Abstract
Purpose
To compare the accuracy for glaucomatous defects and diagnostic power for primary open-angle glaucoma (POAG) between swept-source optical coherence tomography (SS-OCT) and spectral-domain optical coherence tomography (SD-OCT) in myopic eyes.
Design
Prospective, case-control study
Methods
One hundred and fifty (150) myopic POAG eyes and 100 healthy myopic eyes underwent SD-OCT and SS-OCT in random order, on the same day. The locations of glaucomatous defect on SD-OCT thickness and deviation maps and SS-OCT wide-field thickness (thickness surface) and SuperPixel maps were rated, and the maps’ accuracies were compared. The area under receiver operating characteristic (AUROC) of the peripapillary retinal nerve fiber layer (RNFL) and the macular parameters (GCL++: equivalent to ganglion cell-inner plexiform layer [GCIPL] + RNFL; GCL+: equivalent to GCIPL) from each of the devices for myopic POAG were calculated and compared.
Results
The wide-field RNFL thickness (thickness surface) map showed the best accuracy for glaucomatous defect in the inferotemporal (96.4%) and superotemporal (92.4%) regions. The RNFL/GCL++/GCL+ wide-field thickness (thickness surface) map showed better accuracy for glaucomatous defect in both the superotemporal and inferotemporal regions compared with the SD-OCT thickness map (all P s < 0.05). The average GCL++ (87.6%) and GCL+ (87.5%) thicknesses showed significantly greater AUROC for myopic POAG than did GCIPL thickness from SD-OCT (83.8%, all P s < 0.05).
Conclusions
In myopic eyes, the SS-OCT wide-field map exhibited better accuracy for glaucomatous defect and greater diagnostic power for POAG compared with the SD-OCT outcomes. This result might have been due to SS-OCT’s wider scan and measurement area.
Introduction
The prevalence of myopia is dramatically increasing in young generations, especially in East Asians, including Koreans. The public concern for increasing myopia stems from the fact that it can lead to vision-threatening eye diseases such as retinal detachment, choroidal neovascularization, and glaucoma. In particular, many epidemiological studies have indicated increased risk of primary open-angle glaucoma (POAG) in myopic eyes. , A systematic meta-analysis supported the idea that myopia increases the risk of POAG.
Unfortunately, however, diagnosis and monitoring of POAG in myopic eyes are challenging. Myopic eyes have divergent optic disc morphology such as tilted disc, peripapillary atrophy, and chorioretinal atrophy. These structural anomalies make accurate detection of glaucomatous optic disc changes difficult. In addition, vessel temporalization during axial elongation in myopic eyes alters the peak distribution of peripapillary retinal nerve fiber layer (RNFL) thickness and causes false-positives in optical coherence tomography (OCT) measurements. ,
In response to the clinical demand for accurate diagnosis of POAG in myopic eyes, there have been many attempts to improve diagnostic power by using spectral-domain OCT (SD-OCT). The macular ganglion cell-inner plexiform layer (GCIPL) thickness and its asymmetrical difference across the horizontal raphe have shown improved diagnostic accuracy for POAG in highly myopic eyes. Also, optic disc rim measurements such as Bruch’s membrane opening-minimum rim width (BMO-MRW) from Spectralis OCT (Heidelberg Engineering, Inc., Heidelberg, Germany) or three-dimensional neuroretinal rim thickness (3D-NRT) from Cirrus HD-OCT (Carl Zeiss Meditec, Dublin, CA, USA) have exhibited greater diagnostic power for myopic POAG. ,
Recently, swept-source OCT (SS-OCT, DRI OCT Triton, Topcon, Tokyo, Japan) has been proved effective for capturing of a wide 12×9 mm area in a single scan, thanks to its higher scanning speed and longer wavelength penetration relative to SD-OCT. The wide-field map provided by SS-OCT is advantageous over conventional thickness and deviation maps from SD-OCT in that the macular and peripapillary structure can be evaluated simultaneously from a single scan. In this sense, our group reported a good diagnostic utility of the wide-field RNFL thickness map in detecting pre-perimetric and early-perimetric glaucoma eyes. ,
The present study was initiated to confirm whether the wide-field map provided by SS-OCT is useful in detecting glaucomatous changes in myopic eyes. We evaluated the wide-field GCL++ (equivalent to RNFL+GCIPL from Cirrus HD-OCT) and GCL+ (equivalent to GCIPL in Cirrus HD-OCT) maps as well as the RNFL map and compared their accuracies for detection of glaucomatous structural defect relative to RNFL/GCIPL thickness and deviation maps from Cirrus HD-OCT. In addition, the diagnostic power for myopic POAG was compared among the measurement data from the two devices.
