Objectives
After working through this chapter, you should be able to:
Explain what dispersion is
Understand how dispersion occurs
Explain how rainbows are produced
Explain what chromatic aberration is
Discuss the relevance of chromatic aberration in the human eye
Introduction
In this chapter we will start to think about the constituent wavelengths that make up ‘light’ and how white light (e.g. from the sun) can be separated into these wavelengths through a process called dispersion .
Dispersion
As discussed in chapter 1 , white light can be produced when the light source emits all the wavelengths of visible light (e.g. a continuous spectrum), or when the light source emits a large enough range of wavelengths (e.g. a discrete spectrum) that it appears white- ish due to the additive nature of light. For example, sunlight looks white and comprises a continuous spectrum, but if I had a red bulb, a green bulb and a blue bulb, the resultant light would look white but would be classed as a discrete spectrum due to the limited range of wavelengths. However, in some circumstances it is possible for white light to be separated into the individual wavelengths well enough that we can actually see the different colours ascribed to each wavelength (long-red; short-blue). This happens through a process called dispersion . Some of you reading this may have heard about dispersion happening as part of the refractive process when light passes through a prism, but I expect all of you will be familiar with a form of dispersion that happens when it rains on a sunny day to produce a rainbow in the sky! To that end, let’s explain dispersion using the example of a rainbow to start with, and then we can move on to discuss prisms and lenses.
Rainbows
When we see a rainbow in the sky ( Fig. 8.1 ), we will perceive what appears to be a continuous spectrum from long-wavelength light (red) all the way to short-wavelength light (blue/violet). Now, it is no coincidence that the distribution of colours in a rainbow falls in exactly the same pattern as we would expect if we ordered them by wavelength, as the reason that dispersion occurs is because the amount that the light undergoes refraction is partly dependent on the material and partly dependent on the wavelength of the light itself. Sticking with the rainbow example, we probably know that rainbows only occur when there is water in the air (e.g. when it rains), which indicates that it is the refractive properties of the water droplets (the rain) that produces the effect. Most of the time, white light from the sun (all visible wavelengths) can refract through the water droplets in the sky without any trouble, but as we can see in Table 8.1 , the refractive index of materials changes slightly depending on the wavelength of the incident light. For example, shorter wavelengths of light will experience higher refractive indices than longer wavelengths of light. Importantly, this indicates that there can be a difference in the speed each wavelength travels through the material. More specifically, shorter wavelengths are slowed down to a greater extent than the longer wavelengths when they enter these materials, which means that they refract to a greater extent (think back to Snell’s law from chapter 2 ) and therefore will have a greater angle of deviation relative to longer wavelength light. Sometimes we teach this as ‘blue bends best’ to help students learn that short wavelengths (blue-end of the spectrum) will refract at a greater angle than long wavelengths (red-end of the spectrum), however please remember that this only applies to dispersion and refraction – the opposite is true for diffraction which we will learn about in chapter 10 .

Wavelength | Water (n = ∼1.333) | Crown Glass (n = ∼1.523) |
---|---|---|
Red | 1.331 | 1.512 |
Orange | 1.332 | 1.514 |
Yellow | 1.333 | 1.518 |
Green | 1.335 | 1.519 |
Blue | 1.338 | 1.524 |
Violet | 1.342 | 1.530 |
However, as we’ve already discussed, most of the time the white light remains white after refracting through the water droplets, so how does the dispersion produce a rainbow? The answer is that rainbows occur when the sunlight refracts into the droplet, and then reflects back off the farthest surface of the water droplet to make it refract back out of the side it originated from. Fig. 8.2 illustrates what this looks like within a raindrop and how the raindrop can disperse the white light into all the colours of the rainbow. In short, when white light is incident upon a raindrop, each constituent wavelength is refracted by slightly different amounts. The light begins to disperse, but when it reaches the other side of the raindrop, some of the light is reflected back again, and so it is then refracted a second time as it leaves the raindrop. It is the combination of the two refraction opportunities that disperse the light to produce the rainbow – the reflection that occurs on the back surface does not contribute to the dispersion process as reflection does not alter for different wavelengths, but as the wavelengths will already be slightly dispersed, they will each be reflected slightly differently as they will reflect off different locations of the water droplet. However, now you may be wondering – why doesn’t the whole sky look multicoloured in that case? Why is it restricted to a neat little rainbow shape?, Well, we then perceive this process in the sky as a rainbow as we are only able to perceive the light when it reaches our eyes at a particular angle (which makes the rainbow the characteristic arc shape).

Now, if I asked you what shape a rainbow was, what would you say? I suspect you’d say ‘arch’, ‘bridge’, ‘semi-circle’ or something similar to that, and you’d be half right. What if I told you that actually all rainbows are complete circles, but we can only see the top half? In fact, we (as observers close to the ground) are only able to see light reflected above the horizon, and the position of the rainbow will be dictated by our own position on the ground. This means that if I see a rainbow in the sky outside my office and I text my colleague across campus to demand that they look outside, they will see an entirely different rainbow. Ultimately, the key factor affecting the appearance of the rainbow is the antisolar point , defined as the point 180 degrees away from the sun relative to the observer (see Fig. 8.3 for a visual explanation of this). Technically, the central point of the rainbow ( raincircle? ) coincides with the antisolar point, and so logically, in order to see the rainbow you’d have to face away from the sun (which makes sense now that we know rainbows are formed from reflections within raindrops). This also indicates that the height of the sun will directly influence the apparent height of the rainbow, meaning rainbows produced when the sun is low in the sky will appear to be much larger in appearance than those produced when the sun is up high. Interestingly, rainbows form at a specified 42-degree angle between the observer and the antisolar point ( Fig. 8.4 ), which roughly determines the radius of the rainbow itself. This means if the antisolar point falls below the horizon, the rainbow will be set low down relative to the horizon, whereas if the antisolar point is more level with the horizon, then the rainbow will form 42 degrees above that.


