Key Features
- •
Cataracts develop earlier in developing countries, and the cataract surgical rates are lower, resulting in a higher prevalence of cataracts. But improved provision of cataract surgery in some developing nations has significantly reduced cataract prevalence.
- •
Factors in cataract pathogenesis include lens protein oxidation, mitochondrial function, failure of protective mechanisms, protein modification, and abnormalities of calcium metabolism, cellular proliferation, and differentiation.
- •
An accumulation of environmental insults (e.g., ultraviolet light, toxins, drugs, and systemic diseases) results in age-related cataracts. Minor risk factors such as ultraviolet B exposure and smoking can be modified.
- •
Nutritional, pharmacological, and genetic interventions are being investigated.
- •
Anomalies of lens growth are usually associated with other ocular or systemic disorders.
Epidemiology of Cataracts
In 2010 the Vision Loss Expert Group funded by the Bill & Melinda Gates Foundation, Fight for Sight, and others calculated that cataracts caused blindness (visual acuity in the better eye of less than 3/60) in 10.6 million people and moderate to severe visual impairment (MSVI, visual acuity of between 6/18 and 3/60) in 34.4 million people. However, wide regional variations exist in the prevalence of cataracts. In North America, the prevalence of blindness and MSVI was 0.3% and 0.4%, respectively. In South Asia, the respective prevalence was 2% and 6.8%. Sub-Saharan Africa is similar. However, cataract blindness and MSVI have declined since 1990 due to better provision of cataract surgery. Once again the decline in prevalence shows wide regional variations, with the greatest decline in East Asia, Latin America, and Western Europe, where the prevalence fell by more than half. The region with the least decline was Sub-Saharan Africa.
The world’s population is increasing (predominantly in developing countries), and people will live to greater ages. So without accessible, efficient cataract surgery, the prevalence will increase. Developing countries bear an increasing burden for cataract blindness because cataracts occur earlier in life, and the incidence is higher. In India, visually significant cataract occurs 14 years earlier than in the United States, and the age-adjusted prevalence of cataract is three times that of the United States. Cataracts are the leading cause of blindness in middle- and low-income countries, accounting for 50% of blindness but cause 5% of blindness in developed countries.
Low-cost small-incision cataract surgery with lens implantation is a proven clinical strategy. The socioeconomic effect of cataract surgery is substantial. It allows people to increase their economic output to 1500% of the cost of the surgery in the first postoperative year, but if left untreated, it can result in a person being removed from work and dependent on a caregiver who is also removed from work.
The cataract prevalence is influenced by the Cataract Surgical Rate (CSR or number of cataract surgeries performed per million people per year), which varies from less than 200 to over 6000 in different regions. The CSR is determined by the effectiveness of strategies for stimulating demand from patients because of good outcomes and how well easily accessible cataract surgery is delivered.
So a high cataract prevalence rate in some developing countries is not for want of a clinical solution but partially due to ineffective implementation. For example, Sub-Saharan Africa is resource poor with low surgeon productivity (number of procedures done per year). To reduce cataract prevalence, the resource base (infrastructure, equipment, ophthalmologists, and other ophthalmic workers) must improve, as must management of the resources to build the right processes for maximal cost-effective resource utilization. Future solutions will have to address these challenges.
Pathophysiology of Cataracts
The lens transmits, filters, and focuses light onto the retina. The high refractive index and transparency of the lens is due to the high concentration and orientation of intracellular structural proteins: α, β, and γ crystallins. The anterior subcapsular layer of cuboidal lens epithelial cells are nucleated, actively divide, and account for almost all the metabolic activity of the lens. Cuboidal cells in the equatorial zone of the lens undergo oxidation and other biochemical, physiological, and structural changes. The cells elongate into lens fiber cells, lose their intracellular organelles and ability to perform metabolic functions, and form mature lens fibers. Lens fibers migrate toward the nucleus of the lens and are compacted as more fibers are formed around them, resulting in nucleosclerosis and later in opacity.
The transparency of the lens is dependent on the regular organization of the lens cells and intracellular lens proteins. The precise mechanisms by which lens proteins both prevent aggregation and maintain lens transparency are largely unknown. Genetic, metabolic, nutritional, and environmental insults and ocular and systemic diseases disrupt cellular organization and intracellular homeostasis, eventually causing light scattering and absorption, which compromise vision. Once damaged, the lens has limited means of repair and regeneration and may lose its transparency by the formation of opaque lens fibers, fibrous metaplasia, epithelial opacification, accumulation of pigment, or formation of extracellular materials. Several interlinked mechanisms for cataract formation have been proposed, and no single theory completely explains age-related cataract (the commonest form). Much is still unknown about cataractogenesis, but many of the important components are becoming clearer.
Genetics
Many inherited genetic syndromes and metabolic disorders are associated with cataracts, and at least 42 genes and loci have been found to underlie Mendelian inherited forms of isolated or primary cataract. Increasing evidence exists that several genes underlying rare forms of inherited cataract also can influence susceptibility to the much more prevalent forms of age-related cataract. These observations raise the possibility of molecular genetic links between lens development and aging. Age-related cataracts are inherited as a multifactorial or complex trait. Determining the genetics of age-related cataracts is difficult because only a small proportion of the genes involved have been identified, similar gene mutations result in different cataract phenotypes, and cataract epigenetics is complex.
