Introduction
The effects of external trauma and of ophthalmic and systemic disease on human corneal endothelium are best understood by first reviewing the anatomy and physiology of the adult human endothelium. A comprehensive review of this material can be found in Chapter 4.1 .
Fuchs’ Dystrophy
Introduction
Fuchs’ endothelial corneal dystrophy (FD) is a bilateral, noninflammatory, progressive loss of endothelial cells that results in corneal edema and reduction of vision. Its key features include the presence of central guttae, folds in Descemet’s membrane, stromal edema, and microcystic epithelial edema. Corneal endothelial degeneration is the primary defect that leads to the development of corneal edema. Associated features include prominent corneal nerves, stromal opacification, recurrent corneal erosions, female gender predominance, and familial predisposition.
Epidemiology and Pathogenesis
FD is the most common corneal dystrophy to require keratoplasty, accounting for approximately 3.1% of all penetrating keratoplasties (PKPs) and 47.1% of all endothelial keratoplasties in the United States in 2015. The genetic basis of FD is complex and heterogeneous, demonstrating variable expressivity and incomplete penetrance. FD is likely to be complex in etiology, with genetic as well as environmental factors playing a role in its pathogenesis. A significant variation occurs in expressivity between males and females, with a 3–4 : 1 female/male ratio recorded at the time of keratoplasty. It is equally common among white and black patients who undergo keratoplasty but is relatively rare in Asian patients.
Development of guttae and the onset of symptoms are more common in middle age. Patients with FD are believed to have an increased incidence of open-angle glaucoma. Short axial length, shallow anterior chamber, and angle-closure glaucoma also have been seen in conjunction with FD. FD has been associated with keratoconus. The progressive loss of endothelial cell function is primary in nature rather than secondary to any alteration in aqueous humor flow rate or constituency. Endothelial dysfunction is mainly a result of a reduction in sodium, potassium–adenosine triphosphatase (Na + ,K + –ATPase) pump activity, which leads to a reduction in ion flux across the endothelium. Molecular biological studies of corneal buttons from patients with FD suggest that apoptosis may play an important role in endothelial cell degeneration. Recent studies have demonstrated an oxidant–antioxidant imbalance in FD cells, which, in turn, leads to oxidative DNA damage and apoptosis.
Several genetic loci have been identified in patients with FD. The first genetic locus was mapped to chromosome 13, called Fuchs’ corneal dystrophy locus 1 (FCD1). Subsequently, three other loci, FCD2, FCD3, and FCD4, were mapped to chromosomes 18, 5, and 9, respectively. Additionally, other reports have provided evidence for potential linkage to chromosomes 1, 7, 15, 17, and X by genome-wide linkage analysis.
Multiple causal genetic mutations have been linked to FD. Mutations in the ion cotransporter encoded by the SLC4A11 gene, also associated with congenital hereditary endothelial dystrophy, have been linked to the development of FD. Additionally, mutations in the transcription factor TCF8 gene, also associated with posterior polymorphous corneal dystrophy, have been identified in patients with FD. A third causal gene for FD, LOXHD1 , was identified on chromosome 18. This gene has been associated with the development of progressive hearing loss in humans.
A strong association has been discovered between FD and trinucleotide repeats (TNRs) in the transcription factor 4 ( TCF4 ) gene in chromosome 18. This gene encodes the E2-2 transcription factor, which is involved in cellular growth and differentiation. Expansion of TNR lead to messenger RNA (mRNA) missplicing, which leads to RNA toxicity. TNR expansion in TCF4 of more than 50 is highly specific for FD. Changes to the endothelial barrier function, a known event in the development of FD, was identified as a key biological process influenced by the missplicing events.
Mutations in the gene COL8A2 that codes for the α 2 -chain of type VIII collagen have been reported in patients with an early onset endothelial dystrophy that was previously considered an early form of FD. Recent studies suggest that the endothelial dystrophy linked to mutations in COL8A2 represents a disease that is phenotypically distinct from FD.
