Key points
- •
The fibrocartilaginous eustachian tube is part of a system of contiguous organs including the nose, palate, rhinopharynx, and middle ear cleft.
- •
It is not a simple tube, but a complex organ consisting of a dynamic conduit with its mucosa, cartilage, surrounding soft tissue, peritubal muscles, and superior bony support (the sphenoid sulcus).
- •
The authors believe that the principal roles of the fibrocartilaginous eustachian tube could be the optimization of middle ear sound transmission and protection of the inner ear structures.
General introduction
The fibrocartilaginous eustachian tube is part of a system of contiguous organs including the nose, palate, rhinopharynx, and middle ear cleft. The middle ear cleft consists of the tympanic cavity, which includes the bony eustachian tube (protympanum) and the mastoid gas cells system ( Fig. 1 ). The tympanic cavity and mastoid gas cells are interconnected and allow gaseous exchange and pressure regulation.
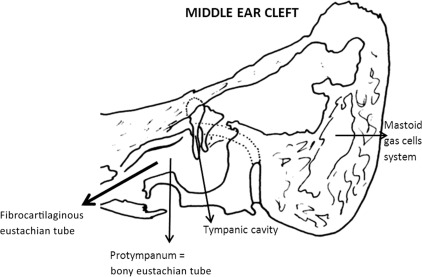
The fibrocartilaginous eustachian tube is not a simple tube, but a complex organ consisting of a dynamic conduit with its mucosa, cartilage, surrounding soft tissue, peritubal muscles (ie, tensor and levator veli palatine, salpingopharyngeus and tensor tympani), and superior bony support (the sphenoid sulcus).
The authors think that the principal roles of the fibrocartilaginous eustachian tube could be the optimization of middle ear sound transmission and protection of the inner ear structures. These roles are aided by maintenance of the indispensable balance of pressure variations within the middle ear cleft, relative to ambient pressures. Given that the fibrocartilaginous eustachian tube links the protected sterile environment of the middle ear cleft to the potentially hostile outside world, it must provide 3 additional specific organic functions, as follows:
- •
Mechanical protection against rhinopharyngeal secretions and pathogenic microorganism reflux, as well as against retrograde propagation of vocal sounds
- •
Local immune defense
- •
Mucociliary clearance into the rhinopharynx of secretions produced in the middle ear cleft and carried away in its inferior part.
Tubal function is an intrinsic and important component of the middle ear cleft pressure regulation system.
General introduction
The fibrocartilaginous eustachian tube is part of a system of contiguous organs including the nose, palate, rhinopharynx, and middle ear cleft. The middle ear cleft consists of the tympanic cavity, which includes the bony eustachian tube (protympanum) and the mastoid gas cells system ( Fig. 1 ). The tympanic cavity and mastoid gas cells are interconnected and allow gaseous exchange and pressure regulation.
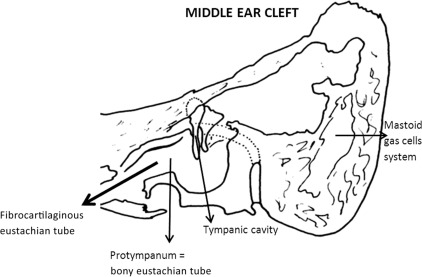
The fibrocartilaginous eustachian tube is not a simple tube, but a complex organ consisting of a dynamic conduit with its mucosa, cartilage, surrounding soft tissue, peritubal muscles (ie, tensor and levator veli palatine, salpingopharyngeus and tensor tympani), and superior bony support (the sphenoid sulcus).
The authors think that the principal roles of the fibrocartilaginous eustachian tube could be the optimization of middle ear sound transmission and protection of the inner ear structures. These roles are aided by maintenance of the indispensable balance of pressure variations within the middle ear cleft, relative to ambient pressures. Given that the fibrocartilaginous eustachian tube links the protected sterile environment of the middle ear cleft to the potentially hostile outside world, it must provide 3 additional specific organic functions, as follows:
- •
Mechanical protection against rhinopharyngeal secretions and pathogenic microorganism reflux, as well as against retrograde propagation of vocal sounds
- •
Local immune defense
- •
Mucociliary clearance into the rhinopharynx of secretions produced in the middle ear cleft and carried away in its inferior part.
Tubal function is an intrinsic and important component of the middle ear cleft pressure regulation system.