Epigenetics are heritable changes in the gene expression without changes in the DNA sequence. Most genes involved in cataract formation show decreased expression. DNA methylation and other histone modifications inhibit RNA transcription, effectively silencing gene expression. For example, in age-related cataracts, the gene DNA for the alpha A-crystallin protein is hypermethylated. Therapy targeted at reversing methylation and upregulating RNA transcription may prevent or reduce lens opacities. Genes that preserve lens clarity function in diverse processes including protein synthesis (e.g., structural proteins, chaperones, and cell-cycle-control proteins) and reducing oxidative stress (e.g., glutathione peroxidases). Decreased expression reduces lens epithelial cell stress tolerance and protein synthesis. Increased gene expression also can cause lens opacities, for example, by increasing lens epithelial cell ionic transport (e.g., calcium-ATPase, which controls calcium channels) and extracellular matrix protein production. Identifying more genetic and epigenetic determinants of inherited and age-related cataracts may result in nonsurgical treatments for cataract or lifestyle interventions (e.g., diet) and may prevent or reverse cataract formation.
Cell Proliferation and Differentiation
Aqueous growth factors including fibroblast growth factor (FGF), epidermal growth factor (EGF), insulin-like growth factor (IGF), platelet-derived growth factor (PDGF), and transforming growth factor (TGF-β) promote proliferation, differentiation into lens fibers, and maturation of the lens fibers. These processes will not occur if growth factor or cytokine concentrations are incorrect. Then undifferentiated cells migrate to the posterior pole, causing posterior subcapsular cataracts.
Metabolic Disturbance and Osmotic Regulation Failure
Altered gene expression changes enzyme growth factor, membrane protein, and other protein levels, which reduces energy production; changes ion transport, calcium metabolism, and antioxidant pathways; and breaks down protective mechanisms. For example, the lens maintains high intracellular potassium and low sodium levels with the opposite extracellular concentrations via the action of the sodium–potassium ATPase pump. Pump inactivation causes increased intracellular osmolality, which results in water accumulation and light scatter.
The aqueous humor is a source of nutrients and mineral ions including calcium (Ca 2+ ). Ca 2+ is an intracellular signal that regulates many functions including the permeability of the cell membranes. The extracellular Ca 2+ concentration is 10 times the intracellular Ca 2+ concentration, which drives Ca 2+ into the epithelial cell. Low intracellular Ca 2+ levels also are maintained by intracellular organelle membrane pumps (on the endoplasmic reticulum, Golgi apparatus, and mitochondria) and by binding to complex proteins (e.g., crystallins). Extracellular Ca 2+ can be bound to the outer layer of the cell membrane. Reduced binding of Ca 2+ by membrane proteins increases cell membrane permeability and causes a rise in intracellular Ca 2+ levels, the formation of calcium oxylate crystals, binding of Ca 2+ to insoluble lens proteins, increased light scattering, and nuclear cataract formation. Increased intracellular Ca 2+ levels also affect lens epithelial cell differentiation, causing posterior subcapsular cataracts. Corticosteroids have been shown to mobilize intracellular Ca 2+ in other tissues, which can increase Ca 2+ levels. In the future, Ca 2+ -regulating drugs may be developed to prevent cataracts.
Calpains
The roles of calpains in the lens are poorly understood, but they may degrade accumulated damaged lens proteins. A lack of calpains can lead to elevated levels of damaged proteins, reduce optical performance, and cause cataract. Also, excessive stimulation of calpain activity by raised Ca 2+ levels can increase proteolysis and cause cataracts. Calpain inhibitors, therefore, could be useful in the nonsurgical treatment of cataract. However, calpain inhibitors of high molecular weight are unable to cross membranes, so have been of no therapeutic use, whereas others are poorly water soluble or are toxic to lenses.
Protein Modification
Additive modifications of lens proteins (e.g., crystallins) include methylation, acetylation, carbamylation, glycation in diabetics, and binding of ascorbate, which may be the cause of lens discoloration. These additions occur especially in disease and can alter the function or properties of a protein. Diabetes (reducing sugars), renal failure (cyanate generated from urea), aging (photooxidation products), and corticosteroid use (ketoamines) have been linked to cataracts. Additive modifications can make proteins more susceptible to photooxidation by ultraviolet (UV) light.
Subtractive modifications include cleavage by enzymes (such as calpains) of crystallin that causes precipitation of lens proteins. Cleavage of channel proteins can affect intercellular communication. Neutral modifications such as isomerization can denature proteins, affecting their function. Deamidation changes the charge and affects protein–protein interactions.
Proteins in the center of the lens are as old as the individual and are very stable, but over time they can undergo conformational changes (unfolding) that expose thiol groups, which are usually “hidden” in the folds of the protein ( Fig. 5.3.1 ). The exposed glutathione groups can be oxidized to form disulfide bonds (GSSG), causing aggregation of proteins. The conformation changes and aggregation result in scattering and absorption of light.

