It is well established that endolymphatic hydrops plays a role in Ménière disease, even though the precise role is not fully understood and the presence of hydrops in the ear does not always result in symptoms of the disease. It nevertheless follows that a scientific understanding of how hydrops arises, how it affects the function of the ear, and how it can be manipulated or reversed could contribute to the development of effective treatments for the disease. Measurements in animal models in which endolymphatic hydrops has been induced have given numerous insights into the relationships between hydrops and other pathologic and electrophysiological changes, and how these changes influence the function of the ear. The prominent role of the endolymphatic sac in endolymph volume regulation, and the cascade of histopathological and electrophysiological changes that are associated with chronic endolymphatic hydrops, have now been established. An increasing number of models are now available that allow specific aspects of the interrelationships to be studied. The yclical nature of Ménière symptoms gives hope that treatments can be developed to maintain the ear in permanent state of remission, possibly by controlling endolymphatic hydrops, thereby avoiding the rogressive damage and secondary pathologic changes that may also contribute to the patient’s symptoms.
Endolymphatic hydrops and Meniere’s disease
Endolymphatic hydrops represents a pathologic anatomic finding in which the structures bounding the endolymphatic space are distended by an enlargement of endolymphatic volume. This finding was initially reported in patients with Meniere’s disease by Hallpike and Cairns and Yamakawa. In the cochlea, endolymphatic hydrops is typically seen as a distension of the Reissner membrane into scala vestibuli (SV). Fig. 1 shows mid-modiolar sections through a normal and a hydropic guinea pig cochlea in which the endolymphatic compartment has been highlighted, showing the marked enlargement of the endolymphatic space and distension of the Reissner membrane. Other membranous structures in the ear may also be displaced to varying degrees, including those bounding the saccule, utricle, and ampullae of the semicircular canals. The degree of distension appears to be related to the mechanical compliance of membranous components of the inner ear, with high compliance (ie, mechanically weaker membranes) in the saccule and lower compliance (ie, mechanically stronger membranes) in the semicircular canals. Variations in mechanical compliance of the boundary membranes (analogous to “weak spots” on a balloon) may also explain the considerable variations in degree of hydrops in different regions of the same specimen. Displacements of the basilar membrane in the apical segments of the cochlea have also been reported, in some patients to a degree whereby the basilar membrane contacts the bony wall of the scala tympani (ST). In some specimens, membrane ruptures, herniations, and “scarring resulting from sealed prior ruptures” have been observed. Ruptures of the boundary membranes may contribute to the episodic “attacks” and functional changes that characterize the disease.
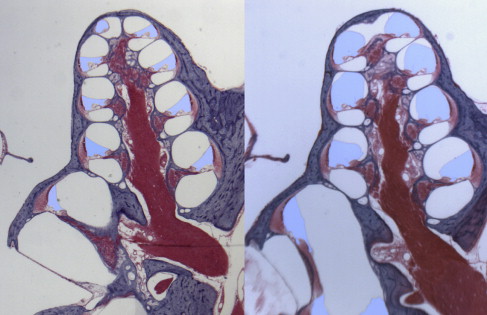
The relationship between endolymphatic hydrops and Meniere’s disease is not a simple, ideal correlation. The temporal bone specimens from patients in the early stages of Meniere’s disease showed both sensory and supporting cells to be remarkably normal in appearance, hence not accounting for the functional deficits exhibited by the patient. Instead, the observation of endolymphatic hydrops in such specimens was considered as a potential cause of the disease. Careful temporal bone studies have confirmed that all patients diagnosed with Meniere’s disease exhibited endolymphatic hydrops in at least one ear. There were, however, a significant number (57 of the temporal bones collected from 963 patients) in which endolymphatic hydrops was observed in specimens from patients with hearing loss, albeit not necessarily fluctuating or in the low frequencies, but not exhibiting the classic symptoms of Meniere’s disease; this has been termed “asymptomatic hydrops.”
Animal models of endolymphatic hydrops
The functional consequences of endolymphatic hydrops depend on the time course over which hydrops is induced. Acute endolymph volume manipulations are dominated by mechanical effects on the endolymphatic boundaries as they are stretched or displaced. In contrast, when hydrops develops over a prolonged period of time there are numerous additional biochemical and morphologic changes that occur. These 2 types of models of hydrops are therefore considered separately.