Tubal mechanics
The proximal one-third of the tubal length is a bony funnel-shaped extension of the middle ear cleft, the protympanum, is narrowest at the isthmus.
The distal two-thirds of the tubal length is the pharyngeal portion, which is composed of a cartilaginous skeleton to which is attached a complex arrangement of peritubal muscles capable of a wide range of dynamic movements.
This cartilaginous portion is normally closed in the resting position because of apposition of the mucosal walls. Closure occurs over a variable length (5–10 mm) just a few millimeters distal to the bony isthmus where the cartilaginous skeleton becomes flexible.
This portion that intermittently dilates to the open position is termed the functional valve because this describes its purpose.
Contraction of the levator veli palatine muscle raises the soft palate and medially rotates the medial cartilaginous lamina. Contraction of the tensor veli palatine muscle tenses the anterolateral membranous wall and distracts it laterally to dilate the tubal valve into the open position.
Intermittent brief tubal dilation is most likely the principal mechanism for equilibration of middle ear cleft pressure with the ambient atmosphere. Involuntary dilation of the fibrocartilaginous eustachian tube occurs throughout the day, typically occurring with a swallow or yawn, but it does not accompany every swallow or yawn. Barometric and chemical receptors within the middle ear cleft are thought to provide autonomic nervous system feedback that influences the frequency of involuntary tubal opening.
Tubal dilation occurs in normal subjects approximately 1.4 times per minute during daytime, with the duration of opening averaging 0.4 seconds. During sleep, the frequency of tubal opening is substantially reduced.
Sequential muscular contractions initiate rotational movements of the cartilaginous framework and create tension within the anterolateral wall, which causes the effacement of its resting bulge. This effacement is the primary action that opens the lumen to the middle ear cleft.
Tubal dilation begins with the action of the levator veli palatine muscle to medially rotate the cartilaginous skeleton, primarily its mobile distal half of the medial cartilaginous lamina. Tensor veli palatine muscle contraction follows, causing the resting convexity of the anterolateral wall to become effaced or even concave as the final step to transiently open the lumen.
It is thought that intermittent brief dilation of the tube is the principal mechanism for equilibration of middle ear cleft pressure with the ambient atmosphere. Middle ear cleft gas exchange is an ongoing process that continually generates a net absorption of gases resulting in an increasingly negative pressure between tubal dilations. There is a gradient of partial pressures of gases between the middle ear cleft gases and the venous capillary soluble gases that drives the net absorption of gas into the circulation over time.
CO 2 has the highest coefficient of diffusion and is most rapidly exchanged with the net exchange passing from the venous blood to the middle ear cleft. The next most rapid diffusion occurs with O 2 , passing from the middle ear cleft into the blood. Diffusing much more slowly into the circulation is N 2 , the predominant partial pressure in both air and blood and the source of the largest gradient. It is the slow absorption of N 2 that causes the increasing relative vacuum between ambient air and the middle ear cleft over time.
Intermittent dilation with opening of the middle ear cleft may principally serve to regulate nitrogen balance.
Tubal dilation is likely naturally facilitated by the presence of surface tension–reducing substances that are found in its mucus. Surfactants are produced within the tubal mucosa and probably aid in reducing the surface tension of the lumen, which reduces the work required to dilate the tube.
Fluid and secretions in the middle ear cleft are cleared by a combination of the muscular pumping action that occurs with the tubal closing process and by mucociliary activity. Reflux of rhinopharyngeal secretions into the middle ear cleft is limited or prevented by the closed position of the resting pharyngeal fibrocartilaginous eustachian tube and by the trapped volume of gas in the tympanic cavity and mastoid gas cells system, which creates a gas cushion. Reflux of the sounds of breathing and vocalization are similarly blocked by the closed resting position of the pharyngeal eustachian tube.
The place of tubal physiology in pressure balancing of the middle ear cleft morphofunctional partition
From the quantitative point of view, gas spaces of the middle ear cleft constitutes most of the pneumatic spaces of the temporal bone. The middle ear cleft consists of both the mastoid gas cells system and the tympanic cavity (see Fig. 1 ). The latter comprises the tympanum and 4 annexes: the epitympanum, hypotympanum, retrotympanum, and protympanum, which correspond with the bony eustachian tube. The middle ear cleft consists of a set of interconnected gas cells lined with the same respiratory mucosa. Because the gas exchange is performed through the mucosa of the cells, the total surface area of mucosa influences the rate of gas exchange.