Oxidation
Oxidation is a key feature in the pathogenesis of most cataracts. Low oxygen levels (O 2 ) are important for maintaining a clear lens. Free radicals and other oxidants, including reactive oxygen species (ROS) and reactive nitrogen species (RNS), are derived from both endogenous sources (mitochondria, peroxisomes, endoplasmic reticulum, phagocytic cells, etc.) and exogenous sources (pollution, alcohol, tobacco smoke, heavy metals, transition metals, industrial solvents, pesticides, and certain drugs like halothane, paracetamol, and radiation). Free radicals can adversely affect nucleic acids, lipids, and proteins, altering the normal redox status and leading to increased oxidative stress and cataracts.
A steep oxygen gradient occurs from the outer part of the lens to the center. Mitochondria in the lens cortex remove most of the oxygen, thus keeping nuclear O 2 levels low. However, in older people, mitochondrial function diminishes and superoxide production by the mitochondria increases, resulting in increased nuclear oxygen and superoxide levels. As the lens ages, a lens barrier develops at approximately the cortex–nuclear interface, which impedes the flow of molecules such as antioxidants (including glutathione) into the nucleus. Unstable nuclear molecules such as peroxide (H 2 O 2 ), which are generated in the nucleus or which penetrate the barrier, therefore, cause protein oxidation. Also, a lower concentration of antioxidants exists. Decomposition of UV filters in the nucleus also produces unstable reactive molecules that bind to proteins, especially if antioxidant glutathione (GSH) levels are low. Ascorbate becomes reactive with proteins in the absence of GSH. These oxidative changes can be detected even in the earliest cataracts and are progressive. Elevated levels of superoxide H 2 O 2 in the aqueous may cause cortical cataracts, since the cortex is closest to the aqueous. Copper and iron are present in higher concentrations in cataract lenses and are involved in redox reactions, which produce hydroxyl radicals.
Defensive Mechanisms
Antioxidant enzymes and antioxidants such as ascorbate, glutathione, tocopherols, and carotenoids maintain lens proteins in the reduced state and are the primary defense mechanisms. In advanced age-related nuclear cataracts, more than 90% of protein sulfhydryl groups and almost half of all methionine residues in the nuclear proteins become oxidized. Secondary defenses include proteolytic and repair processes, which degrade and eliminate damaged proteins, UV filters, and other molecules such as glutathione reductase and free radical scavenging systems. Failure of these protective mechanisms, a shortage of antioxidants, and increased free radicals result in cell membrane and protein damage.
Other Factors
Crystallins may have a number of functions. For example α-crystallin may be a chaperone that binds to other lens proteins to prevent precipitation. Decreased crystallin levels cause proteins to precipitate, which leads to cataract formation. Phase separation of proteins refers to the hydrophobic aggregation of lens proteins causing protein rich and poor regions in the lens fibers, which results in light scatter. The lipid composition of the cell membranes also changes with age, which may have functional consequences.
Genetics
Many inherited genetic syndromes and metabolic disorders are associated with cataracts, and at least 42 genes and loci have been found to underlie Mendelian inherited forms of isolated or primary cataract. Increasing evidence exists that several genes underlying rare forms of inherited cataract also can influence susceptibility to the much more prevalent forms of age-related cataract. These observations raise the possibility of molecular genetic links between lens development and aging. Age-related cataracts are inherited as a multifactorial or complex trait. Determining the genetics of age-related cataracts is difficult because only a small proportion of the genes involved have been identified, similar gene mutations result in different cataract phenotypes, and cataract epigenetics is complex.
Epigenetics are heritable changes in the gene expression without changes in the DNA sequence. Most genes involved in cataract formation show decreased expression. DNA methylation and other histone modifications inhibit RNA transcription, effectively silencing gene expression. For example, in age-related cataracts, the gene DNA for the alpha A-crystallin protein is hypermethylated. Therapy targeted at reversing methylation and upregulating RNA transcription may prevent or reduce lens opacities. Genes that preserve lens clarity function in diverse processes including protein synthesis (e.g., structural proteins, chaperones, and cell-cycle-control proteins) and reducing oxidative stress (e.g., glutathione peroxidases). Decreased expression reduces lens epithelial cell stress tolerance and protein synthesis. Increased gene expression also can cause lens opacities, for example, by increasing lens epithelial cell ionic transport (e.g., calcium-ATPase, which controls calcium channels) and extracellular matrix protein production. Identifying more genetic and epigenetic determinants of inherited and age-related cataracts may result in nonsurgical treatments for cataract or lifestyle interventions (e.g., diet) and may prevent or reverse cataract formation.
Cell Proliferation and Differentiation
Aqueous growth factors including fibroblast growth factor (FGF), epidermal growth factor (EGF), insulin-like growth factor (IGF), platelet-derived growth factor (PDGF), and transforming growth factor (TGF-β) promote proliferation, differentiation into lens fibers, and maturation of the lens fibers. These processes will not occur if growth factor or cytokine concentrations are incorrect. Then undifferentiated cells migrate to the posterior pole, causing posterior subcapsular cataracts.