Acute Models of Hydrops
Injections into the endolymphatic space
The injection of an artificial endolymph into the endolymphatic system, usually into the cochlea, provides an apparently simple model of hydrops. The physiologic effects of endolymphatic injections depend on the rate and volume injected. For low injection rates, endocochlear potential (EP) is increased, due to the reduction in current through the organ of Corti as it is displaced toward ST. With higher injection rates, EP decreases are observed, likely associated with chemical or mechanical trauma to the system. Sirjani and colleagues showed that with a low injection rate (80 nL/min) there were f 2 -f 1 emission changes associated with operating point changes of the transducer and a small threshold elevation of cochlear action potential (CAP) thresholds at 2.8 kHz, whereas EP, summating potential, and CAP thresholds at 8 kHz were unchanged. With higher injection rates (200–400 nL/min) distortion and operating point changes were larger, accompanied by increases of summating potential, EP, elevations of thresholds at 2.8 and 8 kHz, and a suppression of 2f 1 -f 2 emissions. Most of these induced changes occurred transiently during the injection and recovered rapidly afterwards, a result that is not consistent with the volume injection causing a sustained hydrops. Measurements of endolymph pressure relative to that of perilymph during and following endolymphatic injections show that pressure is elevated during injections but rapidly dissipates following the injection. Modeling the time course of pressure change during and following injection showed the pressure time course to be consistent with endolymph passing through the ductus reunions into a compartment more compliant than the cochlea (ie, the saccule). Thus, the primary physiologic changes in the injection model appear to result from induced pressure changes during the injection, rather than from a sustained volume increase of cochlear endolymph. This finding contrasts with those in prolonged hydrops, as detailed later.
Cerebrospinal fluid pressure manipulations
It has been reported that in patients and animals, a decrease in hearing sensitivity may occur after leak or drainage of cerebrospinal fluid (CSF). It has been proposed that a compensatory expansion of the endolymphatic space may be the cause of this hearing loss, but this has not been proven definitively. In addition, there are case reports of large decreases in hearing sensitivity induced in Meniere’s patients by manipulation of intracranial pressure, but it is not known how losses are made worse by the hydropic state. Low-frequency hearing loss (without a proven hydrops) has been found in association with benign intracranial hypertension, a syndrome characterized by increased intracranial pressure without focal signs of neurologic dysfunction.
Low-frequency sound
It was reported that f 2 -f 1 distortion and cochlear transducer operating point changes, consistent with a displacement of the organ of Corti toward ST, were produced by low-frequency sound (200 Hz) delivered at high, but nondamaging levels (115 dB SPL). Measurements of endolymph volume using a marker ion during 200 Hz, 115 dB SPL sound exposures confirmed that a substantial increase of endolymph volume (>30%) was induced by the exposure, with recovery within minutes afterwards. A notable observation in this study was that in the few minutes immediately after the sound exposure, when hydrops was maximal and in the range of 20% to 30% increase relative to the normal state, cochlear sensitivity to sounds showed almost no impairment. CAP thresholds at 8, 4 and 2 kHz were all within 4 dB of the preexposure sensitivity. A contributing factor in accounting for the limited sensitivity change was an increase of EP immediately after the exposure, which would act to increase cochlear sensitivity.
Gel injection into the cochlear apex
Endolymphatic hydrops is expected to cause sustained displacements of the organ of Corti. The functional effects of a sustained displacement have been difficult to study, however, as they are technically difficult to induce. It was recently shown that slow injections of hyaluronate gel into the cochlear apex of guinea pigs produce a sustained transducer operating point change, consistent with a displacement of the organ of Corti toward ST. This situation results from the resistance to the flow of gel passing through ST toward the cochlear aqueduct during injection, causing a small overpressure in SV and endolymph with respect to pressure in the basal turn of ST. In conjunction with the induced organ of Corti displacement, there was a substantial increase in EP, increases in even-order distortions (2f 1 , f 2 -f 1 ), decreases in odd-order distortions (2f 1 -f 2 ), and decreases in cochlear sensitivity as measured by CAP thresholds. These changes were sustained throughout injections of up to 40 minutes in duration and recovered rapidly when injection stopped. In addition, following the injection of 1–2 μL of gel, the helicotrema was apparently obstructed, which produced an approximately 20 dB increase in sensitivity to stimuli of infrasonic frequencies. In the same study, a hypersensitivity to infrasound was reported in animals with surgically induced endolymphatic hydrops, presumed to result from the hydrops similarly obstructing the perilymphatic pathway between SV and ST in the apical turns. Increased sensitivity to infrasonic stimuli may account for anecdotal reports of Meniere’s patients being sensitive to low frequency pressure changes, such as weather fronts and windmills.