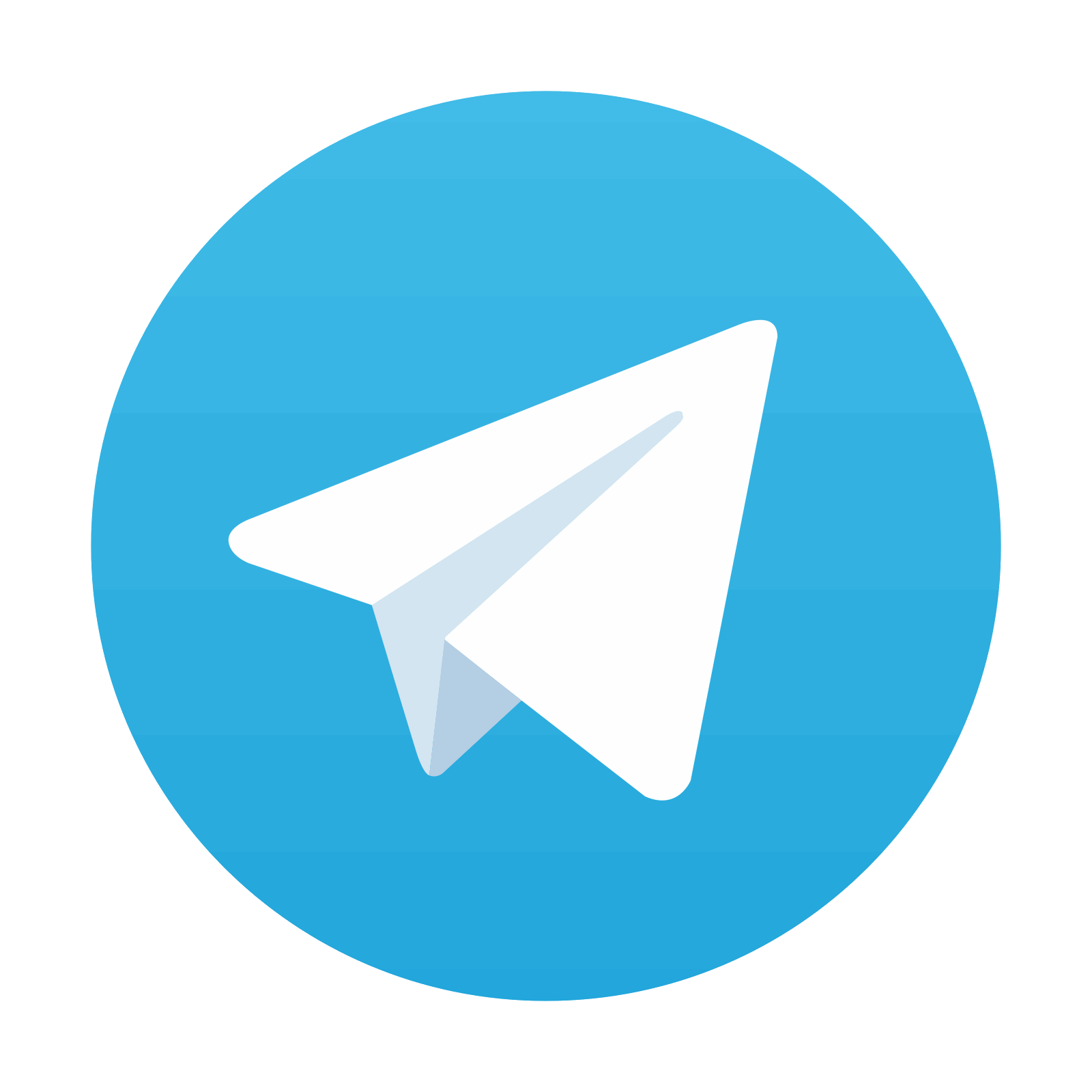
Stay updated, free articles. Join our Telegram channel

Full access? Get Clinical Tree
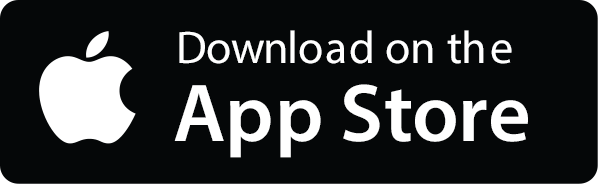
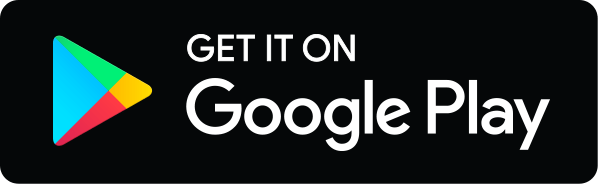
