Purpose
To determine the diagnostic capability of spectral-domain optical coherence tomography (SD OCT) peripapillary retinal thickness (RT) measurements from 3-dimensional (3D) volume scans for primary open-angle glaucoma (POAG).
Design
Cross-sectional study.
Methods
setting : Institutional. study population : 156 patients (89 POAG and 67 normal subjects). observation procedures : One eye of each subject was included. SD OCT peripapillary RT values from 3D volume scans were calculated for 4 quadrants of 3 different sized annuli. Peripapillary retinal nerve fiber layer (RNFL) thickness values were also determined. main outcome measures : Area under the receiver operating characteristic curve (AUROC) values, sensitivity, specificity, positive and negative predictive values, and positive and negative likelihood ratios.
Results
The top 5 RT AUROCs for all glaucoma patients and for a subset of early glaucoma patients were for the inferior quadrant of outer circumpapillary annulus of circular grid (OCA) 1 (0.959, 0.939), inferior quadrant of OCA2 (0.945, 0.921), superior quadrant of OCA1 (0.890, 0.811), inferior quadrant of OCA3 (0.887, 0.854), and superior quadrant of OCA2 (0.879, 0.807). Smaller RT annuli OCA1 and OCA2 consistently showed better diagnostic performance than the larger RT annulus OCA3. For both RNFL and RT measurements, best AUROC values were found for inferior RT OCA1 and OCA2, followed by inferior and overall RNFL thickness.
Conclusion
Peripapillary RT measurements from 3D volume scans showed excellent diagnostic performance for detecting both glaucoma and early glaucoma patients. Peripapillary RT values have the same or better diagnostic capability compared to peripapillary RNFL thickness measurements, while also having fewer algorithm errors.
Glaucoma is the leading cause of irreversible blindness worldwide, and it results in a characteristic optic neuropathy with corresponding visual field defects. Clinical evaluation of the optic nerve head (ONH) and retinal nerve fiber layer (RNFL) is highly observer dependent and is primarily limited by large interobserver variation. Because nerve tissue loss in glaucoma is irreversible and up to 50% of nerve tissue may be lost by the time there is evidence of visual field damage, there is a need to better assess nerve tissue loss more objectively and quantitatively both for diagnosing glaucoma and for evaluating glaucoma progression.
Imaging devices can assess nerve tissue objectively and quantitatively, and optical coherence tomography (OCT) is the most widely used imaging technology for the evaluation of the ONH, RNFL, and ganglion cell region in glaucoma. The Spectralis machine (Heidelberg Engineering, Inc, Heidelberg, Germany) is one of the many commercially available spectral domain (SD) OCT instruments, and it acquires 40 000 A-scans per second with an axial resolution of 7 μm, a lateral resolution of 14 μm, and a scan depth of 1.8 mm. SD OCT machines can measure glaucomatous nerve damage by evaluating different structural parameters, which include the following: RNFL thickness, ganglion cell layer thickness, macular thickness, and ONH parameters (eg, rim area, rim volume, cup volume). The most popular glaucoma parameter in commercially available SD OCT machines is RNFL thickness, and thinning of the RNFL is a strong indicator of glaucoma.
The reliability of OCT RNFL measurements decreases in glaucoma where the RNFL is thinner. Since the coefficient of variation is reciprocally related to reliability, the coefficient of variation is greater in glaucoma patients. One of the main reasons for inaccurate OCT RNFL measurements in glaucoma is that the reflectivity of the RNFL decreases in glaucoma, which makes it difficult for segmentation algorithms to differentiate the normally highly reflective RNFL layer from the underlying tissue. Also, it is difficult to assess glaucomatous RNFL thinning in myopic patients, because nearly half of myopic patients without glaucoma are classified as abnormal. Another factor that affects RNFL thickness measurements is peripapillary atrophy (PPA). Complete loss of the retinal pigment epithelium occurs in patients with ß-zone PPA, and some of the other retinal layers may also terminate in the region of ß-zone PPA, before reaching the disc margin. Although the continuity of the RNFL is not disturbed in PPA, diagnostic performance of RNFL thickness within PPA is decreased. Both PPA and myopia have been associated with glaucoma. Asrani and associates reported that artifacts for RNFL measurements can occur in 19.9% of images. Misalignment artifacts for RNFL measurements can occur in as much as 46.7% of SD OCT images. In addition, the rate of false positives using RNFL thickness for the diagnosis of glaucoma has been reported to be between 18% and 39%.