Ocular Manifestations
The earliest slit-lamp finding in FD is the presence of focal excrescences of extracellular matrix in Descemet’s membrane, called guttae (cornea guttata). In the earliest stages of this disease, the guttae first emerge in the central corneal endothelium ( Fig. 4.21.1 ). Guttae are not specific to FD and may be seen in asymptomatic patients and in the setting of both uveitis and nonspecific superficial keratopathies. Up to 11% of eyes in patients older than age 50 years have guttae. Pathologically identical lesions in peripheral Descemet’s membrane are known as Hassall–Henle warts and are part of the normal aging process (see Chapter 4.22 ).

The guttae initially appear on specular reflection as scattered, discrete, isolated dark structures, smaller than an individual endothelial cell. An associated fine pigment dusting may be observed within the central endothelium. At this stage, referred to as stage 1 , the patient’s vision usually is normal, and the stroma and epithelium are uninvolved. Over time, these individual excrescences increase in number, enlarge, and fuse with adjacent guttae to disrupt the normal endothelial monolayer’s specular reflection. This produces a roughened surface with a specular reflection similar to beaten metal in appearance. Eventually, this process expands from the center of the cornea to involve the corneal periphery. As the disorder progresses, the endothelial monolayer becomes attenuated in thickness, with an increase in average cell size (polymegathism), a decrease in the percentage of hexagonal shaped cells, and an increase in the coefficient of variation in cell size (pleomorphism). In the last stages of the dystrophy, effacement of the endothelium results in overlying stromal edema. At this point, the endothelium becomes more difficult to observe using conventional specular microscopy, but it may still be visualized using confocal microscopy.
As endothelial function progressively declines, the fluid accumulated in the stroma during nighttime lid closure is removed at a reduced rate, which results in significant stromal edema upon awakening. This heralds the onset of stage 2 . Patients note blurred vision, glare, and colored halos around lights. Initially, the stromal edema is localized in front of Descemet’s and behind Bowman’s membrane. Eventually, the entire stroma swells, taking on a ground-glass appearance. With the increase in corneal thickness, the posterior stroma and Descemet’s membrane are thrown into folds. Vision at this time is variable.
With progressive endothelial dysfunction, bulk fluid flow across the cornea results in microcystic and bullous epithelial edema. This development represents stage 3 of the disease. With involvement of the epithelial layer, the optical quality of the tear–air interface is severely degraded, which produces a profound reduction in vision. With the onset of epithelial edema, basal adhesion complexes become disrupted to produce recurrent corneal erosions. As a slit-lamp marker of recurrent epithelial sloughing, duplication of basement layers occurs, which creates fingerprint and map changes.
If erosions are prominent, a vascular pannus between epithelium and Bowman’s membrane may be induced and results in an anterior stromal haze, with further reduction in vision, representing stage 4 of the disease. However, the associated secondary fibrotic layer produced within the pannus often reduces or eliminates the painful recurrent epithelial erosions experienced by the patient. With the increase in stromal edema, glycosaminoglycans elute from the stroma, causing disorganization of the collagen fibrils, which contributes to additional stromal opacification.
Diagnosis and Ancillary Testing
The earliest observable change suggestive of FD is the presence of guttae on slit-lamp examination (see Fig. 4.21.1 ). Specular microscopy provides endothelial cell counts, as well as a photographic record that can be a useful educational aid for the patient ( Fig. 4.21.2 ). Subtle stromal edema can be observed using sclerotic scatter techniques. Corneal pachymetry documents increased corneal thickness. As the disease progresses, more obvious signs may develop, which include folds in Descemet’s membrane, stromal haze, microcystic and bullous epithelial edema, subepithelial fibrosis, and pannus formation. When corneal opacification precludes specular microscopy, confocal microscopy can be used to image the endothelium and obtain reliable endothelial cell counts.

Differential Diagnosis
Differential diagnosis includes posterior polymorphous corneal dystrophy, congenital hereditary endothelial dystrophy, aphakic or pseudo-phakic bullous keratopathy, and Hassall–Henle bodies. No associated systemic diseases exist.
