude a posterior communicating aneurysm.
Fourth Nerve Palsies
Patients with superior oblique paresis complain of vertical diplopia, often with a torsional component, and they have an ipsilateral hypertropia worse on contraversive (contralateral conjugate) horizontal gaze and ipsilateral head tilt (Parks–Bielchowsky three-step test, best demonstrated with alternate cover or Maddox rod testing) ( Fig. 15.31 , and ). In some instances the superior oblique weakness is evident on testing of ductions ( Fig. 15.32 ), but more commonly alternate cover or Maddox rod testing is necessary to document the misalignment. Excyclotorsion of the involved eye may be documented using double Maddox rods. Head tilt toward the contralateral shoulder is usually seen (see Fig. 15.32 ). One can occasionally elicit which fourth nerve is affected by asking the patient to look at a horizontal straight edge such as a door, projector screen, or pen. If the patient sees two images, they can be asked to describe how they intersect. A patient with a unilateral fourth nerve palsy will see a horizontal line and a tilted line below it, intersecting on the side of the abnormal eye (“the arrow points to the affected fourth nerve”).









Trauma is one of the most common causes of acquired fourth nerve palsies (see Table 15.1 ). Traumatic fourth nerve palsies have been attributed to damage to the posterior decussation (anterior medullary vellum) due to its close anatomic relationship with the tentorium. Contusions in this area may be seen on neuroimaging. This is the likely explanation for the high incidence of bilateral fourth nerve palsies in trauma. Alternatively, hemorrhages of the fourth nerve more laterally at the tentorial edge have been documented. In addition, skull base fractures may be responsible for traumatic fourth nerve palsies.
Decompensated congenital fourth nerve palsies are also common and are discussed in more detail later. Ischemic fourth nerve palsies should be considered when an individual older than 50 years with vasculopathic risk factors develops vertical diplopia and a periorbital ache. In such cases the prognosis for spontaneous recovery is excellent. An emergency room study found ischemia to be the most common etiology among patients presenting with acute isolated IVth nerve palsies. Schwannomas ( Fig. 15.33 ), neurinomas and anterior temporal lobectomy for epilepsy are also recognized causes of fourth nerve palsies. Aneurysmal causes of fourth nerve palsies, however, are much less common than in third nerve weakness. Other less frequent causes of fourth nerve palsies include compressive and infiltrative neoplasms, meningitis, multiple sclerosis, posterior midbrain hemorrhages and strokes ( Fig. 15.34 ), vascular malformation, and giant cell arteritis.


Occasionally fourth nerve palsies are accompanied by other neurologic signs that aid in localization. For instance, a fourth nerve palsy accompanied by a contralateral Horner syndrome would localize to the dorsal pontomesencephalic junction (see Fig. 13.21 ). A fourth nerve palsy with contralateral dysmetria suggests a lesion involving the superior cerebellar peduncle, while one accompanied by contralateral INO localizes to the proximal fascicle and MLF. Rarely, a fourth nerve palsy can combine with a relative afferent pupillary defect due to interruption of pupillary axons traveling through the brachium of the superior colliculus. The relative afferent pupillary defect in this instance is unassociated with optic neuropathy.
Decompensated congenital fourth nerve palsies . Most of these patients present with head tilt or insidious onset of intermittent vertical double vision or focusing difficulty. A large hypertropia in adduction of the affected eye is sometimes noticed by the patient or family members. Complaints of torsion are less than in cases of acquired fourth nerve palsies. Evidence of chronicity includes overaction of the ipsilateral inferior oblique muscle (see Fig. 15.32 ) and a large vertical fusional amplitude. The latter refers to the patient’s ability to fuse vertically separated images despite the placement of progressively large amounts of vertical prism over one eye. Facial asymmetries are also frequently associated with the long-standing head tilt ( Fig. 15.35 ). Chronicity can also be established by documenting a long-standing head tilt, as evidenced by review of old photographs and a family member’s report, and the absence of an inciting event. Decompensated congenital fourth nerve palsies are likely the result of progressive breakdown in vertical fusional capability rather than progression of fourth nerve weakness.