Cyclic adenosine monophosphate manipulations
One of the earliest models for endolymphatic hydrops was the injection of cholera toxin into the endolymphatic space. Subsequent studies showed that hydrops was also induced by perilymphatic administration of the toxin, which was believed to act by stimulating adenylate cyclase and elevating cyclic adenosine monophosphate (cAMP). Stimulation of adenylate cyclase by forskolin was found to produce increases of endolymph Cl and of the EP. An increased entry of Cl into endolymph of this model is likely to be the cause of the hydrops. In the frog, it has also been shown that ion transport was modulated by stimulation of cAMP levels with the antidiuretic hormone, vasotocin.
Chronic Models of Hydrops
Surgical ablation of the endolymphatic sac
The most widely studied model of endolymphatic hydrops was created by surgical ablation of the endolymphatic duct and sac in guinea pigs. Within a few days of sac ablation a mild hydrops developed, primarily affecting the membranes bounding the saccule, endolymphatic sinus, Reissner membrane in the cochlea and, to a lesser degree, the utricle. Initially the semicircular canals were unaffected. Over the course of a few months, the hydrops became increasingly severe, with the Reissner membrane severely distended into SV and the space almost filled by endolymph after 3 to 4 months. The model was remarkable because, similar to the pathology reported at the time in Meniere’s disease, it exhibited a notable endolymphatic hydrops without a major loss of hair cells or apparent damage to stria vascularis. Functional studies with this model subsequently showed that the cochlear microphonics became increasingly suppressed with time, especially for low-frequency stimuli. Both the EP and the saccular potential decreased progressively with time. This result contrasts with the cAMP-dependent models of hydrops discussed earlier, in which EP was elevated, demonstrating that the electrochemical causes/effects of endolymphatic hydrops are not consistent and vary with how the hydrops is induced. Sensitivity changes of the ear in this model, measured by CAP thresholds, were small, variable, and confined to low stimulus frequencies within the first month after surgery, progressing to become profound and affecting all frequencies after a period of 3 to 4 months. In contrast to the enlarged summating potential (SP) reported in Meniere patients, the increase of SP in hydropic guinea pigs was small and confined to the initial period after sac ablation, with no SP enlargement present in the later stages when the cochlea was severely hydropic.
There are numerous histopathological changes that develop with time. In the organ of Corti of the hydropic animal, the first abnormalities are a loss of the shorter stereocilia or a disarray and loss of stereocilia of the outer hair cells first occurring in the apical regions. The changes at the endolymphatic surface became more severe with time and outer hair cells are subsequently lost, followed by inner hair cells, with losses greatest in the apical turns. In the lateral wall, there are early changes in the fibrocytes of the spiral ligament. Later intercellular edema is observed between marginal cells, an increase of vesicles, and vacuolization and atrophy of marginal and intermediate cells. Changes in cellular morphology occur in the distended Reissner membrane of the hydropic cochlea. Mesothelial cells may be absent and the epithelial cells on the endolymphatic surface are enlarged, suggesting the membrane has “stretched” rather than “grown.” Similar changes of Reissner membrane have been observed in the hydropic human cochlea. Finally, in the later stages of hydrops in both animals and humans, a loss of spiral ganglion cells occurs, primarily affecting the apical turns.