Metabolic Disturbance and Osmotic Regulation Failure
Altered gene expression changes enzyme growth factor, membrane protein, and other protein levels, which reduces energy production; changes ion transport, calcium metabolism, and antioxidant pathways; and breaks down protective mechanisms. For example, the lens maintains high intracellular potassium and low sodium levels with the opposite extracellular concentrations via the action of the sodium–potassium ATPase pump. Pump inactivation causes increased intracellular osmolality, which results in water accumulation and light scatter.
The aqueous humor is a source of nutrients and mineral ions including calcium (Ca 2+ ). Ca 2+ is an intracellular signal that regulates many functions including the permeability of the cell membranes. The extracellular Ca 2+ concentration is 10 times the intracellular Ca 2+ concentration, which drives Ca 2+ into the epithelial cell. Low intracellular Ca 2+ levels also are maintained by intracellular organelle membrane pumps (on the endoplasmic reticulum, Golgi apparatus, and mitochondria) and by binding to complex proteins (e.g., crystallins). Extracellular Ca 2+ can be bound to the outer layer of the cell membrane. Reduced binding of Ca 2+ by membrane proteins increases cell membrane permeability and causes a rise in intracellular Ca 2+ levels, the formation of calcium oxylate crystals, binding of Ca 2+ to insoluble lens proteins, increased light scattering, and nuclear cataract formation. Increased intracellular Ca 2+ levels also affect lens epithelial cell differentiation, causing posterior subcapsular cataracts. Corticosteroids have been shown to mobilize intracellular Ca 2+ in other tissues, which can increase Ca 2+ levels. In the future, Ca 2+ -regulating drugs may be developed to prevent cataracts.
Calpains
The roles of calpains in the lens are poorly understood, but they may degrade accumulated damaged lens proteins. A lack of calpains can lead to elevated levels of damaged proteins, reduce optical performance, and cause cataract. Also, excessive stimulation of calpain activity by raised Ca 2+ levels can increase proteolysis and cause cataracts. Calpain inhibitors, therefore, could be useful in the nonsurgical treatment of cataract. However, calpain inhibitors of high molecular weight are unable to cross membranes, so have been of no therapeutic use, whereas others are poorly water soluble or are toxic to lenses.
Protein Modification
Additive modifications of lens proteins (e.g., crystallins) include methylation, acetylation, carbamylation, glycation in diabetics, and binding of ascorbate, which may be the cause of lens discoloration. These additions occur especially in disease and can alter the function or properties of a protein. Diabetes (reducing sugars), renal failure (cyanate generated from urea), aging (photooxidation products), and corticosteroid use (ketoamines) have been linked to cataracts. Additive modifications can make proteins more susceptible to photooxidation by ultraviolet (UV) light.
Subtractive modifications include cleavage by enzymes (such as calpains) of crystallin that causes precipitation of lens proteins. Cleavage of channel proteins can affect intercellular communication. Neutral modifications such as isomerization can denature proteins, affecting their function. Deamidation changes the charge and affects protein–protein interactions.
Proteins in the center of the lens are as old as the individual and are very stable, but over time they can undergo conformational changes (unfolding) that expose thiol groups, which are usually “hidden” in the folds of the protein ( Fig. 5.3.1 ). The exposed glutathione groups can be oxidized to form disulfide bonds (GSSG), causing aggregation of proteins. The conformation changes and aggregation result in scattering and absorption of light.

Oxidation
Oxidation is a key feature in the pathogenesis of most cataracts. Low oxygen levels (O 2 ) are important for maintaining a clear lens. Free radicals and other oxidants, including reactive oxygen species (ROS) and reactive nitrogen species (RNS), are derived from both endogenous sources (mitochondria, peroxisomes, endoplasmic reticulum, phagocytic cells, etc.) and exogenous sources (pollution, alcohol, tobacco smoke, heavy metals, transition metals, industrial solvents, pesticides, and certain drugs like halothane, paracetamol, and radiation). Free radicals can adversely affect nucleic acids, lipids, and proteins, altering the normal redox status and leading to increased oxidative stress and cataracts.
A steep oxygen gradient occurs from the outer part of the lens to the center. Mitochondria in the lens cortex remove most of the oxygen, thus keeping nuclear O 2 levels low. However, in older people, mitochondrial function diminishes and superoxide production by the mitochondria increases, resulting in increased nuclear oxygen and superoxide levels. As the lens ages, a lens barrier develops at approximately the cortex–nuclear interface, which impedes the flow of molecules such as antioxidants (including glutathione) into the nucleus. Unstable nuclear molecules such as peroxide (H 2 O 2 ), which are generated in the nucleus or which penetrate the barrier, therefore, cause protein oxidation. Also, a lower concentration of antioxidants exists. Decomposition of UV filters in the nucleus also produces unstable reactive molecules that bind to proteins, especially if antioxidant glutathione (GSH) levels are low. Ascorbate becomes reactive with proteins in the absence of GSH. These oxidative changes can be detected even in the earliest cataracts and are progressive. Elevated levels of superoxide H 2 O 2 in the aqueous may cause cortical cataracts, since the cortex is closest to the aqueous. Copper and iron are present in higher concentrations in cataract lenses and are involved in redox reactions, which produce hydroxyl radicals.