Pathology
Light microscopy findings include a thickened Descemet’s membrane, which may be laminated in appearance with buried guttae, guttae on the surface, or devoid of guttae but thickened ( Fig. 4.21.3 ). The endothelial layer is attenuated. Electron microscopy in FD shows characteristic thickening of Descemet’s membrane caused by the deposition of an additional posterior banded layer (PBL), posterior to the posterior nonbanded layer. The PBL is markedly thickened and contains abnormally deposited collagen and the classic posterior excrescences, guttae. The production of this morphologically abnormal Descemet’s membrane serves as a marker for a dysfunctional endothelium.


Treatment
Early stages of the disease may be managed medically, temporizing the need for a keratoplasty. Medical management includes the use of hypertonic solutions or ointments and decreasing ambient humidity. If intraocular pressure (IOP) is above 20 mm Hg (2.67 kPa), attempts to lower it may reduce the force that drives fluid into the stroma. Treatment measures for painful erosions include hypertonics, bandage contact lenses, anterior stromal puncture, and conjunctival flaps.
With progressive corneal edema refractory to medical management, keratoplasty usually is offered. PKP was traditionally performed for the treatment of advanced cases. If the patient shows signs of visually significant cataracts, keratoplasty may be combined with cataract extraction. In recent years, endothelial keratoplasty, including Descemet’s stripping automated endothelial keratoplasty (DSAEK or DSEK) and Descemet’s membrane endothelial keratoplasty (DMEK), has been performed as an alternative to conventional full-thickness corneal transplantation for the treatment of endothelial disorders. In fact, endothelial keratoplasties represented 92% of the total keratoplasties performed in 2015 in patients with FD. The DSAEK procedure involves replacing the diseased endothelium and deep stroma with a posterior lamellar disc of tissue, including donor corneal endothelium, Descemet’s membrane, and posterior corneal stroma. DMEK involves replacing the diseased tissue with just the donor endothelium and Descemet’s membrane. Both DSAEK and DMEK have been demonstrated to be superior to PKP in terms of earlier visual recovery, postoperative refractive outcomes, wound- and suture-related complications, and intraoperative and late suprachoroidal hemorrhage risk. Studies have reported faster visual rehabilitation and better best corrected visual acuity with DMEK compared with DSAEK. With DMEK, patients also had a lower rate of endothelial rejection (1%) at 2 years compared with DSAEK (9%) and PKP (17 %).
Course and Outcome
Long-term outcomes of patients with FD undergoing PKP have shown that graft clarity approached 90%, and approximately 60% of patients achieved 20/40 (6/12) or better visual acuity 5 years after transplantation. Results after DSAEK have shown that average best-corrected visual acuity (BCVA; mean 9 months after surgery) ranged from 20/34 to 20/66. One year after DMEK surgery, patients had an average BCVA of 20/24, with 98% of patients achieving 20/30 or better. Reports of graft survival rates at 5 years for DMEK and DSAEK were similar to those reported for PKP in patients with FD (95%, 95%, and 93% respectively).
Introduction
Fuchs’ endothelial corneal dystrophy (FD) is a bilateral, noninflammatory, progressive loss of endothelial cells that results in corneal edema and reduction of vision. Its key features include the presence of central guttae, folds in Descemet’s membrane, stromal edema, and microcystic epithelial edema. Corneal endothelial degeneration is the primary defect that leads to the development of corneal edema. Associated features include prominent corneal nerves, stromal opacification, recurrent corneal erosions, female gender predominance, and familial predisposition.
Epidemiology and Pathogenesis
FD is the most common corneal dystrophy to require keratoplasty, accounting for approximately 3.1% of all penetrating keratoplasties (PKPs) and 47.1% of all endothelial keratoplasties in the United States in 2015. The genetic basis of FD is complex and heterogeneous, demonstrating variable expressivity and incomplete penetrance. FD is likely to be complex in etiology, with genetic as well as environmental factors playing a role in its pathogenesis. A significant variation occurs in expressivity between males and females, with a 3–4 : 1 female/male ratio recorded at the time of keratoplasty. It is equally common among white and black patients who undergo keratoplasty but is relatively rare in Asian patients.