Proposed etiologies for congenital fourth nerve palsies include hypoplasia or aplasia of the fourth nerve nucleus, birth trauma to the fourth nerve or superior oblique muscle, anomalous insertion of the muscle, muscle fibrosis, fibrous adhesions, or structural abnormalities of the superior oblique tendon. Usually congenital cases are sporadic. However, familial cases and those associated with central nervous system abnormalities have been reported. Jiang et al. found ARIX gene polymorphisms in patients with congenital superior oblique palsy. Because the ARIX gene is expressed in the brainstem nuclei of oculomotor and trochlear nerves, this genetic association suggests that some cases of congenital superior oblique palsy might be the result of a cranial dysinnervation syndrome.
The pathogenesis of congenital IVth nerve palsy has also been studied by neuroimaging. In one MRI study, 73% of children with a congenital IVth nerve palsy had radiologic absence of the trochlear nerve and a small atrophic superior oblique muscle. Patients with an absent trochlear nerve had higher degrees of head tilt, facial asymmetry, and vertical misalignment. When the trochlear nerve was present, there were higher degrees of inferior oblique overaction.
Bilateral fourth nerve palsies . The etiology of bilateral fourth nerve palsies is age dependent. In infancy, most cases are congenital, while in adulthood they are mostly due to head injury ( Fig. 15.36 ) or rarely neoplasm. The presence of bilateral fourth nerve palsy may also be discovered after strabismus surgery for a presumed unilateral palsy as an operation on that eye may uncover a fourth nerve palsy in the other eye (masked bilateral fourth nerve palsy). Bilateral fourth nerve palsies may be suggested by an alternating hypertropia in lateral gaze, high degrees of excyclotorsion, and a V-pattern esotropia.


Diagnostic Evaluation of Adults With Acquired Fourth Nerve Palsies
Without a history or clinical features of a long-standing decompensated congenital palsy, patients with fourth nerve palsies should undergo neuroimaging to exclude an intracranial process. In addition, mimickers such as myasthenia gravis, thyroid-associated ophthalmopathy, and skew deviation should be excluded.
Management of Fourth Nerve Palsy
The management of fourth nerve palsy involves temporary occlusion, prisms, and surgery. Some patients can be treated with a base-down prism over the affected eye, and usually press-on Fresnel prisms are used before permanent ones are ground into the lenses. High satisfaction with prismatic correction has been reported in patients with decompensated congenital IVth nerve palsy. However, excyclotorsion and incomitance of the deviation may leave some patients unsatisfied with prisms. Others, particularly those with ischemic palsies, in whom recovery is expected, can occlude the lower half of the lens over the affected eye. Opaque or black tape, for instance, can be used to for this purpose and provides occlusion of the affected eye in the direction of action of the superior oblique muscle.
Patients with persistent fourth nerve palsies who are unsatisfied with prisms or patching may require strabismus surgery. The decision to intervene surgically is based on the presence of increasing symptoms of double vision and an inability to carry on normal activities combined with stable measurements over a period of several months.
Fourth Nerve Palsies in Children
In many children, minor superior oblique paresis is harder to detect, especially when the third nerve is also involved. In Harley’s and Kodsi and Younge’s series, trauma was the most common cause of an acquired fourth nerve palsy ( Table 15.4 ). However, in Harley’s and the CHOP series, congenital or decompensated congenital fourth nerve palsies were the most common of all cases.
Harley 1980 (1968–1979) | Kodsi 1992 (1966–1988) | CHOP 2010 (1993–2006) | |
---|---|---|---|
Congenital | 12 | Not included | 28 |
Trauma | 5 | 7 | 3/(4) |
Neoplasm | 0 | 1 | 1/(3) |
Inflammatory | 1 | 1 | 1/(3) |
Surgical | 0 | 1 | 2 |
Idiopathic | 0 | 4 | 0 |
Other | 0 | 5 | 2/(1) a |
Total | 18 | 19 | 37/(11) |
a Elevated intracranial pressure, cavernous carotid aneurysm.