Defensive Mechanisms
Antioxidant enzymes and antioxidants such as ascorbate, glutathione, tocopherols, and carotenoids maintain lens proteins in the reduced state and are the primary defense mechanisms. In advanced age-related nuclear cataracts, more than 90% of protein sulfhydryl groups and almost half of all methionine residues in the nuclear proteins become oxidized. Secondary defenses include proteolytic and repair processes, which degrade and eliminate damaged proteins, UV filters, and other molecules such as glutathione reductase and free radical scavenging systems. Failure of these protective mechanisms, a shortage of antioxidants, and increased free radicals result in cell membrane and protein damage.
Other Factors
Crystallins may have a number of functions. For example α-crystallin may be a chaperone that binds to other lens proteins to prevent precipitation. Decreased crystallin levels cause proteins to precipitate, which leads to cataract formation. Phase separation of proteins refers to the hydrophobic aggregation of lens proteins causing protein rich and poor regions in the lens fibers, which results in light scatter. The lipid composition of the cell membranes also changes with age, which may have functional consequences.
Cataract Causes, Associations, and Prevention
Age
The cumulative effect of many environmental factors (UV light, x-irradiation, toxins, metals, corticosteroids, drugs, and diseases, including diabetes) causes age-related cataracts. Gene expression changes result in altered enzyme, growth factor, and other protein levels. Protein modification, oxidation, conformational changes, aggregation, formation of the nuclear barrier, increased proteolysis, defective calcium metabolism, and defense mechanisms occur with increasing age. Compromised ion transport leads to osmotic imbalances and intercellular vacuolation. Age-related abnormal cellular proliferation and differentiation also produce opacities ( Fig. 5.3.2 ). There is also an increased incidence of diseases such as diabetes that causes cataracts.

Sunlight and Irradiation
UV-B light causes oxidative damage, which is cataractogenic. The level of free UV filters in the lens decreases with age, and breakdown products of the filters act as photosensitizers that promote the production of reactive oxygen species and oxidation of proteins in the aging lens. The risk of cortical and nuclear cataract is highest in those with high sun exposure at a younger age. Exposure later in life was more weakly associated with these cataracts. Wearing sunglasses, especially when younger, has some protective effect. Unfortunately, the risk attributable to sunlight exposure is small, and cortical cataracts are less debilitating than nuclear or posterior subcapsular cataracts. Therefore, reducing sunlight exposure may have a limited benefit in delaying the onset of cataracts. Exposure to high levels of X-rays and whole-body irradiation also causes cataracts.
Smoking and Alcohol
Smoking causes a threefold increase in the risk of developing nuclear cataracts, and cessation of smoking reduces this risk. Smoking also may be associated with posterior subcapsular cataracts. Smokers are more likely to have a poor diet and high alcohol consumption, which also are risk factors for cataract. Smoking causes a reduction in endogenous antioxidants. Tobacco smoke contains heavy metals such as cadmium, lead, and copper, which accumulate in the lens and cause toxicity. No association between passive smoking and cataract has been demonstrated. Alcohol use has little, if any, association with cataract risk, and study reports are mixed.
Body Mass Index
A number of health-related factors—diabetes, hypertension, and body mass index (BMI)—are associated with various forms of lens opacity, and they may be interrelated. A high BMI increases the risk of developing posterior subcapsular and cortical cataracts. A high BMI also is associated with diabetes and hypertension, which are associated with cataracts. Severe protein-calorie malnutrition is a risk factor for cataracts. Therefore, a moderate calorie intake may be optimal to reduce the risk of developing cataracts.
Myopia
After controlling for age, gender, and other cataract risk factors (diabetes, smoking, and education), posterior subcapsular cataracts are associated with myopia, deeper anterior chambers, and longer vitreous chambers.
Trauma
Blunt trauma that does not result in rupture of the capsule may allow fluid influx and swelling of the lens fibers. The anterior subcapsular region whitens and may develop a characteristic flower-shaped pattern ( Fig. 5.3.3 ) or a punctate opacity. A small capsular penetrating injury results in rapid fiber hydration and a localized lens opacity, and a larger rupture results in complete lens opacification. Penetrating injuries can be caused by accidental or surgical trauma such as a peripheral iridectomy or during a vitrectomy.


Electric shocks as a result of lightning or an industrial accident cause coagulation of proteins, osmotic changes, and fernlike, grayish white anterior and posterior subcapsular opacities. Ionizing radiation, such as from X-rays, damages the capsular epithelial cell DNA, affecting protein and enzyme transcription and cell mitosis. An enlarging posterior pole plaque develops. Nonionizing radiation, such as infrared, is the cause of cataract in glassblowers and furnace workers working without protective lenses. A localized rise in the temperature of the iris pigment epithelium causes a characteristic posterior subcapsular cataract, which may be associated with exfoliation of the anterior capsule.
Systemic Disorders
In uncontrolled type 1 diabetes mellitus in young people, hyperglycemia causes glucose to diffuse into the lens fiber, where aldose reductase converts it to sorbitol. The cell membrane is impermeable to sorbitol, and therefore it accumulates. The osmotic effect draws water into the lens fibers, which swell and then rupture. The cataract progresses rapidly with the development of white anterior and posterior subcapsular and cortical opacities.