Because of the inherent difficulties in measuring RNFL thickness in glaucoma patients (owing to decreased RNFL reflectivity, myopia, and PPA), we believe that peripapillary retinal thickness (RT) may provide improved diagnostic performance for glaucoma and may be more easily measured in a greater percentage of glaucoma patients. Although peripapillary RT is a useful metric in diabetic retinopathy and radiation optic neuropathy, and although the effect of acute intraocular pressure elevation on peripapillary RT in monkeys has been evaluated, the diagnostic capability of peripapillary RT in glaucoma patients has not yet been studied using 3D volume scans. Past publications by our group have suggested that RT may be useful in glaucoma diagnosis. Therefore, in this study, we evaluated the diagnostic capability of peripapillary RT from SD OCT volume scans of the peripapillary region in primary open-angle glaucoma (POAG) patients, who were imaged with the Spectralis machine.
Subjects and Methods
Participants and Examinations
All study subjects were recruited from the Glaucoma Service at the Massachusetts Eye and Ear Infirmary between January 2009 and July 2013, as part of the prospective SIG (Spectral Domain OCT in Glaucoma) Study, which was approved by the Massachusetts Eye and Ear Infirmary institutional review board. Informed consent form was obtained from all the subjects participating in the study. All methods adhered to the tenets of the Declaration of Helsinki for research involving human subjects, and the study was conducted in accordance with Health Insurance Portability and Accountability Act regulations. All study subjects underwent a complete eye examination by a glaucoma specialist (T.C.C.), and this included history, visual acuity testing, refraction, Goldmann applanation tonometry, slit-lamp biomicroscopy, gonioscopy, ultrasonic pachymetry, dilated ophthalmoscopy, stereo disc photography (Visucam Pro NM; Carl Zeiss Meditec, Inc), visual field (VF) testing (Swedish Interactive Threshold Algorithm 24-2 test of the Humphrey visual field analyzer 750i; Carl Zeiss Meditec, Inc), and volume scans using Spectralis OCT (HRA/Spectralis software version 5.4.8.0, Heidelberg Engineering GmbH, Heidelberg, Germany).
Patients were included if they had a spherical equivalent between −5.0 and +5.0 diopters and a best-corrected visual acuity of 20/40 or better. Only patients with reliable VF testing were included, with less than 33% fixation losses, less than 20% false-positive results, and less than 20% false-negative results. Patients were excluded if they had discernible congenital anomalies of the anterior chamber, corneal scarring or opacities, diabetic proliferative or severe nonproliferative retinopathy, VF loss attributable to a non-glaucoma condition, or a dilated pupil diameter of less than 2 mm.
Glaucoma patients were defined as having characteristic changes of the ONH with corresponding abnormal VF defects. The VF was considered to be abnormal if 3 or more contiguous test locations in the pattern standard deviation plot were depressed significantly at the P < .05 level with at least 1 at the P < .01 level on the same side of the horizontal meridian and if the VF defect corresponded to the optic nerve appearance. Only POAG patients were included. VF abnormalities were classified as early (mean deviation [MD] >−6 decibels [dB]), moderate (−12 dB< MD ≤−6 dB), or severe (MD ≤−12 dB).
Normal subjects were those without ocular disease, except for mild cataract, and those with normal VF test results, as defined by a pattern standard deviation of more than 5% and glaucoma hemifield test results within normal limits. If both eyes were eligible for the study, 1 eye was selected randomly by using the RANDBETWEEN (min,max) function in Microsoft Office Excel 2007. We defined “1” for the “min” and “2” for the “max.” We ran the command = RANDBETWEEN (1,2) for every row of patients whose both eyes were eligible. We picked the right eye for the result “1” and left eye for the result “2.”