Development of guttae and the onset of symptoms are more common in middle age. Patients with FD are believed to have an increased incidence of open-angle glaucoma. Short axial length, shallow anterior chamber, and angle-closure glaucoma also have been seen in conjunction with FD. FD has been associated with keratoconus. The progressive loss of endothelial cell function is primary in nature rather than secondary to any alteration in aqueous humor flow rate or constituency. Endothelial dysfunction is mainly a result of a reduction in sodium, potassium–adenosine triphosphatase (Na + ,K + –ATPase) pump activity, which leads to a reduction in ion flux across the endothelium. Molecular biological studies of corneal buttons from patients with FD suggest that apoptosis may play an important role in endothelial cell degeneration. Recent studies have demonstrated an oxidant–antioxidant imbalance in FD cells, which, in turn, leads to oxidative DNA damage and apoptosis.
Several genetic loci have been identified in patients with FD. The first genetic locus was mapped to chromosome 13, called Fuchs’ corneal dystrophy locus 1 (FCD1). Subsequently, three other loci, FCD2, FCD3, and FCD4, were mapped to chromosomes 18, 5, and 9, respectively. Additionally, other reports have provided evidence for potential linkage to chromosomes 1, 7, 15, 17, and X by genome-wide linkage analysis.
Multiple causal genetic mutations have been linked to FD. Mutations in the ion cotransporter encoded by the SLC4A11 gene, also associated with congenital hereditary endothelial dystrophy, have been linked to the development of FD. Additionally, mutations in the transcription factor TCF8 gene, also associated with posterior polymorphous corneal dystrophy, have been identified in patients with FD. A third causal gene for FD, LOXHD1 , was identified on chromosome 18. This gene has been associated with the development of progressive hearing loss in humans.
A strong association has been discovered between FD and trinucleotide repeats (TNRs) in the transcription factor 4 ( TCF4 ) gene in chromosome 18. This gene encodes the E2-2 transcription factor, which is involved in cellular growth and differentiation. Expansion of TNR lead to messenger RNA (mRNA) missplicing, which leads to RNA toxicity. TNR expansion in TCF4 of more than 50 is highly specific for FD. Changes to the endothelial barrier function, a known event in the development of FD, was identified as a key biological process influenced by the missplicing events.
Mutations in the gene COL8A2 that codes for the α 2 -chain of type VIII collagen have been reported in patients with an early onset endothelial dystrophy that was previously considered an early form of FD. Recent studies suggest that the endothelial dystrophy linked to mutations in COL8A2 represents a disease that is phenotypically distinct from FD.
Ocular Manifestations
The earliest slit-lamp finding in FD is the presence of focal excrescences of extracellular matrix in Descemet’s membrane, called guttae (cornea guttata). In the earliest stages of this disease, the guttae first emerge in the central corneal endothelium ( Fig. 4.21.1 ). Guttae are not specific to FD and may be seen in asymptomatic patients and in the setting of both uveitis and nonspecific superficial keratopathies. Up to 11% of eyes in patients older than age 50 years have guttae. Pathologically identical lesions in peripheral Descemet’s membrane are known as Hassall–Henle warts and are part of the normal aging process (see Chapter 4.22 ).