Methods
The present study was a prospective case-control series study performed at Seoul National University Hospital (SNUH) after approval by the SNUH Institutional Review Board (IRB No.: H-1806-153-953). The participants in the study were patients enrolled consecutively from August 2018 who met the eligibility criteria. The study followed the tenets of the Declaration of Helsinki (1964), and written informed consent was obtained from each subject. The study protocol has been registered in the Clinical Research Information Service (CRIS) of the Korea Centers for Disease Control and Prevention (KCDC) (registration number: KCT0004980).
Study Subjects
For inclusion in the present study, subjects had to have myopia with refraction < –0.5 D or axial length (AXL) > 24 mm. High myopia was defined as refraction < –6.0 D or AXL > 26 mm. POAG was defined as including the presence of glaucomatous optic disc changes such as focal notching and thinning, RNFL defects on disc stereo photography and red-free fundus photography, glaucomatous visual field (VF) defect, and an open angle confirmed by gonioscopic examination. Glaucomatous VF defect was defined as (1) glaucoma hemifield test values outside the normal limits or (2) three or more abnormal points with a probability of being normal of P < 5%, of which at least one point has a pattern deviation of P < 1%, or (3) a pattern standard deviation of P < 5%. The VF defects were confirmed on two consecutive reliable tests (fixation loss rate ≤ 20%, false-positive and false-negative error rates ≤ 25%). Subjects were deemed to be healthy when no definite RNFL defect was found on red-free RNFL photography and they were found to be within normal limits on a glaucoma hemifield test of the standard automated perimetry.
The subjects initially underwent complete ophthalmic examinations including a medical history review, best-corrected visual acuity (BCVA), phakic status, refraction, slit-lamp biomicroscopy, Goldmann applanation tonometry, gonioscopy, dilated fundus examination, stereo optic-disc photography, red-free RNFL photography (VISUCAM, Carl Zeiss Meditec), and standard automated perimetry (HVF, Humphrey C 24-2 SITA-Standard visual field; Carl Zeiss Meditec). Also, the central corneal thickness (CCT) (Pocket II; Quantel Medical, Clermont-Ferrand, France) and AXL (AXIS-II Ultrasonic Biometer; Quantel Medical S.A., Bozeman, MT, USA) were measured.
The present study excluded subjects with a history of any ocular surgery such as glaucoma surgery, or a history of any retinal disease (e.g., diabetic retinopathy, uveitis, retinal vein occlusion, epiretinal membrane, or age-related macular degeneration). Eyes with ambiguous RNFL on red-free RNFL photography also were excluded. If both eyes were eligible for the present study, one eye was selected randomly.
SD-OCT and SS-OCT Imaging
The subjects underwent SD-OCT (Cirrus HD-OCT, Carl-Zeiss Meditec) and SS-OCT (DRI OCT Triton, Topcon) imaging in random order on the same day. All eyes were dilated (1% tropicamide, 2.5% phenylephrine) before undergoing OCT imaging. For Cirrus HD-OCT, a 200×200 macular cube scan and a 200×200 optic disc cube scan were obtained. The following macular GCIPL and peripapillary RNFL thicknesses were measured automatically by the built-in software (Cirrus HD-OCT Review Software, Ver. 10.0): average and sectoral (superotemporal, superior, superonasal, inferonasal, inferior, inferotemporal) measurements for GCIPL and average, sectoral (superior, nasal, inferior, and temporal) and 12 clock-hour measurements for RNFL thickness. For DRI OCT Triton, a 12×9 mm 3D Wide scan were obtained. The 3D Wide scan in DRI OCT Triton provides a 12×9 mm wide-field thickness map, a thickness surface map, and SuperPixel maps for the RNFL, GCL++ and GCL+ layers. The thickness surface map is displayed in 3D for selected layers (RNFL, GCL++, or GCL+) and can be rotated or zoomed-in and -out by mouse operation ( Figure 1 ). The SuperPixel-200 map consists of 26×26 grids within a 5.2×5.2 mm 2 peripapillary area and 30×30 grids within a 6.0×6.0 mm 2 macular area, thereby providing a significance map based on the built-in normative database. The side length of each grid is 200 μm. The uncolored pixels indicate the normal range, whereas the yellow-colored or red-colored pixels indicate abnormality at P = 1%-5% and P < 1% of the normal level, respectively. The average, sectoral (superior, nasal, inferior, and temporal), and 12 clock-hour peripapillary RNFL thicknesses and average and sectoral (superotemporal, superior, superonasal, inferonasal, inferior, inferotemporal) GCL++ and GCL+ thicknesses were measured automatically by the built-in review program (IMAGEnet 6 Version 1.25, Topcon). All of the analyses were performed according to the right-eye orientation.