Spectralis Optical Coherence Tomography Peripapillary Retinal Volume Scan
After pupillary dilation, all SD OCT imaging was performed with the Spectralis OCT machine, which uses an 870-nm superluminescent diode source. Additional details of the SD OCT technique have been described elsewhere. Spectralis OCT provides an automatic real-time (ART) function with an eye-tracking system that can increase image quality. With the ART function activated, multiple frames of the same scanning location are obtained. These data then are averaged for noise reduction, and eye-motion artifacts are reduced. As suggested by the manufacturer, scans with signal strength of less than 15 dB (range, 0–40) were excluded from the analysis. In addition, the criteria for determining adequate scan quality were as follows: a clear fundus image with good optic disc and scan area visibility before and during image acquisition, overlay of volume scan visible and without interruptions, and a continuous scan pattern without missing or blank areas. The Spectralis OCT software enables volume scans, which are performed with a 20 × 20-degree field and which are centered on the ONH. One-hundred ninety-three sections were taken with the high speed rate and 3 frames for ART. All 193 B-scans for each subject were checked for algorithm artifacts and errors.
Analysis of the volume scans was performed using Heidelberg Eye Explorer version 1.7.0.0 (Heidelberg Engineering GmbH). The scan area overlay was lowered to zero, and the circular grid pattern was centered on the ONH by one of the authors (H.S.). The mean retinal thickness, determined by an internal program algorithm, was recorded in regions we term circumpapillary annuli. For these circumpapillary annuli, mean retinal thickness values are shown in black ( Figure 1 , Bottom left). For this paper, we only analyzed the outer annulus (OCA) for each of the circular grid scan options (gray areas, Figure 2 ), because the inner circular region and inner annulus covered portions of the optic nerve. The outer annuli (gray areas, Figure 2 ) were further subdivided by quadrant: superior, temporal, inferior, and nasal. For circumpapillary annulus 1 ( Figure 1 , Top left and Figure 2 , Left), the inner area is bounded by circles of diameters 1.00 mm and 2.00 mm, and the outer area by circles of diameters 2.00 mm and 3.00 mm (gray area OCA1, Figure 2 , Left). For circumpapillary annulus 2 ( Figure 1 , Top middle and Figure 2 , Center), the inner area is bounded by circles of diameters 1.00 mm and 2.22 mm, and the outer area by circles of diameters 2.22 mm and 3.45 mm (gray area OCA2, Figure 2 , Center). For circumpapillary annulus 3 ( Figure 1 , Top right and Figure 2 , Right), the inner area was bounded by circles of diameters 1.00 mm and 3.00 mm, and the outer area by circles of diameters 3.00 mm and 6.00 mm (gray area OCA3, Figure 2 , Right). Throughout the rest of the paper, the outer circumpapillary annuli of circular grids 1, 2, and 3 will be called OCA1, OCA2, and OCA3, respectively. If parts of OCA1, OCA2, or OCA3 extended outside the 20 × 20-degree field, these areas were also excluded from the final data analysis.
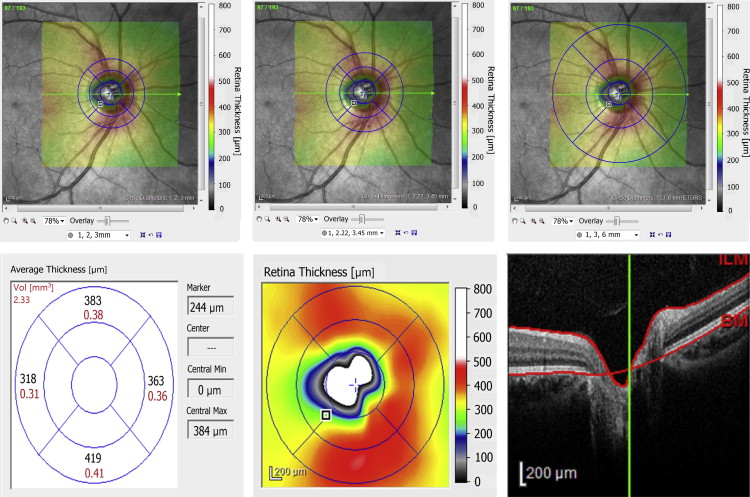
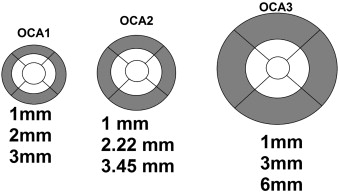
The average RNFL thickness values for the overall RNFL (360 degrees), for each 90-degree quadrant (superior, temporal, inferior, and nasal), and for each sector (superior-temporal [TS], superior nasal [NS], inferior-temporal [TI], and inferior-nasal [NI]) were recorded from the Spectralis OCT RNFL printouts.
