Key Features
- •
Spectacle lens advantages: inexpensive; suitable to correct myopia, hyperopia, astigmatic refractive errors, and presbyopia; no permanent ocular alterations. Drawbacks: some patients may reject for cosmetic reasons; may cause image jump, object displacement, and alter retinal image size.
- •
Contact lens advantages: excellent cosmetic appearance, free from prismatic effects, minimize astigmatic distortions and problems with alterations in retinal image size. Drawbacks: correction of astigmatic errors and presbyopia may be difficult; expose patients to risk of corneal oxygen deprivation and corneal infections.
- •
Orthokeratology advantages: patients may be able to function without glasses or contact lenses during daytime. Drawbacks: require nighttime contact lens wear; correction may wane during daytime hours.
- •
Intraocular lens implant advantages: preferred correction for aphakia; may allow for correction of extreme myopia in phakic patients. Drawbacks: limited correction of presbyopia, astigmatism; phakic intraocular lenses may induce cataract formation.
- •
Keratorefractive surgery advantages: may eliminate need for glasses. Drawbacks: less predictable for correction of larger refractive errors; may destabilize cornea; risk of complications (small).
Introduction
In an ideal eye, the combined optical contribution of the front of the eye focuses incoming rays of light onto the retina to produce a clear image. If this optically ideal eye requires no aid or accommodation to focus distant objects onto the retina, the eye is said to be emmetropic. Conversely, if an eye in its relaxed, nonaccommodating state and devoid of other pathology fails to produce a clear image on the retina from rays of light of a distant object, the eye is said to be ametropic. Ametropia encompasses a wide range of optical imperfections including myopia (nearsightedness or shortsightedness), hyperopia (farsightedness or long-sightedness), and astigmatism, itself a collection of different optical imperfections. Indeed, an almost limitless number of optical abnormalities exist beyond these, but such optical aberrations are not amenable to correction by spectacles, and discussion of these will be limited in this chapter.
In addition to the very obvious disadvantage of reducing visual clarity, ametropia may be an indicator of more serious ophthalmic disease. An adult whose previously stable refraction has begun to demonstrate increasing myopia may be demonstrating the refractive effect of a maturing nuclear cataract. A 20-year-old whose astigmatism has begun to worsen may be suffering from progressive keratoconus, a disease of ectasia of the most refractively important part of the eye, the cornea. The highly hyperopic middle-aged woman is at substantial risk of angle-closure glaucoma for precisely the same reason for which she is hyperopic, a small and therefore crowded eye.
A number of clinical modalities exist to correct ametropia. Each of these has limitations, and different types of correction are advantageous for different clinical settings. The simplest of these and the only method without medical risk is spectacle correction.
Spectacle Correction
Evidence of spectacle use dates to at least the late thirteenth century. Although this certainly implies that people with refractive errors could seek no remedy for the countless millennia that preceded the invention of spectacles, it has been suggested that ametropia, particularly myopia, was far less common in the ancient world than it is at present.
Spectacle Material
Whether a person wears glasses for correction of refractive error, protection of eyes, or for cosmetic reasons, the materials of the lenses can play a significant role. For the patient who desires the best optical quality throughout her or his visual field, a thin lens with significant peripheral blurring might be a poor choice. Likewise, for the patient for whom impact protection is paramount, a glass lens that can easily break and shatter would be unsuitable.
Spectacle lenses are manufactured from a variety of materials with characteristics that can be condensed into a few properties that describe the optical quality, strength, and density. The ideal material would be thin, lightweight, impact and scratch resistant, inexpensive, and devoid of optical distortion. The variety of available materials may be seen as a demonstration that no single optimal material exists. One unwanted property of lens material is the tendency to disperse white light into its component wavelengths. Rainbows are pretty in the sky but the color fringing that results from wavelength dispersion in spectacles is distracting and degrades image quality. This wavelength dispersion is referred to as chromatic aberration, and the degree to which materials disperse wavelengths is quantified as the Abbe number or V-number. Materials that minimize chromatic dispersion—those with higher Abbe numbers—are generally more desirable.