Additionally excluded from the present study were eyes with motion or blink artifacts ( n = 14), defocus ( n = 12), algorithm segmentation failure ( n = 12), a signal strength < 6 ( n = 16) or image quality < 30 ( n = 3) on OCT scans, or any abnormalities (e.g., large peripapillary atrophy in the circumpapillary region that affected the scan circle where the OCT RNFL thickness measurement was obtained) ( n = 4).
Definition of Glaucomatous Structural Defect
Detailed definitions of RNFL defect on red-free photography, Cirrus HD-OCT, and DRI OCT Triton have been provided elsewhere. , RNFL defects for red-free RNFL photography have been defined as diverging, arched or wedge-shaped, wider than the main retinal vessel at a distance of 1-disc diameter from the edge of the disc.
RNFL defect on the Cirrus HD-OCT thickness map was defined as a wedge-shaped dark-blue area surrounding an abrupt color-scale change. RNFL defect on the deviation map was defined as the presence of a wedge-shaped area of at least 20 contiguous yellow/red pixels along with RNFL thinning. On both maps, the width of the defect increased from the disc toward the edge of the map, and the minimum defect size was larger than the diameter of the major retinal vessel. GCIPL defects on the Cirrus HD-OCT thickness map were defined as the presence of a blue/black region. For the deviation map, it was defined as the presence of an area coded in yellow/red, the size of which is greater than 10 pixels.
Defect on the DRI OCT Triton wide-field thickness map was defined as an arcuate or wedge-shaped diverging dark-blue (for RNFL and GCL+) or light-blue (for GCL++) area surrounding an abrupt color-scale change. The minimum defect size was larger than the diameter of a major retinal vessel. The wide-field thickness surface map was used to cross-check the three-dimensional (3D) thickness difference with the surrounding tissues. Defect on the SuperPixel-200 map was defined as the presence of a wedge-shaped area of at least 20 contiguous yellow/red pixels.
When RNFL defect on the red-free RNFL photography was not subsequently identified on the OCT maps, it was classified as a false-negative. If a defect on the OCT map did not match a defect on the red-free RNFL photography and/or HVF test results, it was classified as a false-positive. The inter-rater agreement for assessment of glaucomatous structural defect was obtained from three glaucoma specialists (YWK, JHL, and JSK) by estimation of Fleiss’ kappa. If the readings did not match each other, they were determined by consensus of the three readers.
Data Analysis
Continuous variables were compared between the two groups by student t-test. Categorical variables were compared using a chi-square test. The accuracy for glaucomatous structural defect was measured according to the following equation: Accuracy = (true-positive + true-negative) / (true-positive + false-positive + true-negative + false-negative). McNemar’s test was used to compare the sensitivity and specificity for detection of glaucomatous structural defect between the two OCT devices. The diagnostic performance of each parameter from the two OCT devices for the detection of myopic glaucoma was determined by calculating the area under receiver operating characteristic (AUROC) curve. The best cut-off value was selected according to the Youden index value (which maximizes the value of ‘sensitivity + specificity – 1’). A receiver operating characteristic (ROC) analysis was performed using the “pROC” package in the open-platform R software. The AUCs were compared using the “ROCtest” function in the “pROC” package, based on the method introduced by DeLong et al. The false discovery rate (FDR) was controlled for using the Benjamini-Hochberg method. Except where stated otherwise, the data are presented as mean ± standard deviations, and the level of statistical significance was set at P < 0.05.