Statistical Analysis
All of the calculations in this article were performed using MedCalc for Windows, version 11.4.2 (MedCalc Software, Ostend, Belgium). Demographic characteristics of the normal and glaucoma groups were compared using χ 2 tests for categorical variables and nonpaired 2-tailed Student t tests for continuous variables. Receiver operating characteristic (ROC) curves were created, and areas under the receiver operating characteristic (AUROC) curves were calculated for the retinal volume scans of the 4 quadrants (ie, superior, temporal, inferior, and nasal) for each of the OCA1, OCA2, and OCA3. Sensitivity, specificity, positive predictive value (PPV), negative predictive value (NPV), positive likelihood ratio (PLR), and negative likelihood ratio (NLR) were calculated. The cutoff value for each variable is calculated by the statistical program automatically, depending on the maximum value of the Youden index (J), which is equal to the maximum of [Sensitivity + Specificity − 1]. This value corresponds with the point on the ROC curve furthest from the diagonal line. Pair-wise comparisons of ROC curves were performed to determine which quadrant had higher accuracy in the diagnosis of glaucoma. False-positive and false-negative values were evaluated with respect to the cutoff value. Normal subjects who had RT values smaller than the cutoff value were counted as false positives, and POAG patients who had RT values larger than the cutoff were counted as false negatives. The effects of PPA on false-positive and false-negative determinations were calculated by χ 2 tests.
ROC curves for RNFL thickness values were created, and AUROC curves were calculated for overall RNFL, the 4 quadrants, and the 4 sectors. Sensitivity, specificity, PPV, NPV, PLR, and NLR were calculated as described above. Pair-wise comparisons of ROC curves were performed for the best AUROC curve values for RT and RNFL thickness measurements, both for all POAG patients and for the early POAG patient subset. Differences were considered significant at P values <.05.
Results
There were 156 study patients, with 67 normal subjects and 89 POAG subjects. Of the 89 POAG subjects, 33 of them had early glaucoma (37.0%), 24 of them had moderate glaucoma (27.0%), and 32 of them had severe glaucoma (36.0%), which represents an equal distribution. Demographics of the study population are shown in Table 1 . All 193 B-scans for each subject were checked for algorithm artifacts and errors, and no segmentation errors were noted for any of the included automated RT determinations. For all quadrants of RT annuli OCA1, OCA2, and OCA3, POAG and early POAG patients had thinner retinas compared to normal patients ( P < .0001 and P < .01, respectively, Table 2 ).
Normal | POAG | P a | Early POAG | P b | |
---|---|---|---|---|---|
Number of eyes | 67 | 89 | 33 | ||
Number of right eyes/left eyes | 37/30 | 51/38 | .871 | 22/11 | .190 |
Mean age (y) | 62.6 ± 11.6 | 66.0 ± 10.6 | .055 | 67.1 ± 7.9 | .051 |
Refractive error (D) | |||||
Sphere | 0.008 ± 1.41 | 0.04 ± 1.23 | .878 | −0.19 ± 1.01 | .500 |
Cylinder | −0.57 ± 0.74 | −0.45 ± 0.81 | .371 | −0.74 ± 0.57 | .095 |
Spherical equivalent | −0.28 ± 1.52 | −0.19 ± 1.18 | .678 | 1.52 ± 1.01 | .828 |
VF | |||||
Mean deviation (dB) | −1.92 ± 1.99 | −11.20 ± 7.92 | <.0001 | −3.97 ± 1.50 | <.001 |
Pattern standard deviation (dB) | 1.84 ± 0.70 | 7.01 ± 3.35 | <.0001 | 4.40 ± 1.77 | <.001 |