Of course, the primary reason for which lenses are designed is to bend or refract light. The degree to which a spectacle lens bends light is a function of its curvature and of the material from which it is made. For any given shape, certain materials will bend light more than others. The degree to which a material can bend or refract light is a function of the speed of light through that material. We generally think of the speed of light as the immutable value c or 299,792,458 m/s, but of course this is the speed of light through a vacuum. Through any other medium, light propagates at a velocity lower than c. Media through which light moves especially slowly have the greatest ability to refract. The degree to which a material is capable of refracting light is given by the ratio of c over the speed of light through the material. This ratio is called the index of refraction, and higher values indicate a greater ability of a material to refract light. Because a lens with greater intrinsic ability to refract light requires a less curved surface to achieve the desired refraction, high refractive index lenses can be made thinner than low refractive index lenses. For this reason, patients with high degrees of ametropia often opt for high index lenses.
Spectacles also serve as a barrier between the environment and the eyes. For some patients—those who are monocular or amblyopic and those who are engaged in work in which ocular trauma is possible—the strength of the lens material may be its most important property. Standards are set by the US Food and Drug Administration (FDA) and American National Standards Institute (ANSI) for resistance of a material to penetration or shattering. Although glass lenses offer excellent optical quality and scratch resistance at a low cost, glass has fallen out of favor because of its poor impact resistance and high specific gravity, making it a heavy material. Plastic, also known as hard resin or Columbia resin (CR-39), has become the most common material for spectacles. It is light, impact resistant, and versatile, naturally UV blocking, and can be easily tinted or treated to be scratch resistant. However, plastic has a low index of refraction and a thicker lens is therefore required. A stronger material, polycarbonate, is commonly used in protective wear. Originally made for aerospace and used in helmet visors and space shuttle windshields, this petroleum derivative plastic polymer has a high refractive index and low specific gravity with very high impact resistance. Although these lenses are thin and lightweight, polycarbonate has a relatively low Abbe number, and so high chromatic dispersion results. Polycarbonate is also prone to scratches, making scratch-resistant coating essential.
For high refractive errors, high refractive index materials can be beneficial, although not without costs—both optical and financial. Plastic or glass materials that are made with higher index of refraction (i.e., over 1.6) are lighter and thinner but are also prone to more chromatic aberration and distortion at the periphery of the lens. They also tend to be less impact and scratch resistant.
Monofocal Spectacles
Monofocal spectacles correct for spherical refractive errors such as myopia and hyperopia, astigmatic refractive errors, and for combinations of spherical and astigmatic errors. For patients incapable of any degree of accommodation, for example patients who have undergone cataract extraction, these spectacles bring clear focus to objects at only a defined distance from the eye. For example, monofocal distance spectacles would not bring near objects into clear focus for such a patient. In clinical practice, monofocal lenses are prescribed to young, nonpresbyopic patients for all tasks and for presbyopic patients for limited tasks. For emmetropic presbyopic patients, those with naturally good distance vision but for whom accommodation is inadequate, monofocal reading glasses often are prescribed.
Clinicians prescribing monofocal spectacles specify only three parameters for each eye: the power of the spherical correction, the power of the astigmatic correction, and the orientation (axis) of the astigmatic correction. Although this prescription may compensate for the patient’s refractive error, a number of factors other than these determine whether the patient will tolerate the prescribed lenses. These factors have in common the drawback that image distortion is intrinsic to spectacle correction. Most important, distortions arise from asymmetrical image magnification and may be illustrated in this thought experiment: Imagine an emmetropic patient looking through a telescope at a distant object. The telescope, a Galilean telescope in this case, consists of a plus-power or converging objective (the lens at the large end of the telescope) and a minus-power or diverging eyepiece. For this experiment, let’s imagine that the minus-power eyepiece is a contact lens. Indeed, the telescope would exhibit the same degree of magnification if the eyepiece were a conventional lens or a contact lens. If we further imagine that our patient, rather than being emmetropic, has instead a refractive error equivalent to the contact-lens eyepiece, she or he would experience the same degree of magnification as our original emmetrope. Our ametropic patient is hyperopic. We know this because the correcting lens (i.e., the objective of the telescope) is plus powered and thus we see that hyperopes corrected with plus spectacles experience a degree of image magnification. The case of the myope is identical in every regard except that the “telescope” is now oriented backward and produces object minification.