Although initial studies suggested that endolymph Na + was elevated in the hydropic cochlea, several subsequent studies failed to confirm this finding. In the early stages as hydrops develops there are no significant composition changes of the major ions (K + , Na + , Cl − ) in either endolymph and perilymph of the hydropic ear. As hydrops became more pronounced with time, a small decline in endolymph K + , Cl − , and osmolarity occurred in the basal turn. In the normal ear, basal turn endolymph is slightly hypertonic with respect to perilymph and with respect to endolymph of the apical turns. This difference occurs even though the water permeability between endolymph and perilymph is high (equilibration half-time 7.6 minutes). The hyperosmolarity can be accounted for by the biophysical concept of the “reflection coefficient” in which the osmotic activity of a solute depends on its permeability through the membrane dividing compartments. If the solute permeates the membrane, the osmotic pressure generated will be lower than that theoretically expected. In the cochlea, based on studies suggesting that circulating currents are carried predominantly by K + , it can be inferred that the endolymphatic boundary is more permeable to K + than to Na + . If K + is more permeable, it will have a lower reflection coefficient, meaning that a higher concentration will be necessary in endolymph to maintain osmotic equilibrium with perilymph, for which the predominant cation is Na + . The reduction in K + and osmolarity in the basal turn of the hydropic cochlea is therefore more likely related to permeability changes of the endolymphatic boundaries than to fundamental changes in ion transport processes.
In contrast to the major ions, which show only minor changes in hydrops, endolymph Ca 2+ does show a notable increase in the hydropic ear. In the normal ear, the Ca 2+ content of cochlear endolymph is approximately 20 μM, which is extremely low relative to other extracellular fluids. In hydropic ears an increase of endolymph Ca 2+ was observed. The magnitude of the increase was subsequently found to be correlated with the decline of EP. A study of EP and Ca 2+ changes with time confirmed that the Ca 2+ increase correlated with the decline of EP as hydrops progressed. These studies confirmed that the abnormal endolymph Ca 2+ was probably a secondary consequence of endolymphatic hydrops rather than the primary cause. Nevertheless, because endolymph Ca 2+ has been shown to influence transduction in hair cells, it is likely that endolymph Ca 2+ changes contribute to functional losses present in the hydropic cochlea of animals, and possibly in the ears of humans with Meniere’s disease too.
Aldosterone/partial sac ablation
One perceived limitation of the sac ablation model is that the endolymphatic sac is totally nonfunctional, which contrasts with the expected state for human ears with Meniere’s disease. As a result there have been some attempts to induce hydrops in animals without completely ablating the endolymphatic sac. One such model used a partial dissection of the endolymphatic sac, detaching the distal portion from the sigmoid sinus while leaving the proximal, intraosseous portion undamaged. This procedure was performed in conjunction with systemic dosing of aldosterone. The rationale for the use of aldosterone was based on studies showing that animals maintained on a low-Na, high-K diet developed elevated systemic aldosterone levels, which cause an elevation of Na/K ATPase levels in stria vascularis. It was speculated that an enhanced secretion of K into endolymph would increase the rate of “endolymph production,” which combined with partially impaired sac function could create a volume excess. The study found that aldosterone alone caused slight hydrops in some animals, while the sac dissection and sac dissection combined with aldosterone both caused moderate and severe hydrops. No details of the variations between groups were provided, so it was not apparent whether the aldosterone significantly contributed to the degree of hydrops observed. At the very least, this study demonstrates that even partial sac ablation can cause hydrops and that aldosterone may exacerbate the dysfunction.
Systemic vasopressin
It has been reported that in animals given vasopressin (also known as antidiuretic hormone, ADH) subcutaneously for 1 week by a mini-osmotic pump showed a dose-dependent increase in endolymphatic volume, with an estimated endolymph increase ratio of 17% at a dosage of 1000 U/kg/min. Vasopressin (V2) receptors have been demonstrated in inner ear tissues, including the lateral wall and the endolymphatic sac, and may regulate water movements through cochlear tissues by control of aquaporin expression (reviewed in Ref. ). By contrast, intracochlear administration of the V2 receptor antagonist OPC-31260 was shown to reverse the endolymphatic hydrops caused by endolymphatic sac ablation. Ultrastructural studies show an enlargement of the intrastrial space by acutely administered vasopressin. On the other hand, vasopressin plays a pivotal role in water regulation by the kidneys, being lowered at times of water excess, resulting in diuresis, and being elevated at times of dehydration to minimize urine volume. Based on the vasopressin model of hydrops, a period spent in a dehydrated state would elevate vasopressin and possibly result in the generation of endolymphatic hydrops. One study has suggested that the endolymphatic sacs of patients with Meniere’s disease have significantly higher vasopressin receptor levels, suggesting that the ears of these patients may be more sensitive to vasopressin. Other studies have reported elevated systemic vasopressin levels in Meniere’s patients. One recent study showed that increasing the water intake of Meniere’s patients, presumed to reduce the systemic vasopressin level, reduced the severity of their symptoms. While the possibility of vasopressin playing a role in endolymph volume regulation and Meniere’s disease is likely, it still remains uncertain how the vasopressin/aquaporin system interacts with other hormonal systems influencing ion transport and how such a system could be controlled to achieve a stable endolymph volume in the face of varying hydration status.