Normal | POAG a | Early POAG b | |
---|---|---|---|
Mean ± SD (μm) | Mean ± SD (μm) | Mean ± SD (μm) | |
OCA1 | |||
Superior | 356.0 ± 47.3 | 289.4 ± 38.0 | 311.15 ± 32.74 |
Temporal | 308.7 ± 28.9 | 264.4 ± 32.9 | 275.42 ± 33.51 |
Inferior | 352.7 ± 28.8 | 278.0 ± 31.2 | 292.39 ± 23.14 |
Nasal | 303.4 ± 26.8 | 270.3 ± 29.0 | 283.91 ± 20.50 |
OCA2 | |||
Superior | 344.9 ± 45.2 | 288.3 ± 31.3 | 304.55 ± 28.81 |
Temporal | 306.1 ± 26.1 | 270.7 ± 26.2 | 279.52 ± 26.70 |
Inferior | 341.5 ± 26.2 | 278.7 ± 27.1 | 290.24 ± 22.42 |
Nasal | 297.8 ± 24.1 | 272.7 ± 26.6 | 282.21 ± 19.90 |
OCA3 | |||
Superior | 295.2 ± 23.5 | 265.2 ± 21.9 | 269.80 ± 19.08 |
Temporal | 290.5 ± 31.0 | 266.6 ± 25.3 | 273.52 ± 27.41 |
Inferior | 290.8 ± 21.4 | 255.0 ± 19.6 | 261.36 ± 17.49 |
Nasal | 285.0 ± 29.7 | 262.8 ± 28.6 | 264.17 ± 24.79 |
When analyzing OCA1 and OCA2, there were no subjects who were excluded owing to OCA1 and OCA2 being outside the 20 × 20-degree field scan area. When analyzing OCA3, 38 of 156 subjects (23.7%) were excluded from the analysis, because the 20 × 20-degree scan area did not fully cover the ringed area for OCA3. Twenty-one of these incomplete scanned regions were in the superior quadrant, 24 of them were in the nasal quadrant, 6 of them were in the temporal quadrant, and 3 of them were in the inferior quadrant ( Figure 1 ).
Table 3 shows the AUROC curve values and the RT cutoff values that are associated with the highest sensitivity and specificity values for distinguishing normal patients from POAG and early POAG patients. The highest 2 AUROC curves for distinguishing between normal and both POAG and early POAG patients were both associated with the inferior region (ie, inferior OCA1 [0.959, 0.939] and inferior OCA2 [0.945, 0.921]). Pair-wise comparisons of ROC curves also revealed that inferior OCA1 and inferior OCA2, which were the highest 2 ranked AUROC curves, had statistically better diagnostic performance than other quadrants of OCAs for both POAG and early POAG patients.
Normal vs POAG | Normal vs Early POAG | |||
---|---|---|---|---|
AUROC (SE) | Cutoff μm (≤) | AUROC (SE) | Cutoff μm (≤) | |
OCA1 | ||||
Superior | 0.890 (0.0283) | 318 | 0.811 (0.0464) | 344 |
Temporal | 0.842 (0.0336) | 292 | 0.777 (0.0500) | 292 |
Inferior | 0.959 (0.0176) | 318 | 0.939 (0.0234) | 322 |
Nasal | 0.806 (0.0368) | 295 | 0.725 (0.0512) | 292 |
OCA2 | ||||
Superior | 0.879 (0.0296) | 320 | 0.807 (0.0463) | 310 |
Temporal | 0.832 (0.0346) | 293 | 0.765 (0.0516) | 293 |
Inferior | 0.945 (0.0203) | 309 | 0.921 (0.0290) | 322 |
Nasal | 0.758 (0.0402) | 288 | 0.693 (0.0539) | 304 |
OCA3 | ||||
Superior | 0.835 (0.0346) | 289 | 0.799 (0.0455) | 288 |
Temporal | 0.727 (0.0420) | 268 | 0.659 (0.0585) | 268 |
Inferior | 0.887 (0.0287) | 268 | 0.854 (0.0414) | 267 |
Nasal | 0.717 (0.0452) | 270 | 0.717 (0.0590) | 270 |
In quadrant-based pair-wise comparisons of ROC curves of normal vs POAG patients, OCA1 had better diagnostic capability than OCA3 for all quadrants ( P superior = .0051, P temporal = .0020, P inferior = .0001, P nasal = .0031). OCA2 had better diagnostic capability than OCA3 for all quadrants ( P superior = .0031, P temporal = .0028, P inferior < .0001, and P nasal = .0012). AUROC curve values are higher in OCA1 than in OCA2. There is no difference found between ROC curves of OCA1 and OCA2 except in the nasal quadrant ( P superior = .0723, P temporal = .3718, P inferior = .0537, and P nasal = .0113).