The tympanic cavity is constricted in its superior third by the interatticotympanic diaphragm, which is a bony membranous barrier perforated by 2 small permanent openings: the anterior tympanic isthmus, which is situated between the tensor tympani tendon and the stapes; and the posterior tympanic isthmus, which is between the double posterior ligament of the incus and the bony posterior tympanic wall.
This anatomic barrier divides the middle ear cleft into 2 separate compartments: an anteroinferior compartment, principally devoted to the mucociliary clearance function, and a posterosuperior compartments, more devoted to the gas exchange function. The barrier forms a diaphragm that is composed of 2 complementary types of structure: mucosal folds and bones with muscular and ligament structures, namely the head and neck of the malleus, the body and short process of the incus, the tensor tympani muscle, the anterior and lateral mallear, and the double posterior incudal ligaments ( Fig. 2 ). This concept of partition makes it easier to understand the mechanisms involved in the pathogenesis of otitis media. Clinical as well as surgical management is thereby enhanced.
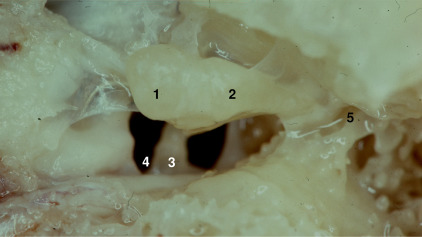
The anteroinferior compartment of the middle ear cleft, which is situated under the diaphragm, includes the protympanum, mesotympanum, and hypotympanum, and is covered by secretory or nonsecretory ciliated cells that enable mucociliary clearance. It consists of a less rigid chamber because of the presence of the eardrum. Because of the fibrocartilaginous eustachian tube, it opens into an intermittently ventilated gas pocket. It communicates with the posterosuperior compartment by both the anterior and posterior tympanic isthmi. It is often the site of secondary bacterial infections from the rhinopharynx. An inflammatory process involving the mucosa of the anteroinferior middle ear cleft compartment leads to dysfunction in mucociliary clearance and to the accumulation of mucus ( Fig. 3 ).
The posterosuperior compartment of the middle ear cleft, which is situated above the diaphragm, includes the epitympanum and retrotympanum, aditus ad antrum, antrum, and mastoid gas cells system. It is covered by a richly vascularized cuboidal epithelium that is devoted primarily to gas exchange. It consists of a rigid chamber and an open nonventilated gas pocket that communicates with the anteroinferior compartment via both openings. It may be the site of viral hematogenous infections. Inflammation of the mucosa of the posterosuperior middle ear cleft compartment impairs the gas exchange, which in turn leads to the development of a gas deficit in the middle ear cleft (compare with the peripheral and central nervous regulating system, as discussed later). The interatticotympanic diaphragm conditions the topography of the tympanic membrane retraction pockets. When only 1 opening is blocked, the posterosuperior quadrant of the pars tensa is attracted in the direction of the retrotympanum. When both openings are completely blocked, the pars flaccida is drawn in toward the epitympanum. In the same way, the diaphragm also influences the invasion of the middle ear cleft by a cholesteatoma.
What, then, is the role of the mastoid? The physiology of the mastoid can only be understood in the context of balancing pressure variations in the middle ear cleft. The middle ear cleft is essentially a noncollapsible, poorly ventilated gas pocket through which sound wave energy is transported to reach the inner ear. All gases must be contained in a three-dimensional container or volume (V). The pressure (P) of a gas is created by the molecules of gas striking the walls of the container. It is a force per unit of area. The physical characteristics of a gas are linked by the ideal gas law: PV = nRT, in which n is the amount of gas expressed in moles, R is the universal gas constant, and T is the absolute temperature. The middle ear cleft is submitted to physiologic variations in volume and pressure. It has the capacity to maintain a steady state in volume and pressure, which is achieved by various regulatory mechanisms that neutralize or minimize the pressure and volume variations by adjusting the quantity of gas, its diffusion, and/or the volume of the middle ear cleft.