Diagnostic evaluation in children with fourth nerve palsies . If the fourth nerve palsy is consistent with an isolated, decompensated congenital one, then no workup is necessary. Otherwise, head trauma and evidence for a posterior fossa mass should be excluded. If the etiology is unclear, an MRI can be performed, with attention to the posterior midbrain, cavernous sinus, and orbit. In children with a normal MRI but with a fever or stiff neck, an LP should be performed to exclude meningitis.
Sixth Nerve Palsies
Patients with a lateral rectus palsy complain of binocular horizontal double vision worse on ipsiversive gaze and at distance. In many cases the limitation of abduction is evident ( Fig. 15.37 ), but, in more subtle instances, alternate cover or Maddox rod testing would confirm an esotropia largest on ipsiversive gaze. Abducens nerve palsy is a relatively common neuro-ophthalmic disorder that has a wide range of causes in both pediatric and adult populations. Its long intracranial course, tortuosity, and tight attachment to the skull base contribute to the nerve’s vulnerability.


Nuclear/fascicular . A lesion in the region of the sixth nerve nucleus would result in an ipsiversive conjugate gaze palsy and ipsilateral facial weakness (facial colliculus syndrome; see Chapters 14 and 16 and Fig. 16.5 ). A lesion of the caudal ventral pons involving the abducens fascicle and corticospinal tract would result in a lateral rectus palsy and a contralateral hemiparesis (Raymond syndrome). However, isolated sixth nerve palsies due to pontine lesions may be seen in hypertension or diabetes. Embolic or thrombotic occlusion of paramedian penetrating branches of the basilar artery is the usual cause for disturbances in these areas, but multiple sclerosis, neuromyelitis optica, vascular malformations, and metastases should also be considered.
Subarachnoid/base of skull . The sixth nerve, as it climbs along the clivus then over the petrous apex, is vulnerable to injury during downward brainstem shifts resulting from supratentorial masses (“false localizing sign”). Changes in intracranial pressure that occur in pseudotumor cerebri, supratentorial masses ( Fig. 15.38 ), or hydrocephalus (intracranial hypertension) or after LP, myelography, epidural spinal catherization, or CSF leak (intracranial hypotension) may also cause sixth nerve palsies. These tend to resolve within days or weeks after the intracranial pressure is normalized.

Skull base neoplasms involving the parasellar, petrous apex, and cavernous sinus regions such as meningiomas and chordomas (see below) are common causes of chronic isolated sixth nerve palsies. Sixth nerve palsies in this setting may sometimes improve spontaneously and therefore mimic inflammatory and ischemic palsies. Isolated acute sixth nerve palsy can occur in association with pituitary apoplexy. Since the parasympathetic fibers of the facial nerve travel along the floor of the middle cranial fossa on their way to supply the ipsilateral lacrimal gland, a patient with a sixth nerve palsy and ipsilateral dry eye may be harboring a mass in this region.
Otherwise, the same infectious and inflammatory processes in the subarachnoid space which affect the third nerve can also cause a sixth nerve palsy. Similarly, trauma and ischemia can lead to sixth nerve palsies. As with other traumatic ocular motor palsies, the trauma tends to be severe and accompanied by skull fractures ( Fig. 15.39 ). The prognosis for spontaneous improvement within weeks or months is excellent, but patients with complete palsies at presentation have a poorer chance for recovery. Ischemic sixth nerve palsies are typically painful, have relatively sudden onset, and resolve within 3 months. Benign, idiopathic sixth nerve palsies which spontaneously resolve in young adults are also well recognized (see Fig. 15.37 ). Guillain–Barré syndrome, Miller Fisher syndrome, and Gradenigo’s syndrome should also be in the differential diagnosis of sixth nerve palsies. Other less common causes include schwannomas, giant cell arteritis, and compression of the sixth nerve by dolichoectatic basilar, vertebral, or carotid arteries.