In quadrant-based pair-wise comparisons of ROC curves of normal vs early POAG patients, although OCA1 had higher AUROC curve values than OCA2, there was no statistically significant difference between ROC curves ( P superior = .5986, P temporal = .5469, P inferior = .0850, and P nasal = .0799). OCA1 had higher AUROC curve values than OCA3 in all quadrants. After comparing ROC curves of OCA1 and OCA3, the diagnostic capability of OCA1 was statistically better than OCA3 for temporal and inferior quadrants ( P temporal = .0342 and P inferior = .0005). The superior and nasal quadrants of OCA1 and OCA3 had similar ROC curves ( P superior = .4784 and P nasal = .1827). After comparing ROC curves of OCA2 and OCA3, OCA2 had better ROC curves than OCA3 in the temporal and inferior quadrants ( P temporal = .0216 and P inferior = .0008) but there was no difference between the superior and nasal quadrants ( P superior = .5454 and P nasal = .3667).
The diagnostic performance of RT parameters with the best sensitivity and specificity values for distinguishing normal vs POAG patients is shown in Table 4 . The diagnostic performance of RT parameters with the best sensitivity and specificity values for distinguishing normal vs early POAG patients is shown in Table 5 .
Sensitivity (CI) | Specificity (CI) | PLR (CI) | NLR (CI) | PPV (CI) | NPV (CI) | |
---|---|---|---|---|---|---|
OCA1 | ||||||
Superior | 79.78 (69.9–87.6) | 85.07 (74.3–92.6) | 5.34 (4.6–6.2) | 0.24 (0.1–0.5) | 87.7 (78.4–94.0) | 76.0 (64.7–85.1) |
Temporal | 83.91 (74.5–90.9) | 76.12 (64.1–85.7) | 3.51 (3.0–4.1) | 0.21 (0.1–0.4) | 82.0 (72.5–89.4) | 78.5 (66.5–87.7) |
Inferior | 93.18 (85.7–97.5) | 88.06 (77.8–94.7) | 7.80 (7.0–8.7) | 0.077 (0.03–0.2) | 91.1 (83.2–96.1) | 90.8 (80.9–96.6) |
Nasal | 83.53 (73.9–90.7) | 65.67 (53.1–76.8) | 2.43 (2.0–3.0) | 0.25 (0.1–0.4) | 75.5 (65.6–83.8) | 75.9 (62.7–86.2) |
OCA2 | ||||||
Superior | 85.39 (76.3–92.0) | 77.61 (65.8–86.9) | 3.81 (3.3–4.5) | 0.19 (0.10–0.4) | 83.5 (74.2–90.5) | 80.0 (68.2–88.9) |
Temporal | 83.53 (73.9–90.7) | 77.61 (65.8–86.9) | 3.73 (3.2–4.4) | 0.21 (0.1–0.4) | 82.6 (72.8–89.9) | 78.8 (66.9–88.0) |
Inferior | 88.64 (80.1–94.4) | 89.55 (79.7–95.7) | 8.48 (7.6–9.5) | 0.13 (0.05–0.3) | 91.8 (83.7–96.6) | 85.7 (75.3–92.9) |
Nasal | 77.65 (67.3–86.0) | 62.69 (50.0–74.2) | 2.08 (1.7–2.6) | 0.36 (0.2–0.6) | 72.5 (62.1–81.4) | 68.9 (55.7–80.1) |
OCA3 | ||||||
Superior | 90.24 (81.7–95.7) | 63.64 (50.9–75.1) | 2.48 (2.0–3.0) | 0.15 (0.07–0.3) | 75.5 (65.8–83.6) | 84.0 (70.7–92.9) |
Temporal | 59.52 (48.3–70.1) | 77.61 (65.8–86.9) | 2.66 (2.1–3.3) | 0.52 (0.3–0.9) | 76.9 (64.7–86.5) | 60.5 (49.3–70.8) |
Inferior | 79.78 (69.9–87.6) | 85.07 (74.3–92.6) | 5.34 (4.6–6.2) | 0.24 (0.1–0.5) | 87.7 (78.4–94.0) | 76.0 (64.7–85.1) |