The guttae initially appear on specular reflection as scattered, discrete, isolated dark structures, smaller than an individual endothelial cell. An associated fine pigment dusting may be observed within the central endothelium. At this stage, referred to as stage 1 , the patient’s vision usually is normal, and the stroma and epithelium are uninvolved. Over time, these individual excrescences increase in number, enlarge, and fuse with adjacent guttae to disrupt the normal endothelial monolayer’s specular reflection. This produces a roughened surface with a specular reflection similar to beaten metal in appearance. Eventually, this process expands from the center of the cornea to involve the corneal periphery. As the disorder progresses, the endothelial monolayer becomes attenuated in thickness, with an increase in average cell size (polymegathism), a decrease in the percentage of hexagonal shaped cells, and an increase in the coefficient of variation in cell size (pleomorphism). In the last stages of the dystrophy, effacement of the endothelium results in overlying stromal edema. At this point, the endothelium becomes more difficult to observe using conventional specular microscopy, but it may still be visualized using confocal microscopy.
As endothelial function progressively declines, the fluid accumulated in the stroma during nighttime lid closure is removed at a reduced rate, which results in significant stromal edema upon awakening. This heralds the onset of stage 2 . Patients note blurred vision, glare, and colored halos around lights. Initially, the stromal edema is localized in front of Descemet’s and behind Bowman’s membrane. Eventually, the entire stroma swells, taking on a ground-glass appearance. With the increase in corneal thickness, the posterior stroma and Descemet’s membrane are thrown into folds. Vision at this time is variable.
With progressive endothelial dysfunction, bulk fluid flow across the cornea results in microcystic and bullous epithelial edema. This development represents stage 3 of the disease. With involvement of the epithelial layer, the optical quality of the tear–air interface is severely degraded, which produces a profound reduction in vision. With the onset of epithelial edema, basal adhesion complexes become disrupted to produce recurrent corneal erosions. As a slit-lamp marker of recurrent epithelial sloughing, duplication of basement layers occurs, which creates fingerprint and map changes.
If erosions are prominent, a vascular pannus between epithelium and Bowman’s membrane may be induced and results in an anterior stromal haze, with further reduction in vision, representing stage 4 of the disease. However, the associated secondary fibrotic layer produced within the pannus often reduces or eliminates the painful recurrent epithelial erosions experienced by the patient. With the increase in stromal edema, glycosaminoglycans elute from the stroma, causing disorganization of the collagen fibrils, which contributes to additional stromal opacification.
Diagnosis and Ancillary Testing
The earliest observable change suggestive of FD is the presence of guttae on slit-lamp examination (see Fig. 4.21.1 ). Specular microscopy provides endothelial cell counts, as well as a photographic record that can be a useful educational aid for the patient ( Fig. 4.21.2 ). Subtle stromal edema can be observed using sclerotic scatter techniques. Corneal pachymetry documents increased corneal thickness. As the disease progresses, more obvious signs may develop, which include folds in Descemet’s membrane, stromal haze, microcystic and bullous epithelial edema, subepithelial fibrosis, and pannus formation. When corneal opacification precludes specular microscopy, confocal microscopy can be used to image the endothelium and obtain reliable endothelial cell counts.

Pathology
Light microscopy findings include a thickened Descemet’s membrane, which may be laminated in appearance with buried guttae, guttae on the surface, or devoid of guttae but thickened ( Fig. 4.21.3 ). The endothelial layer is attenuated. Electron microscopy in FD shows characteristic thickening of Descemet’s membrane caused by the deposition of an additional posterior banded layer (PBL), posterior to the posterior nonbanded layer. The PBL is markedly thickened and contains abnormally deposited collagen and the classic posterior excrescences, guttae. The production of this morphologically abnormal Descemet’s membrane serves as a marker for a dysfunctional endothelium.


Treatment
Early stages of the disease may be managed medically, temporizing the need for a keratoplasty. Medical management includes the use of hypertonic solutions or ointments and decreasing ambient humidity. If intraocular pressure (IOP) is above 20 mm Hg (2.67 kPa), attempts to lower it may reduce the force that drives fluid into the stroma. Treatment measures for painful erosions include hypertonics, bandage contact lenses, anterior stromal puncture, and conjunctival flaps.