Lipopolysaccharide and aldosterone
It has been reported that mice treated with intratympanic lipopolysaccharide (LPS) combined with systemic aldosterone over a 5-day period develop mild to moderate endolymphatic hydrops. LPS (a toxin extracted from Escherichia coli ) causes an immune response (a noninfectious labyrinthitis) in the ear. The advantage of this model is that it allows the endolymphatic system of the ear and of the undisturbed endolymphatic sac to be evaluated independently. Although the degree of hydrops in the cochlea was similar for LPS and aldosterone + LPS groups, the endolymphatic sac was more dilated in the aldosterone + LPS group compared with the LPS alone, in which the sac lumen was partially collapsed. A subsequent study with this model has allowed the influence of epinephrine on endolymph in the cochlea and sac to be evaluated. Epinephrine was found to increase the dilation of the intraosseous portion of the sac in the hydrops model. As epinephrine has been shown to modulate K + secretion by marginal cells, the use of this animal model allows the potential influence of different transport processes to be evaluated at the system level.
Pathophysiology of Hydrops
Pressure considerations
Both acute endolymphatic hydrops and the early phase (up to 5 weeks) of chronic hydrops generally occur with negligible (<0.5 mm Hg) change in pressure between endolymph and perilymph, because the endolymphatic boundaries are very compliant, so that endolymph volume increase can occur with very small pressure changes, less than those occurring with respiration and cardiac pulsations. For this reason it is incorrect to characterize the condition as “endolymphatic hypertension” or “glaucoma of the ear.” It is generally accepted that glaucoma in the eye usually results from impaired blood flow, for which increased ocular pressure is one possible cause. Furthermore, perilymphatic pressure is not elevated in the hydropic cochlea and is not elevated in patients with Meniere’s disease, so there is no perilymph pressure abnormality to influence blood flow. Even in the later stages of hydrops (>5 weeks after sac ablation), one study found no endolymph pressure increase in 33% of the animals and a mean increase of less than 1 mm Hg, and another found no significant elevation at all, with a mean increase of 0.003 mm Hg. These findings in the ear contrast with measurements of ocular pressure, which vary from 10 to 21 mm Hg in the normal eye and are typically greater than 22 mm Hg in patients with open-angle glaucoma. In terms of the dependence of pathology on pressure, it is apparent that endolymphatic hydrops and glaucoma of the eye are completely different and that the role pressure plays in endolymphatic hydrops is, at best, limited.
A recent study has shown that the endolymphatic sac may play some part in endolymph pressure regulation. Systemic isoproterenol (β-adrenergic agonist) increased endolymph pressure and decreased the potential of the endolymphatic sac lumen, while not affecting CSF pressure. This action was suppressed in animals in which the endolymphatic sac was surgically ablated, implicating the sac as the source of the changes.
Regulation of cochlear fluids volume and composition
The relationship between ion transport and endolymph composition and volume is complex, as numerous forms of ion transport and many ion-transporting tissues bound the endolymphatic space. A disturbance of any ion transport system at any location in the ear could potentially contribute to volume disturbance. The original concept that hydrops arose due to an imbalance between endolymph secretion in the cochlea and endolymph resorption by the endolymphatic sac (impaired when the sac is ablated) has been shown to be false. Unlike many other body fluids, endolymph is not secreted in volume and does flow along the endolymphatic space at a rate that has physiologic significance. The study that gave rise to the erroneous concept of endolymphatic flow involved the injection of large volume of marker substance into the endolymphatic space, a procedure that has subsequently been shown to induce large nonphysiologic endolymph flows. The observation that normal endolymphatic composition is maintained for days following endolymphatic sac ablation, when the hydrops is mild, further confirms that endolymph homeostasis does not require volume secretion. Instead, homeostasis appears to be dominated by the local transport of ions, with water equilibrating according to osmotic gradients, which is comparable to the situation for cytoplasm of a single cell.