Gas Exchanges
The gas content of the middle ear cleft is constituted and maintained by gas exchanges between the middle ear cleft and the neighboring structures, including the environment across the tympanic membrane, the inner ear via the round window membrane, the blood compartment via the mucosa, and the rhinopharynx through the fibrocartilaginous eustachian tube.
The gases that are present in the middle ear cleft are identical to those found in the blood and in the atmosphere: oxygen (O 2 ), carbon dioxide (CO 2 ), nitrogen (N 2 ), argon (Ar), and water vapor (H 2 O). Their relative amounts may be expressed by their partial pressure P(x). Nitrogen is neither produced nor consumed at the level of the middle ear cleft. The P(O 2 ) in the middle ear cleft is slightly lower and the P(CO 2 ) is slightly higher than in venous blood. This difference means that there is a modest consumption of O 2 and production of CO 2 in the middle ear cleft. Oxygen and nitrogen are absorbed from the middle ear cleft via the mucosa into the blood compartment. Carbon dioxide and water vapor are diffused from the blood compartment, via the mucosa, into the middle ear cleft.
The steady exchange of gas through the mucosa depends on the functional properties of the cells of the mucosa; the specific diffusion rate of the gas, which is a constant value for a particular gas; and the behavior of the vascular system. The primary purpose of the mucosa is to facilitate gas exchange between the middle ear cleft and the blood compartment through a constitutive tissular barrier.
To vibrate in an optimal manner, the tympano-ossicular system must remain in balance, which means that the intra–middle ear cleft pressure must be equivalent to the atmospheric pressure (760 mm Hg). In ambient air, this equivalent value is obtained by calculating the sum of the partial pressures of the 4 main constitutive gases in the air: oxygen (158 mm Hg), carbon dioxide (0.3 mm Hg), nitrogen (596 mm Hg), and water vapor (5.7 mm Hg).
However, in the middle ear cleft, the composition of gas varies for 2 main reasons. The middle ear cleft is a closed cavity that is connected with the nasal cavities by the fibrocartilaginous eustachian tube. The gas in the rhinopharynx, which enters the middle ear cleft via the tube, consists of exhaled gas that contains less oxygen and more carbon dioxide than ambient air. This gas composition also varies because gas diffusion occurs between the middle ear cleft and the arterial and venous blood, via the mucosa. In blood, the partial pressures of these gases differ. There is more oxygen in the arterial blood (93 mm Hg) and more carbon dioxide in the venous blood (44 mm Hg) because the gas exchange occurs at the level of the pulmonary air cells and the tissues throughout the body, respectively.
Thus, the composition of gas in the middle ear cleft differs from that in ambient air. The gradient out from middle ear cleft to the capillaries is 57 mm Hg for oxygen, and the gradient in from the capillaries to the middle ear cleft is 39 mm Hg for carbon dioxide. These gradients should result in a negative pressure in the middle ear cleft. However, in spite of the difference in gas composition, the pressure in the middle ear cleft approximates the atmospheric pressure to enable optimal sound transmission.
The reason for this balance is 3-fold:
- •
Because the sum of the partial pressures of oxygen and carbon dioxide is lower in the middle ear cleft (90 mm Hg) than in ambient air (150 mm Hg), nitrogen exerts the higher partial pressure in the middle ear cleft (623 mm Hg) because of its slow diffusion toward the capillaries.
- •
The composition of the exhaled gas that enters the middle ear cleft via the tube contains less oxygen and more carbon dioxide than ambient air, and this reduces the passive diffusion of these gases through the mucosa.
- •
The blood flow through the middle ear cleft mucosa is probably low, which limits the importance of gas diffusion and enables the oxygen and carbon dioxide partial pressures to nearly equalize, because each has a high diffusion rate. The same mechanism applies to water vapor, for which the diffusion rate is also very high. Transmucosal gas exchange results in gas absorption.
Regulating Systems
The steady state in volume and pressure into the middle ear cleft is preserved by 2 types of regulating mechanisms: the in situ adaptation systems, and both central and peripheral nervous regulating systems with retrocontrol between the middle ear cleft and the muscles of the fibrocartilaginous eustachian tube.
The in situ adaptation systems
The in situ adaptation systems consist mainly of the compliance of the tympanic membrane lamina propria, the fibrocartilaginous eustachian tube function, and the behavior of the vascular system of the middle ear cleft mucosa, especially that of the mastoid.