The magnification produced by spectacles is generally tolerated well by patients as long as its magnitude is similar in each eye. However, asymmetrical magnification of only a few percent can produce symptoms sufficient to make spectacle correction intolerable. The degree of magnification is a function of two variables: the dioptric power of the spectacle lens (equivalent to the telescope’s object lens) and the distance between the spectacle lens and the eye, known as the vertex distance (and equivalent to the length of the telescope’s tube).
The condition of perceived difference in image size between right and left eyes is called aniseikonia. As a general rule, adults who are unaccustomed to large differences in prescriptions between right and left eyes will tolerate no more than 3 or 4 diopters of asymmetry in spectacles. This challenge arises clinically in the case of patients who are developing an asymmetrical increase in myopia from a maturing nuclear cataract (so-called myopic shift) or after cataract surgery if the refractive errors are substantially asymmetrical postoperatively.
Symptoms attributable to asymmetrical magnification are not limited to aniseikonia from asymmetrical spectacles. Asymmetrical magnification also can produce symptoms monocularly. Because the degree of magnification produced by a spectacle lens is dependent partly on the distance between the lens and the eye, the degree of magnification or minification is least at the center of the spectacle lens where the lens is closest to the cornea and increases farther away from the center of the spectacle lens. This produces a type of distortion characteristic to each type of lens.
Spectacles to correct myopia employ minus-powered lenses. These lenses produce minification and, because the periphery of the image is minified to a greater extent, produce a sort of distortion in which the periphery of the image is rendered at a smaller scale than the center. This is referred to as “barrel distortion.” Such distortion is readily observed when looking into a convenience store mirror, when looking at one’s self in a mirrored sphere like a ball bearing, or viewing a scene through a fisheye lens.
Similarly, plus-powered spectacles for the correction of hyperopia produce distorted images in which the periphery is more magnified than the center. This “pin-cushion distortion” also may be observed when using a magnifying glass ( Fig. 2.4.1 ).

Perhaps the most disturbing sort of asymmetrical magnification induced by spectacle lenses is the meridional magnification intrinsic to astigmatic lenses. In this case the meridian of highest plus power produces relative magnification compared with the meridian of highest minus power. The net result is to produce images that are short and fat, tall and skinny, or magnified obliquely. This is especially disturbing when, as is often the case, astigmatic axes are oblique and at mirror-image orientations in the two eyes. Oppositely oriented meridional magnification may be misinterpreted as distortion in depth perception and can make spectacles intolerable even if the right and left lenses are of equal dioptric power.
Bifocals
Traditional bifocal and trifocal lenses have been largely displaced by progressive lenses. Still, these older forms remain in wide use and in some clinical settings have advantages over the newer progressive designs. Bifocal lenses are distinguished by discrete segments for distance and for near with an abrupt transition between the two ( Fig. 2.4.2 ). Unlike monofocal lenses, the prescriptions for which are ground into the back surface of the lens, bifocal segments are applied to the front surface. Bifocal designs take a number of forms, and the appropriate choice of bifocal segment is a function of the lens to which it is applied.

All bifocal segments are plus-dioptric powers. As such, they may be thought of as portions of a larger plus-powered lens. The round-type bifocal would then derive from the top of a plus-powered lens and the flat-top from the bottom of a plus-powered lens. This is highly significant because each type of bifocal adds a certain degree of prism to the bottom of the spectacles and produces a certain amount of discontinuity at the line of transition between the distance and near portions of the lens. The discontinuity of the image produced at the line between the distance and the near portion of the lens is called “image jump.” The degree of image jump is a function of the power of the reading add and of the distance between the optical center of the reading add and the top of the reading segment. By Prentice rule, discussed earlier in this volume, the amount of prism induced at the top of the bifocal segment is proportional to the distance between the optical center of the segment and the top of the segment. Round-top bifocals have the largest distance between these two points and therefore will produce the largest image jump for any given bifocal power. Traditional flat-top bifocals have only a small distance between the optical center and the top of the bifocal segment and produce only a very small amount of image jump.