In type 2 diabetic adults, an early onset age-related type of cataract occurs and is more prevalent with longer duration of the diabetes. Many mechanisms are involved and include sorbitol accumulation, protein glycosylation, increased superoxide production in the mitochondria, and phase separation. During hyperglycemia, glucose is reduced to sorbitol, depleting antioxidant reserves, and less glutathione is maintained in the reduced form, which causes other oxidative damage. Levels of lens Ca 2+ also are elevated, which activates calpains, causing unregulated proteolysis of crystallins. The cataracts are usually cortical or posterior subcapsular or, less frequently, nuclear and progress more rapidly than age-related cataract.
Galactosemia is an autosomal recessive disorder in which a lack of one of the three enzymes involved in the conversion of galactose into glucose causes a rise in serum galactose levels. Galactitol accumulates in the lens fibers, drawing water into them. Infantile anterior and posterior subcapsular opacities progress to become nuclear. Galactose 1-phosphate uridyltransferase galactosemia is associated with failure to thrive, mental retardation, and hepatosplenomegaly. Dietary restriction of galactose prevents cataract progression. Galactokinase deficiency is associated with galactosemia and cataract but without the systemic manifestations.
Fabry’s disease is an X-linked lysosomal storage disorder that results in accumulation of the glycolipid ceramide trihexoside. The patient suffers from episodic fever, pains, hypertension, renal disease, and a characteristic rash. In the affected man and the carrier woman, a typical mild, spokelike, visually insignificant cataract develops.
Lowe’s or oculocerebrorenal syndrome is a severe X-linked disorder that results in mental retardation, renal tubular acidosis, metabolic acidosis, and renal rickets. Congenital glaucoma, cataracts, and corneal keloids can cause blindness. The lens is small and discoid with a total cataract. Female carriers may show focal dot opacities in the cortex.
Alport’s syndrome is a dominant, recessive, or X-linked trait disease causing hemorrhagic nephropathy and sensorineural deafness. Congenital or postnatal cortical cataract, anterior or posterior lenticonus, and microspherophakia occur.
Dystrophia myotonica is a dominantly inherited disorder and results in muscle wasting and tonic relaxation of skeletal muscles. Other features include premature baldness, gonadal atrophy, cardiac defects, and mental retardation. Cataract is a key diagnostic criterion and may develop early, but usually occurs after 20 years of age and progresses slowly, eventually becoming opaque. Early cataract consists of polychromatic dots and flakes in the superficial cortex. As the opacities mature, a characteristic stellate opacity appears at the posterior pole. Other ocular features include hypotony, blepharitis, abnormal pupil responses, and pigmentary retinopathy.
Rothmund–Thomson syndrome is an autosomal recessive disorder characterized by poikiloderma, hypogonadism, saddle-shaped nose, abnormal hair growth, and cataracts, which develop between the second and fourth decades of life and progress rapidly.
Werner’s syndrome or adult progeria is an autosomal recessive disorder with features that include premature senility, diabetes, hypogonadism, and arrested growth. Juvenile cataracts are common. The condition usually leads to death at about 40 years of age.
Cockayne’s syndrome causes dwarfism but with disproportionately long limbs with large hands and feet, deafness, and visual loss from retinal degeneration, optic atrophy, and cataracts.
Dermatological Disorders
The skin and the lens are of ectodermal origin embryologically. Therefore, skin disorders may be associated with cataract formation.
Atopic dermatitis and eczema may affect any part of the body, especially the limb flexures. Localized proliferation of lens epithelium occurs in some atopic adults, usually as a bilateral, rapidly progressive “shield” cataract (a dense, anterior subcapsular plaque with radiating cortical opacities, and wrinkling of the anterior capsule). Posterior subcapsular opacities also may occur.
Ichthyosis is an autosomal recessive disorder that features hypertrophic nails, atrophic sweat glands, cuneiform cataracts, and nuclear lens opacities.
Incontinentia pigmenti is an X-linked dominant disorder that affects skin, eyes, teeth, hair, nails, and the skeletal, cardiac, and central nervous systems. Blistering skin lesions occur soon after birth, followed by warty outgrowths. Ocular pathology includes cataract, chorioretinal changes, and optic atrophy.
Central Nervous System Disorders
Neurofibromatosis (types I and II) is an autosomal dominant disorder causing numerous intracranial and intraspinal tumors and acoustic neuromata. Ocular features include combined hamartoma of the retina and retinal pigment epithelium, epiretinal membranes, Lisch nodules (a diagnostic sign), and posterior subcapsular or cortical cataracts that develop in the second or third decade of life.
Zellweger syndrome, also known as hepatocerebrorenal syndrome, is an autosomal recessive disorder characterized by renal cysts, hepatosplenomegaly, and neurological abnormalities. Ocular features include corneal clouding, retinal degeneration, and cataracts.