Diagnostic Evaluation in Adults With Acquired Sixth Nerve Palsies
As in adults with other acquired ocular motor palsies, we prefer to use MRI for patients with acquired sixth nerve palsies to exclude both intraaxial and extraaxial intracranial processes. In younger adults, neuroimaging is particularly important because of the relatively higher risk for neoplasms in this age group. Serologies, including testing for syphilis and Lyme disease, as well as a sedimentation rate to exclude giant cell arteritis, may be performed in appropriate settings. Lumbar puncture may be necessary when infectious, carcinomatous, or lymphomatous meningitis are suspected.
Management of Sixth Nerve Palsies
Once again, the options that exist acutely include occlusion and press-on Fresnel prisms. Base-out prisms can be used to significantly reduce the head turn and expand the field of single binocular vision while the patient is being followed for recovery. Alternatively, opaque or black tape can be placed over the temporal half of the lens over the affected eye. This occludes the affected eye in the direction of the lateral rectus palsy. In addition, botulinum injections to the ipsilateral medial rectus muscle can be helpful in restoring a field of single binocular vision and potentially reducing the risk of medial rectus contracture, although some studies have reported ineffective outcomes after botulinum toxin injections. Moreover, botulinum toxin injections may be limited by the side-effect of ptosis and the need for repeated injections. Once the sixth nerve palsy has been followed for 6–12 months without recovery, the patient can be offered strabismus surgery.