Results
Subject Demographics
The present study enrolled 150 myopic glaucoma eyes and 100 healthy myopic eyes. The average age was 54.8 ± 12.8 years for myopic glaucoma and 51.8 ± 13.4 years for healthy myopic eyes (the controls), respectively ( P = 0.11). There were no significant differences in age, gender, BCVA, phakic status, IOP, SE, disc area, AXL or CCT between the two groups ( Table 1 ). The range of disc area was 1.03–3.55 mm 2 for myopic glaucoma and 1.04–3.22 mm 2 for healthy myopia. There was no difference in the microdisc proportion (disc area < 1.63 mm 2 ) between myopic glaucoma (n = 49, 32.7%) and healthy myopia (n = 27, 27%) ( P = 0.42). The mean deviation (MD) of VF was –4.23 ± 5.68 dB for myopic glaucoma and 0.23 ± 1.83 dB for the healthy controls ( P < 0.001). Among the myopic glaucoma eyes, 39 (26.0%) had RNFL defect in the inferotemporal region, 31 (20.7%) in the superotemporal region, and 80 (53.3%) in both the inferotemporal and superotemporal regions. The detailed demographic data are provided in Table 1 .
Myopic POAG (n = 150) | Healthy Myopia (n = 100) | P -value | |
---|---|---|---|
Age, yr | 54.8 ± 12.8 | 51.8 ± 13.4 | 0.11 a |