The compliance of the tympanic membrane lamina propria
The compliance of the tympanic membrane lamina propria is used in sudden pressure changes (eg, altitude, flight, diving, explosions) This compliance occurs largely because of the viscoelastic properties of the lamina propria and the flexibility of the incudomalleolar joint, which acts as a static pressure receptor for the tympanic membrane, ensuring three-dimensional movement into the malleus. Deformation of the eardrum causes a volume change of the semirigid middle ear cleft and can therefore compensate partially for fast pressure changes. The eardrum is an active pressure buffer but also a passive pressure victim.
The fibrocartilaginous eustachian tube function
The fibrocartilaginous eustachian tube is closed most of the time, and it therefore does not function as a ventilation hole, which keeps pressure inside the middle ear cleft equal to the outside. Only at large pressure differences, more than 2 kPa, does the fibrocartilaginous eustachian tube open spontaneously.
Changes in position seem to affect the function of the fibrocartilaginous eustachian tube. The mean volume of gas passing through the tube in the upright position is reduced by one-third when the body is elevated at 20° to the horizontal position. It is reduced by two-thirds in the horizontal position. This difference is the result of the increase in the venous tissular pressure around the fibrocartilaginous eustachian tube.
The vascular system in combination with the mastoid gas cell system
The behavior of the vascular system plays an important regulatory role in the physiologic balance of pressure variations in the middle ear cleft. Variations in the middle ear cleft blood flow, associated with variations in the permeability of the vessels, allow ample adaptation to normal gas pressure fluctuations. The normal extensive variations regularly observed in middle ear cleft pressure over a 24-hour period are related to the vascular adaptations required by body position and sleep. The mastoid gas cell system constitutes the most important volume of the middle ear cleft and therefore represents the major part of the middle ear cleft mucosal area available for vascular gas exchange.
The amount of gas present in the mastoid is important in regulating middle ear cleft pressure for 2 reasons. First, the physical, anatomic, properties of mastoid volume affect compliance: the greater the volume, the more compliant the system. Second, the surface area affects mucosal gas exchanges. Both are important in the regulation of middle ear cleft pressure. The gas contained in the mastoid gas cells system acts as a physiologic passive pressure buffer.
The size of the mastoid gas cells system varies greatly between individuals. However, whatever the cause, be it genetic determination of growth or environmental factors, there is unanimous agreement that the small mastoid gas cell system has a pathologic association. The smaller the system, the faster the deviation from normal pressures. However, nature is a matter of balance. It is not a question of determining the volume of the mastoid in order to predict its clinical potential. In normal conditions and with healthy mucosa, a small mastoid in an adult is normal in that it constitutes the complete outcome of normal and standard development (ie, the individual simply possesses the morphologic characteristic of being small). A small mastoid in an adult is normal, in that it is sufficient for the physiologic balance of pressure variations in the middle ear cleft. This small mastoid is no more likely to develop disorder than another is. However, when the mucosa undergoes an inflammatory process, a small mastoid in an adult (eg, as an outcome of disturbed or failed development) is at a disadvantage when it is exposed to excessive variations in pressure. This mastoid is too small compared with the rest of the middle ear cleft. Fluctuations in middle ear cleft pressure in the presence of a small mastoid gas cells system result in greater forces applied to the tympanic membrane, compared with the same pressure changes in a larger mastoid gas cells system. If the expansion reservoir is too small, it cannot play its role adequately.
In normal conditions and healthy mucosa, a slight negative pressure is present in the middle ear cleft compared with the outside air, created by exchanges of gas between the cleft and the blood compartment. Gas exchanges through the mucosa of the middle ear cleft are induced by the gradient of partial pressure of gases between the cleft and the capillaries of the submucosal connective tissue. Given the capacity of gas diffusion in the middle ear cleft, partial pressures of those gases establish a balance on both sides of the mucosal barrier of the middle ear cleft. The progressive diffusion of the gases is capable of modifying the gas composition in the middle ear cleft as well as in the circulating blood. The gas that penetrates into the middle ear cleft from the rhinopharynx is not outside air. Its composition is close to exhaled air. Quantitatively, the main gas that enters the middle ear cleft is nitrogen. Nitrogen diffuses much more slowly in the blood than the other gases (35 times more slowly than CO 2 and 1.8 times more slowly than O 2 ) ( Fig. 4 ).