With progressive corneal edema refractory to medical management, keratoplasty usually is offered. PKP was traditionally performed for the treatment of advanced cases. If the patient shows signs of visually significant cataracts, keratoplasty may be combined with cataract extraction. In recent years, endothelial keratoplasty, including Descemet’s stripping automated endothelial keratoplasty (DSAEK or DSEK) and Descemet’s membrane endothelial keratoplasty (DMEK), has been performed as an alternative to conventional full-thickness corneal transplantation for the treatment of endothelial disorders. In fact, endothelial keratoplasties represented 92% of the total keratoplasties performed in 2015 in patients with FD. The DSAEK procedure involves replacing the diseased endothelium and deep stroma with a posterior lamellar disc of tissue, including donor corneal endothelium, Descemet’s membrane, and posterior corneal stroma. DMEK involves replacing the diseased tissue with just the donor endothelium and Descemet’s membrane. Both DSAEK and DMEK have been demonstrated to be superior to PKP in terms of earlier visual recovery, postoperative refractive outcomes, wound- and suture-related complications, and intraoperative and late suprachoroidal hemorrhage risk. Studies have reported faster visual rehabilitation and better best corrected visual acuity with DMEK compared with DSAEK. With DMEK, patients also had a lower rate of endothelial rejection (1%) at 2 years compared with DSAEK (9%) and PKP (17 %).
Course and Outcome
Long-term outcomes of patients with FD undergoing PKP have shown that graft clarity approached 90%, and approximately 60% of patients achieved 20/40 (6/12) or better visual acuity 5 years after transplantation. Results after DSAEK have shown that average best-corrected visual acuity (BCVA; mean 9 months after surgery) ranged from 20/34 to 20/66. One year after DMEK surgery, patients had an average BCVA of 20/24, with 98% of patients achieving 20/30 or better. Reports of graft survival rates at 5 years for DMEK and DSAEK were similar to those reported for PKP in patients with FD (95%, 95%, and 93% respectively).
Congenital Hereditary Endothelial Dystrophy
Introduction
First described by Maumenee in 1960, congenital hereditary endothelial dystrophy (CHED) is but one of the many causes of bilateral corneal clouding in full-term infants and usually requires keratoplasty. Key features of this autosomal dominant or recessive condition are a corneal thickness 2–3 times normal, normal IOP, and normal corneal diameter. Associated features are corneal pannus, nystagmus, and esotropia.
Epidemiology and Pathogenesis
Prevalence, incidence, and gender distribution for this disorder are unknown. The onset is usually at birth in a term infant; corneal clouding may be maximal at birth or progress over a period of years. Family pedigree studies support that autosomal dominant (CHED1) and recessive (CHED2) forms exist, as well as sporadic occurrences. Autosomal recessive inheritance is associated with bilateral corneal edema without photophobia but with nystagmus that is present at birth. Autosomal dominant inheritance is associated with the progressive onset of corneal edema 1–2 years post partum with photophobia but without nystagmus. Autosomal dominant and autosomal recessive forms of CHED have been linked to chromosome 20, mapped to the loci 20p11.2-q11.2 and 20p13, respectively. Mutations in the SLC4A11 gene in chromosome 20 have been highly associated with the development of autosomal recessive CHED. A related X-linked endothelial dystrophy has been described in males that clinically resembles CHED very closely.
CHED is believed to result from abnormal neural crest cell terminal induction during the late term to perinatal period. At this time, failure to complete final differentiation of the endothelial monolayer occurs, which results in a dysfunctional endothelium. This dysfunctional endothelium is believed to have faulty growth regulation mechanisms that lead to accumulation of a functionally abnormal and structurally exaggerated form of posterior nonbanded Descemet’s membrane.
Ocular Manifestations
The usual presentation is bilateral, symmetrically edematous, cloudy corneas evident at birth or early in the postnatal period. Examination reveals the corneas to have a diffuse gray-blue, ground-glass coloring. Corneal thickness is 2–3 times normal and often greater than 1 mm centrally. Both IOP and horizontal corneal diameter are normal. Rarely, CHED is associated with glaucoma and should be considered if corneal opacification fails to resolve after normalization of IOP.