Many of the ion transport processes in tissues bounding endolymph have been shown to be under the regulation of hormonal mechanisms, including β-adrenergic, muscarinic, and purinergic receptors. The linkage between K + transport and endolymph K concentration and volume remains uncertain, however. Although it is commonly assumed that a change in transport of one ion (eg, K + ) will alter endolymph concentration or volume, a volume change can only occur if there is a change in transport of both K + and of a suitable counter ion (eg, Cl − or HCO 3 − ), allowing accumulation of electrolyte in endolymph and resulting in an osmotic influx of water. Aquaporins, also possibly under hormonal regulation, play a role in water equilibration across the endolymphatic boundaries (reviewed in Ref. ). So while potential elements that may be involved in endolymph volume homeostasis have been identified, it is not yet clear how these systems are integrated, and what are the specific roles of the different structures of the ear. One difficulty is the issue of how abnormal endolymph volume states are detected, given the high compliance of the endolymphatic boundaries. Pressure measurements during the initial volume injection into endolymph of the cochlea show identical pressure changes in both endolymph and perilymph, with no difference between them. To correct abnormal volume states and to maintain the normal volume, there must be a way to detect small changes in endolymph volume status where pressure changes are minuscule. While it is accepted that the endolymphatic sac plays a primary role in endolymph volume regulation, its location (adjacent to the pulsations of the sigmoid sinus) makes it unlikely to be a sensitive mechanical detector. Furthermore, while the endolymphatic duct connects endolymph in the saccule to the sac lumen, the endolymphatic sac is not bounded by perilymph and the tissue-filled endolymphatic duct restricts the access of perilymph to the periphery of the sac. Therefore if the detection of hydrops requires the detection of a small pressure of endolymph with respect to perilymph, then the endolymphatic sac is poorly situated for this purpose. Instead, it has been proposed that the endolymphatic sinus, a small structure between the saccule and the utriculo-endolymphatic valve at the entrance to the endolymphatic duct, performs this function. The walls of the sinus, similar to the membranes of the saccule, are highly distensible and their position will be sensitive to even small endolymph volume changes. It has been shown that when pressure was applied to the perilymph, the endolymphatic duct became occluded, presumably as endolymph in the sinus was displaced into the endolymphatic sac, allowing the sinus membrane to occlude the endolymphatic duct opening. It is apparent that the amount of endolymph displaced from the sinus into the endolymphatic sac will depend on the degree of distension of the sinus, with more endolymph entering the sac when the sinus is enlarged. Thus the sinus could control the amount of endolymph being driven into the sac according to the volume status. The pressure fluctuations driving endolymphatic movements may include body movements (fluid inertia) or may result from tensor tympani muscle contractions, which cause the stapes to be displaced toward the labyrinth during swallowing.
Approaches to the imaging and measurement of hydrops
Endolymphatic hydrops was originally documented, and in some later studies quantified, using histologic methods that required fixation, decalcification, embedding, and sectioning of the cochlea. Several groups have quantified endolymph volume increase from scala area measurements from histologic sections either by comparison with the opposite, nonoperated ear or based on the area of scala media relative to the idealized Reissner membrane position defined by a straight line between the attachment points at the spiral limbus and the lateral wall. Endolymph volume in normal and hydropic cochleae was also quantified by 3-dimensional reconstructions of magnetic resonance (MR) images of fixed, but not decalcified, intact specimens. One concern with all these methods is whether the degree of hydrops was influenced by the fixation and processing methods. It was shown that dissected, fresh Reissner membrane segments in vitro underwent substantial shrinkage during fixation with some protocols, suggesting that the degree of hydrops could be underestimated based on measurements from fixed tissues. Recently, there have been major advances in MR imaging that have allowed endolymphatic hydrops to be visualized and quantified in the live ears of animals and humans. These studies offer great potential to correlate the patient’s symptoms or results of other diagnostic tests with the degree of hydrops as a function of time or during different therapies (eg, Ref. ). This subject is covered by Pyykkö and colleagues in more detail elsewhere in this issue for further exploration of this topic.