Norrie’s disease is an X-linked recessive disorder that causes leukokoria and congenital infantile blindness and is associated with mental retardation and cochlear deafness. In the eye, vitreoretinal dysplasia, retinal detachment, vitreous hemorrhage, and formation of a white retrolental mass occur. Eventually, a cataract forms.
Ocular Disease and Cataracts
Inflammatory uveitis (e.g., Fuchs’ heterochromic cyclitis and juvenile idiopathic arthritis) usually results in posterior subcapsular or posterior cortical lens opacities. Infective uveitis (e.g., ocular herpes zoster, toxoplasmosis, syphilis, and tuberculosis) can cause cataracts, but the organism does not penetrate the lens. In maternal rubella infection, after 6 weeks of gestation, the virus can penetrate the lens capsule, causing unilateral or bilateral, dense, nuclear opacities at birth, or they may develop several weeks or months later. Corticosteroid treatment can cause cataracts. Retinal pigment degenerations such as retinitis pigmentosa, Usher’s syndrome, and gyrate atrophy are associated with cataracts, which are usually posterior subcapsular opacities. Retinal detachment and retinal surgery may cause a posterior subcapsular cataract particularly in association with vitrectomy, silicone oil injection, and tamponade, or an anterior subcapsular form may develop because of metaplasia of the lens epithelium after vitreoretinal surgery. High myopia is associated with posterior cortical, subcapsular, and nuclear cataracts. Ciliary body tumors may be associated with cortical or lamellar cataract in the affected quadrant. Anterior segment ischemia may cause a subcapsular or nuclear cataract, which progresses rapidly.
Toxic Causes
Topical, inhaled, and systemically administered corticosteroids can cause posterior subcapsular cataracts. Direct mechanisms included interaction of corticosteroids with enzymes that affects their function, e.g., corticosteroid modulation of Na + ,K + -ATPase may cause sodium–potassium pump inhibition affecting osmotic regulation. Corticosteroids also induce crystallin conformational changes, causing aggregation and affect intracellular Ca 2+ homeostasis, causing protein bonding. Indirectly, corticosteroids affect DNA/RNA synthesis of proteins and enzymes, causing metabolic changes, and also may affect ciliary body growth hormone levels responsible for lens cellular differentiation, causing posterior subcapsular opacities.
Chronic use of long-acting anticholinesterases previously used in the treatment of chronic open-angle glaucoma may cause anterior subcapsular vacuoles and posterior subcapsular and nuclear cataracts. Pilocarpine, a shorter acting agent, causes less marked changes. The mechanism of action is unknown. Phenothiazines, such as chlorpromazine, may cause deposition of fine, yellow-brown granules under the anterior capsule in the pupillary zone and may develop into large stellate opacities but are not usually visually significant. The development of the opacities may be related to the cumulative dose of the medication, and photosensitization of the lens may play a role. Allopurinol used in the treatment of gout is associated with cataracts. Psoralen–UV-A therapy for psoriasis and vitiligo has been shown to cause cataracts in very high doses in animal studies but is rare in humans; concomitant UV exposure may be a risk factor. Antimitotic drugs used in the treatment of chronic myeloid leukemia, such as busulfan, may cause posterior subcapsular cataract. The antimalarial chloroquine (but not hydroxychloroquine), which is also used in the treatment of arthritis, may cause white, flake-like posterior subcapsular lens opacities. Amiodarone is used to treat cardiac arrhythmias and causes insignificant anterior subcapsular opacities and corneal deposits. The relationship between statins and cataracts is still controversial.
Siderosis, from a ferrous intraocular foreign body, causes iron deposits in the lens epithelium and iris and results in a brown discoloration of the iris and a flower-shaped cataract. Wilson’s disease, an autosomal recessive disorder of copper metabolism, causes a brown ring of copper deposition in Descemet’s membrane and the lens capsule, resulting in a sunflower cataract—an anterior and posterior capsular disc-shaped polychromatic opacity in the pupillary zone with petal-like spokes that is not usually visually significant. Hypocalcemia in hypoparathyroidism is associated with cataracts. In children, the cataract is lamellar; in adults it produces an anterior or posterior punctate subcapsular opacity.
Congenital and Juvenile Cataracts
Congenital cataracts are noted at birth, infantile cataracts occur in the first year, and juvenile cataracts develop during the first 12 years of life. Hereditary cataracts may be associated with other systemic syndromes, such as dystrophia myotonica. About one-third of all congenital cataracts are hereditary and unassociated with any other metabolic or systemic disorders.
Trisomy 21, or Down syndrome, is the most common autosomal trisomy, with an incidence of 1 per 800 births. Systemic features include mental retardation, stunted growth, mongoloid facies, and congenital heart defects. Ocular features include visually disabling lens opacities in 15% of cases, narrow and slanted palpebral fissures, blepharitis, strabismus, nystagmus, light-colored and spotted irides (Brushfield spots), keratoconus, and myopia. Cataract also is associated with trisomy 13 (Patau’s syndrome), trisomy 18 (Edwards’ syndrome), Cri du chat syndrome (deletion of short arm of chromosome 5), and Turner’s syndrome (X chromosome deletion).