Gender, female, n (%) | 65 (43.3) | 52 (52.0) | 0.27 b |
BCVA, logMAR | 0.04 ± 0.12 | 0.02 ± 0.12 | 0.37 |
Phakia, n (%) | 139 (92.7) | 95 (95.0) | |
IOP, mmHg | 13.3 ± 2.7 | 13.6 ± 2.6 | 0.44 a |
SE, D | –3.78 ± 3.07 | –3.14 ± 2.66 | 0.13 a |
AXL, mm | 25.26 ± 1.17 | 24.89 ± 1.21 | 0.09 a |
High myopia, n (%) | 46 (30.7) | 21 (21.0) | 0.12 b |
CCT, μm | 546.6 ± 36.6 | 544.5 ± 37.2 | 0.68 a |
RNFL thickness, μm | 73.5 ± 10.8 | 87.3 ± 9.6 | <0.001 a |
Rim area, mm 2 | 0.84 ± 0.23 | 1.12 ± 0.23 | <0.001 a |
Disc area, mm 2 | 1.89 ± 0.51 | 1.91 ± 0.41 | 0.68 a |
Average C/D | 0.72 ± 0.12 | 0.61 ± 0.15 | <0.001 a |
Vertical C/D | 0.73 ± 0.12 | 0.57 ± 0.15 | <0.001 a |
Cup volume, mm 3 | 0.425 ± 0.281 | 0.284 ± 0.222 | <0.001 a |
Average GCIPL thickness, μm | 69.6 ± 8.4 | 79.9 ± 6.3 | <0.001 a |
Minimum GCIPL thickness, μm | 59.9 ± 11.7 | 75.3 ± 11.4 | <0.001 a |
MD. dB | –4.23 ± 5.68 | 0.23 ± 1.83 | <0.001 a |
PSD, dB | 5.94 ± 4.52 | 1.84 ± 1.03 | <0.001 a |
VFI, % | 87.1 ± 16.5 | 98.9 ± 2.2 | <0.001 a |
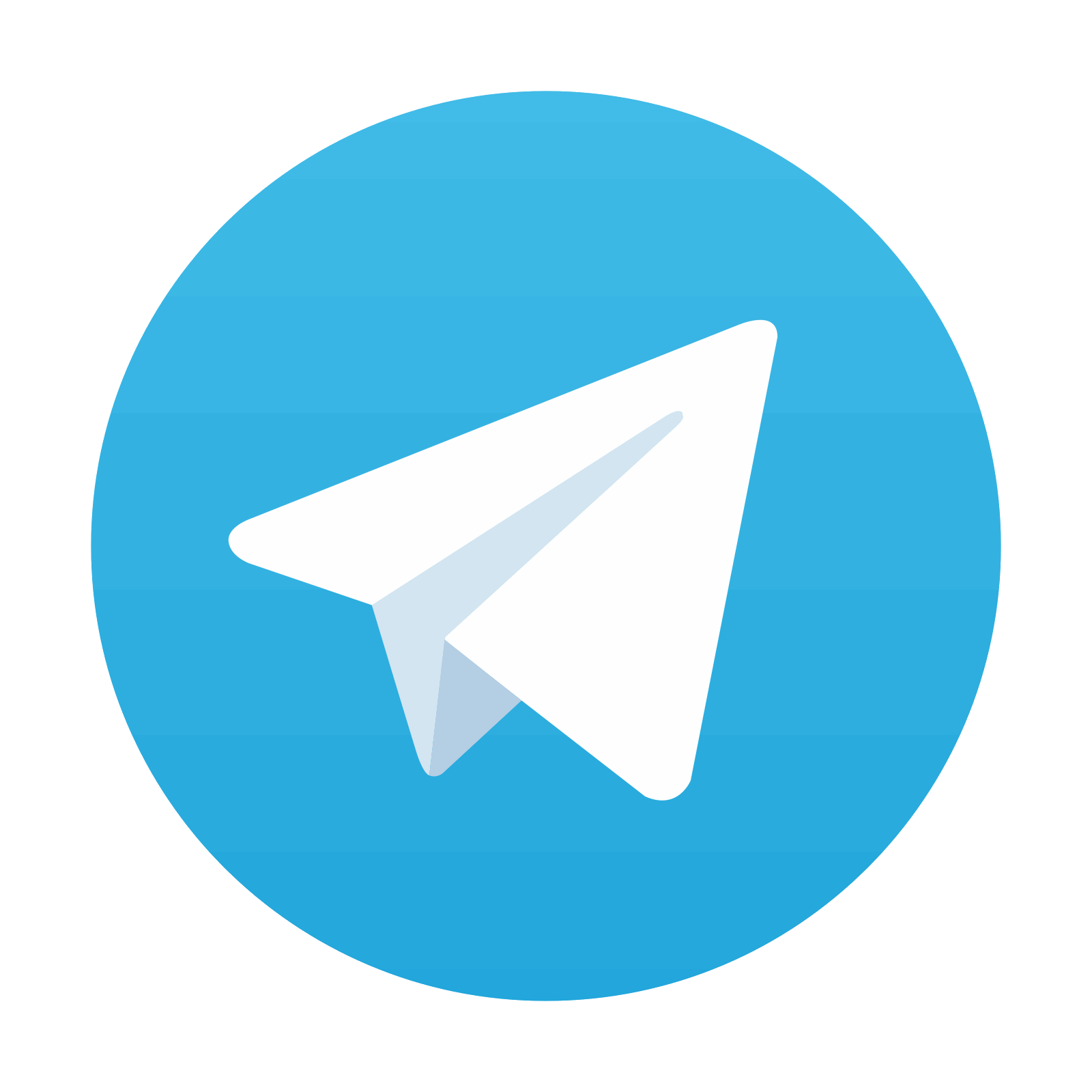
Stay updated, free articles. Join our Telegram channel

Full access? Get Clinical Tree
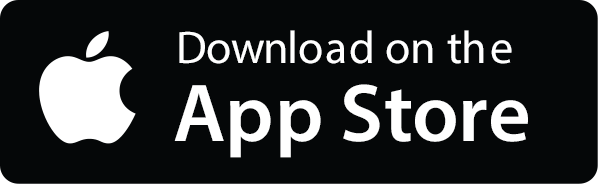
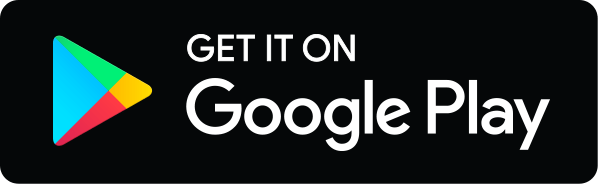