Closer examination reveals the texture of the epithelial surface to be irregular, with a diffuse pigskin-like roughness. Occasionally, discrete white dots may be seen in the stroma. In areas where stromal opacification is less dense, Descemet’s membrane appears gray, and on specular reflection may have a peau d’orange texture. The endothelial layer may or may not be visualized. A fine corneal pannus may be seen, as well as low-grade inflammation.
Diagnosis
A tentative diagnosis usually is possible when examination under anesthesia demonstrates typical bilateral stromal opacification, gross corneal thickening, normal horizontal diameter, normal IOP, and absence of breaks in Descemet’s membrane.
Differential Diagnosis
Differential diagnosis includes congenital glaucoma without buphthalmos, posterior polymorphous corneal dystrophy, macular stromal dystrophy, mucopolysaccharidosis, intra-uterine infection, and birth trauma from forceps. Harboyan’s syndrome is an entity defined as CHED accompanied by progressive, sensorineural hearing loss. This disorder has been linked to the SLC4A11 gene mutation.
Pathology
Light microscopy shows epithelial atrophy with basal cell hydrops, subepithelial calcification or fibrosis, patchy loss of Bowman’s membrane, and variable vascularization or spheroidal degeneration of the stroma. Descemet’s membrane is thickened, often with discrete laminations. The endothelial layer is attenuated.
Treatment
If the edema is stationary and mild, use of hypertonics and desiccating measures may be employed. Usually, however, these patients require keratoplasty because of the bilateral nature of the corneal edema. Keratoplasty in infants and children is a high-risk procedure and is technically difficult, and the long-term prognosis for graft clarity is worse than it is for adults. No definitive clinical guidelines have emerged with regard to the timing of surgical intervention, as a result of significant heterogeneity in disease severity, follow-up periods, and patient ages at both diagnosis and surgery, among the few published studies. Delayed PKP (after age 12 years) may offer better graft outcomes and visual prognosis in patients with CHED, even in the presence of nystagmus, according to a publication. DSAEK has been performed in recent years as a therapeutic alternative for CHED. DSAEK performed in eyes with CHED has allowed rapid restoration of corneal clarity while minimizing intraoperative and postoperative complications normally associated with pediatric PKP.
The decision regarding surgery may be difficult because despite significant corneal haze and absence of a red reflex, patients often seem to see much better than expected. If patients maintain good fixation with normal alignment, surgery may be delayed; loss of fixation or development of nystagmus may lead to earlier intervention.
Course and Outcome
In one large study, during a mean follow-up period of over 70 months, 69% of eyes retained full graft clarity. Postoperative visual acuity improvement of 1 or more Snellen lines was seen in 5 of 10 eyes in which the patients were old enough for accurate assessment of visual acuity; however, just four of these 10 eyes attained a visual acuity of 20/200 or better. First graft survival rates range from 25% at 3 months in earlier studies to 62%–90% at 2–3 years in more recent series.
Results from a small series of patients older than age 12 months who underwent DSAEK revealed that BCVA of 20/40 or better was achieved in 8 of the 9 patients, and visual acuity of 20/70 was achieved in the remaining patient. In the infant group, the three patients who had DSAEK were able to “fix and follow” after the procedure.