Nasal | 68.49 (56.6–78.9) | 71.67 (58.6–82.5) | 2.42 (1.9–3.0) | 0.44 (0.3–0.7) | 74.6 (62.5–84.5) | 65.2 (52.4–76.5) |
Sensitivity (CI) | Specificity (CI) | PLR (CI) | NLR (CI) | PPV (CI) | NPV (CI) | |
---|---|---|---|---|---|---|
OCA1 | ||||||
Superior | 87.88 (71.8–96.6) | 62.69 (50.0–74.2) | 2.36 (1.9–2.9) | 0.19 (0.07–0.5) | 53.7 (39.6–67.4) | 91.3 (79.2–97.6) |
Temporal | 72.73 (54.5–86.7) | 76.12 (64.1–85.7) | 3.05 (2.4–3.9) | 0.36 (0.2–0.7) | 60.0 (43.3–75.1) | 85.0 (73.4–92.9) |
Inferior | 93.94 (79.8–99.3) | 85.07 (74.3–92.6) | 6.29 (5.5–7.2) | 0.071 (0.02–0.3) | 75.6 (59.7–87.6) | 96.6 (88.2–99.6) |
Nasal | 72.73 (54.5–86.7) | 67.16 (54.6–78.2) | 2.21 (1.7–2.9) | 0.41 (0.2–0.8) | 52.2 (36.9–67.1) | 83.3 (70.7–92.1) |
OCA2 | ||||||
Superior | 63.64 (45.1–79.6) | 86.57 (76.0–93.7) | 4.74 (3.6–6.2) | 0.42 (0.2–0.9) | 70.0 (50.6–85.3) | 82.9 (71.9–90.9) |
Temporal | 69.70 (51.3–84.4) | 77.61 (65.8–86.9) | 3.11 (2.4–4.0) | 0.39 (0.2–0.8) | 60.5 (43.4–76.0) | 83.9 (72.3–92.0) |
Inferior | 90.91 (75.7–98.1) | 83.58 (72.5–91.5) | 5.54 (4.8–6.4) | 0.11 (0.03–0.4) | 73.2 (57.1–85.8) | 94.9 (85.7–99.0) |
Nasal | 87.88 (71.8–96.6) | 49.25 (36.8–61.8) | 1.73 (1.3–2.3) | 0.25 (0.1–0.6) | 46.0 (33.4–59.1) | 89.2 (74.3–97.0) |
OCA3 | ||||||
Superior | 86.67 (69.3–96.2) | 63.64 (50.9–75.1) | 2.38 (1.9–3.0) | 0.21 (0.08–0.6) | 52.0 (37.4–66.3) | 91.3 (79.0–97.6) |
Temporal | 51.52 (33.5–69.2) | 77.61 (65.8–86.9) | 2.30 (1.6–3.3) | 0.62 (0.4–1.1) | 53.1 (34.7–70.9) | 76.5 (64.6–85.9) |
Inferior | 84.85 (68.1–94.9) | 79.10 (67.4–88.1) | 4.06 (3.4–4.9) | 0.19 (0.08–0.5) | 66.7 (50.5–80.4) | 91.4 (80.9–97.2) |
Nasal | 75.86 (56.5–89.7) | 71.67 (58.6–82.5) | 2.68 (2.1–3.5) | 0.34 (0.2–0.7) | 56.4 (39.6–72.2) | 86.0 (73.3–94.2) |
We evaluated images for the presence of PPA because we wanted to determine if PPA was associated with higher rates of false-positive or false-negative diagnoses of glaucoma. In OCA1 ( Figure 2 , gray area, Left image), ß-zone PPA was detected in a total of 34.0% of patients (53 of 156 patients). In OCA2 ( Figure 2 , gray area, Center image), ß-zone PPA was detected in 21.2% of patients (33 of 156 patients). There were no patients who had PPA that extended into OCA3 ( Figure 2 , gray area, Right image). For each of the 3 scan patterns, a total of 624 quadrants (156 subjects × 4 quadrants) were analyzed. Table 6 shows that PPA was noted in 16.5% (103 of 624) of the OCA1 quadrants. The presence of PPA was not significantly correlated with a misdiagnosis of glaucoma, on the basis of false-positive and false-negative results ( P = .709, P = .239). In OCA2, PPA was noted in 8.5% (53 of 624) of quadrants. The presence of PPA again was not significantly associated with an inaccurate glaucoma diagnosis ( P = .419, P = .640). Detailed information about PPA with false-positive and false-negative rates is shown in Table 6 .