A total cataract is a complete opacity present at birth. It may be hereditary (autosomal dominant or recessive) or associated with systemic disorders such as galactosemia, rubella, and Lowe’s syndrome. Infantile cataracts cause amblyopia if unilateral and may cause strabismus and nystagmus if bilateral. The incidence is about 0.4% of newborns, but the majority of cases are not associated with poor vision. Amblyopia depends on the size, location, and density of the cataract. The causes of infantile cataracts are many and include maternal infections (such as rubella), systemic diseases, hereditary disorders, and ocular disease.
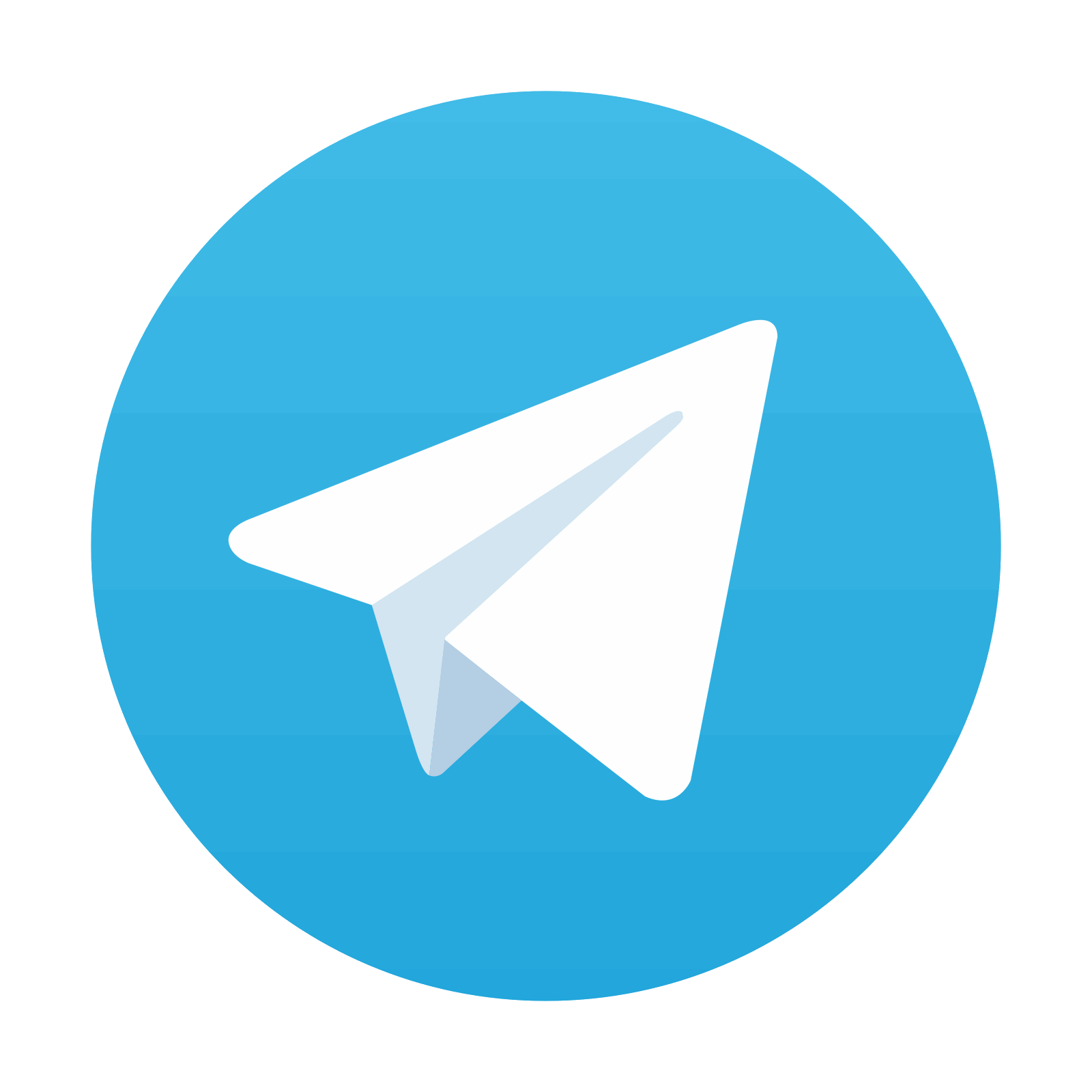
Stay updated, free articles. Join our Telegram channel

Full access? Get Clinical Tree
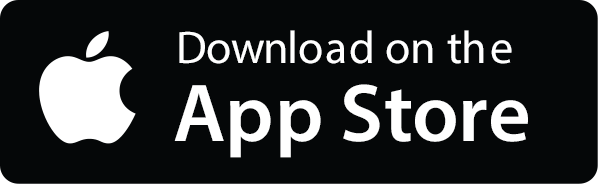
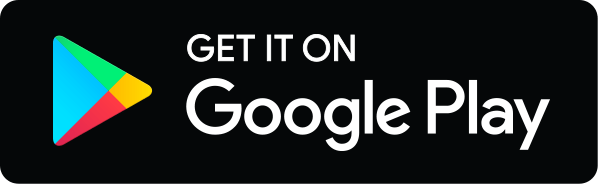