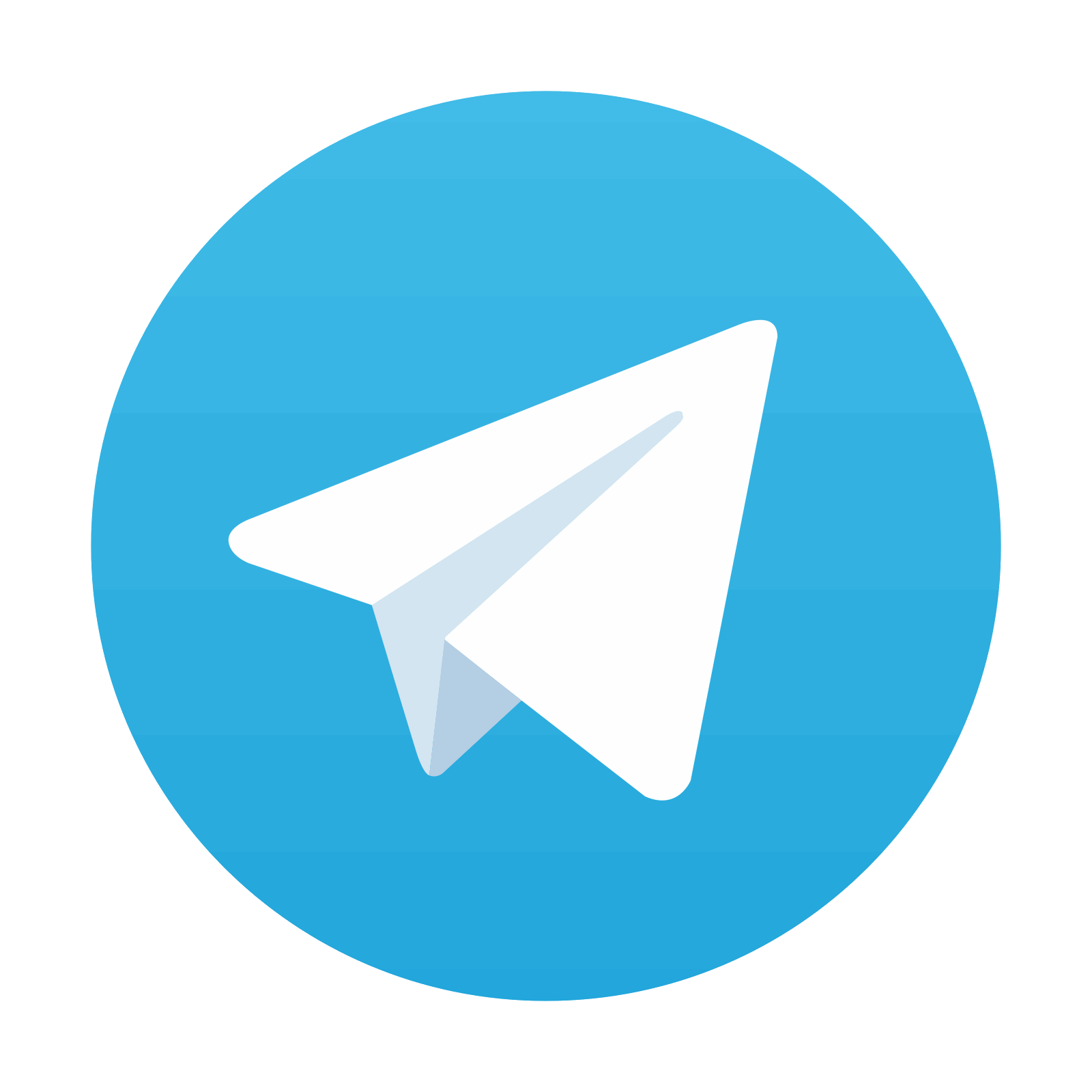
Stay updated, free articles. Join our Telegram channel

Full access? Get Clinical Tree
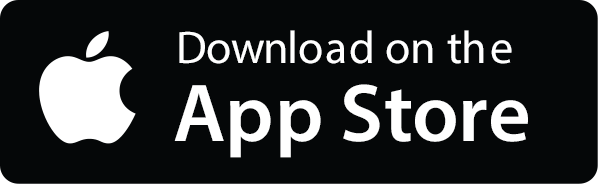
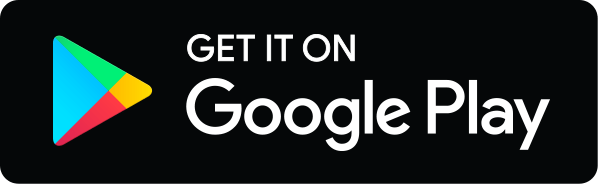