Although image jump may be annoying to patients, it is not the chief determinant in choosing the appropriate bifocal segment design. Rather, the bifocal design is chosen in an effort to minimize the total amount of prismatic power induced at the bottom of the patient’s spectacle. All spectacles, even those without bifocal segments, demonstrate prismatic power for all locations away from the optical center. The amount of prismatic power at the bottom of the spectacle lens is important because the shift in the image induced by this prism may cause patients to miss obstacles on the ground or misjudge the location of curbs or steps. It is advantageous to choose a bifocal design that will incorporate a prism of opposite orientation to that already existing in the patient’s spectacles. In the case of hyperopic patients who are wearing spectacles that already incorporate a base up prism in the bottom portion of the lens, the appropriate bifocal choice would be one that demonstrates a base down prism ( Figs. 2.4.3 and 2.4.4 ). This is necessarily a round-top bifocal segment. Such a choice will minimize the total prismatic power at the bottom of the patient’s spectacles and make it less likely that the patient will miss obstacles in his path. Keep in mind that this is the appropriate choice despite the fact that round-top bifocals produce more substantial image jump than flat-top type bifocals.


In the case of the myope who demonstrates a base down prism in the bottom portion of her or his spectacle lens, a base up bifocal design will prove complementary, and therefore a flat-top bifocal is the appropriate choice for this patient ( Figs. 2.4.5 and 2.4.6 ).


Currently, choices exist beyond simple round-top and flat-top designs. Some flat-top shaped bifocals known as C-designs and D-designs actually extend above the optical centers of the reading segment. This results in only a small amount of induced prism over much of the area of the bifocal segment. Therefore this may be an acceptable design even in the case of hyperopic patients. It must be remembered that although this design does not make the image displacement produced by the total prism materially worse, the choice of this type of bifocal represents a missed opportunity to make the image displacement better.
Progressive Spectacles
Progressive spectacles or progressive-addition lenses (PAL) hold a number of advantages over traditional bifocal and trifocal lenses. PAL spectacles appeal to patients because they are without visible lines and so do not reveal that the patient is of presbyopic age. The lenses have several functional advantages as well. Because no discrete interface exists between the distance and near portions, no image jump occurs. Image displacement is not an independent variable in this type of lens design, and so no choices need be made by the prescribing physician to minimize image displacement either. Progressive lenses incorporate variable dioptric power between the distance portion in primary and upgaze and the reading portion at the bottom of the lens. Because the lens powers are graduated, there is at least some portion of the spectacle lens that will bring objects at intermediate distance into focus.
Progressive lenses vary substantially in design, but all share a number of features. From primary gaze through the center of the lens to the top of the frame, progressive lenses provide a single correction for distance vision. The bottom of the lens contains a region of single power for reading. These two regions are connected in a narrow-waist corridor. The regions to the right and left of the corridor (looking down and to either side) do not provide clear focus at any distance because of the aberrations necessary to produce the graduated power along the centerline of the lens. These afocal areas are called blend zones and are the source of complaints from patients who find it difficult to adapt to progressive lenses ( Fig. 2.4.7 ).

The width of the corridor and the amount of the lens devoted to intermediate vision vary by the particular lens design and manufacturer. Because sufficient room in the spectacle frame must exist beneath primary gaze for the graduation to incorporate an adequate reading portion, some frames are unacceptably short for the incorporation of a progressive lens.
Occupational Bifocals
Before the advent of desktop computers, bifocal spectacles were probably adequate to most patients’ needs. The distinguishing feature of desktop computers is that their screens represent an intermediate distance task that is performed in primary (i.e., straight-ahead) gaze or slightly below primary gaze. Because it is impossible to prescribe spectacles that have two different prescriptions in primary gaze, dedicated computer glasses are often warranted. Progressive spectacles may provide a useful solution if the computer screen can be placed so as to intercept the zone of intermediate-add correction. If the screen is placed too high for the patient to view the computer screen, he must adopt a chin-up position. This is inadvisable for lengthy computer use.