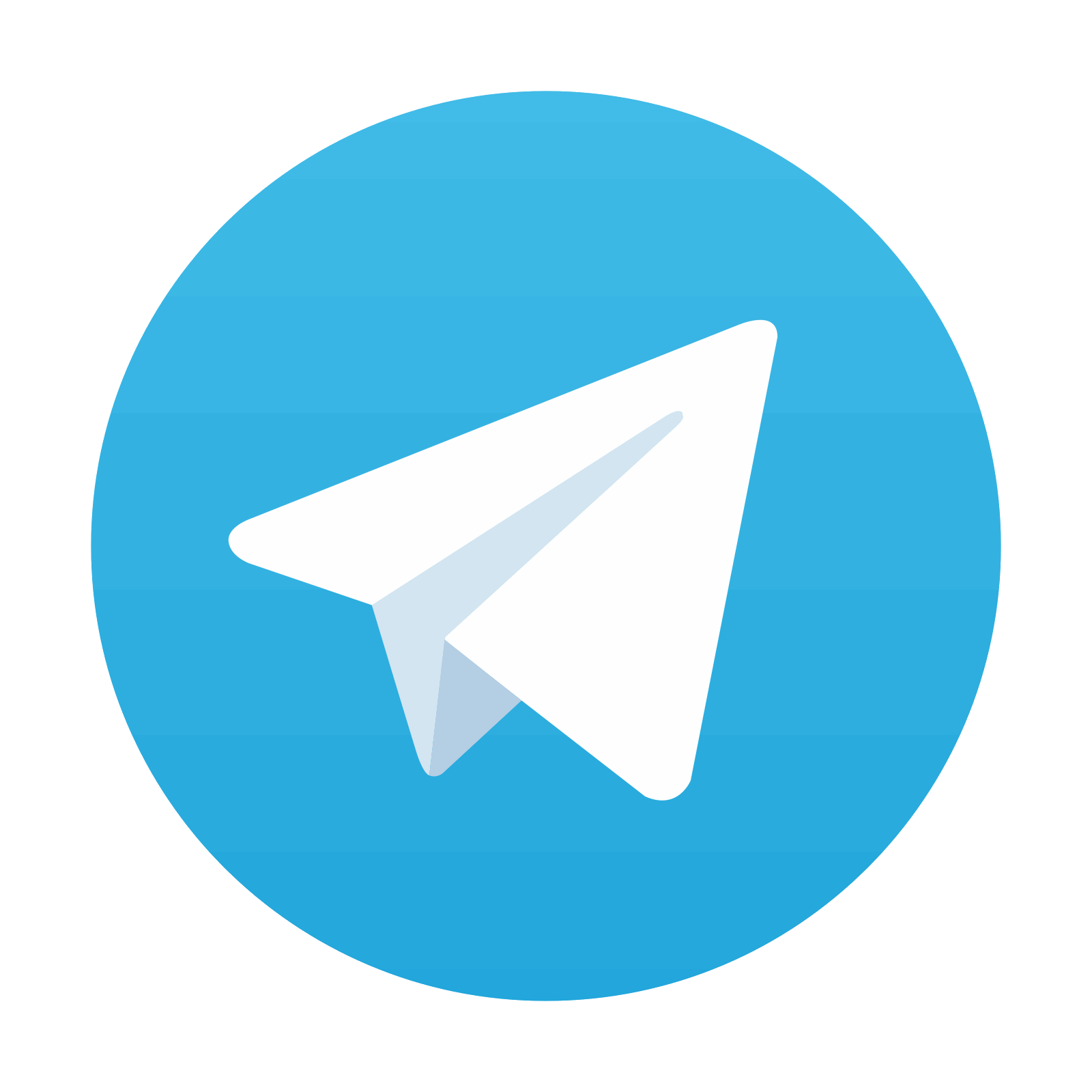
Stay updated, free articles. Join our Telegram channel

Full access? Get Clinical Tree
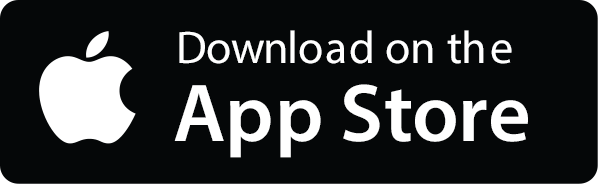
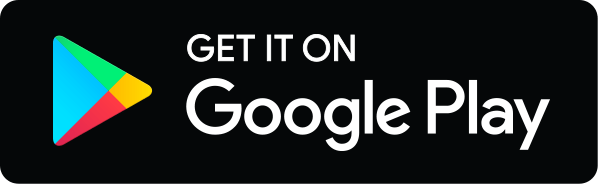