Sixth Nerve Palsies in Children (Acquired)
In three pediatric series, trauma was the most common cause of an acquired sixth nerve palsy. In the series by Robertson et al., Aroichane and Repka, , CHOP, and Merino, neoplasms were the most frequent cause. Thus, accounting for the results from all series, the most common cause of acquired, nontraumatic sixth nerve palsy is a tumor ( Table 15.5 ). Separating sixth nerve palsies by etiology is often difficult because of the considerable overlap: Trauma, neoplasms, and meningitis can be associated with elevated intracranial pressure or hydrocephalus, which by themselves are independent causes of sixth nerve palsies.
Robertson 1970 (1952–1964) | Harley 1980 (1968–1979) | Afifi 1992 (1966–1988) | Kodsi 1992 (1966–1988) | Aroichane 1995 (1985–1993) (Age <7) | CHOP 2010 (1993–2006) | |
---|---|---|---|---|---|---|
Congenital | Not included | 5 | 17 | Not included | Not included | 10/(2) |
Trauma | 26 | 21 | 37 | 37 | 12 | 23/(13) |
Neoplasm | 52 | 17 | 25 | 18 | 21 | 58/(6) |
Surgical | 34 | |||||
Inflammatory/infectious | 23 | 8 | 13 | 5 | 4 | 23/(6) |
Increased ICP (nontumor) | 15 | 3 | 17 | 2 | 15 | 25/(1) |
Idiopathic | 12 | 4 | 14 | 13 | 3 | 26 |
Other | 5 | 4 | 9 | 13 | 9 | 11/(2) a |
Total | 133 | 62 | 132 | 88 | 64 | 210/(30) |
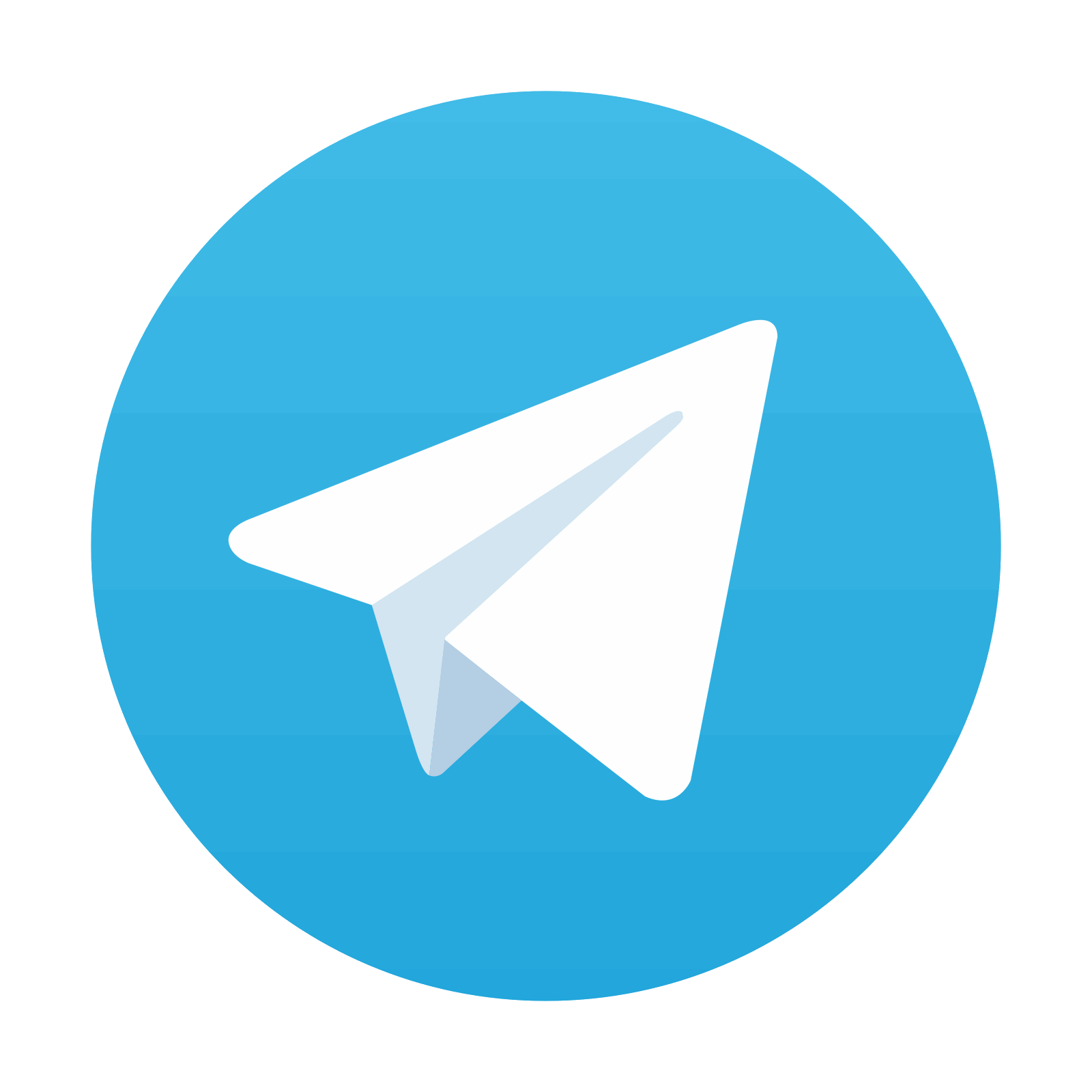
Stay updated, free articles. Join our Telegram channel

Full access? Get Clinical Tree
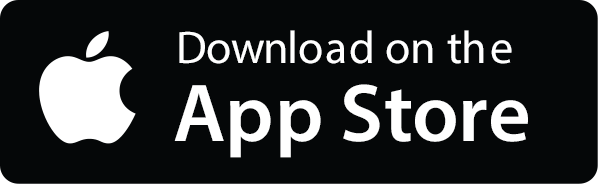
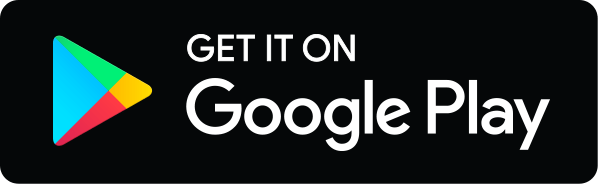