Another solution is to prescribe either single-vision spectacles for the computer distance—often 21 or 22 inches from the patient’s eyes—or to prescribe glasses that are indeed bifocal but incorporate only the computer distance and reading distance without having any portion dedicated to far distance vision. These are called occupational bifocals and allow the patient to see the computer screen in primary gaze and to bring printed copy to the closer reading position in downgaze.
Pantoscopic Tilt and Wrap Angle
In the course of normal activities, people tend to employ either primary gaze or downgaze. Few of our tasks require substantial upgaze. If spectacle lenses were oriented in the horizontal or coronal plane, the spectacle lens would be farther from the eye in downgaze than in primary gaze. To compensate for this, spectacles incorporate a tilt to the lens about the horizontal axis. This pantoscopic tilt keeps the vertex distance (the distance between the cornea and the lens) more consistent in primary and downgaze. An additional benefit is that the patient gains a larger field of vision in the reading portion of the lens because it is closer to the eye. A similar tilt may be incorporated along the vertical axis. This is referred to as Z-tilt or as the wrap angle of the lens.
Base Curve and Center Thickness
A number of other variables influence the degree of magnification produced by spectacle lenses. Because myopic spectacles induce a degree of minification, parameters such as base curve (or back curve) and center lens thickness may be altered to reduce this minification. Increasing the convexity of the back surface of the lens and increasing the thickness of the center of the lens will produce a small degree of magnification. In practice, these may be difficult to implement because increasing the thickness of the already thick lens of a high myope will make the spectacle heavy and less attractive and because increasing the back curve tends to increase the vertex distance of the lens.
Spectacle Material
Whether a person wears glasses for correction of refractive error, protection of eyes, or for cosmetic reasons, the materials of the lenses can play a significant role. For the patient who desires the best optical quality throughout her or his visual field, a thin lens with significant peripheral blurring might be a poor choice. Likewise, for the patient for whom impact protection is paramount, a glass lens that can easily break and shatter would be unsuitable.
Spectacle lenses are manufactured from a variety of materials with characteristics that can be condensed into a few properties that describe the optical quality, strength, and density. The ideal material would be thin, lightweight, impact and scratch resistant, inexpensive, and devoid of optical distortion. The variety of available materials may be seen as a demonstration that no single optimal material exists. One unwanted property of lens material is the tendency to disperse white light into its component wavelengths. Rainbows are pretty in the sky but the color fringing that results from wavelength dispersion in spectacles is distracting and degrades image quality. This wavelength dispersion is referred to as chromatic aberration, and the degree to which materials disperse wavelengths is quantified as the Abbe number or V-number. Materials that minimize chromatic dispersion—those with higher Abbe numbers—are generally more desirable.
Of course, the primary reason for which lenses are designed is to bend or refract light. The degree to which a spectacle lens bends light is a function of its curvature and of the material from which it is made. For any given shape, certain materials will bend light more than others. The degree to which a material can bend or refract light is a function of the speed of light through that material. We generally think of the speed of light as the immutable value c or 299,792,458 m/s, but of course this is the speed of light through a vacuum. Through any other medium, light propagates at a velocity lower than c. Media through which light moves especially slowly have the greatest ability to refract. The degree to which a material is capable of refracting light is given by the ratio of c over the speed of light through the material. This ratio is called the index of refraction, and higher values indicate a greater ability of a material to refract light. Because a lens with greater intrinsic ability to refract light requires a less curved surface to achieve the desired refraction, high refractive index lenses can be made thinner than low refractive index lenses. For this reason, patients with high degrees of ametropia often opt for high index lenses.
Spectacles also serve as a barrier between the environment and the eyes. For some patients—those who are monocular or amblyopic and those who are engaged in work in which ocular trauma is possible—the strength of the lens material may be its most important property. Standards are set by the US Food and Drug Administration (FDA) and American National Standards Institute (ANSI) for resistance of a material to penetration or shattering. Although glass lenses offer excellent optical quality and scratch resistance at a low cost, glass has fallen out of favor because of its poor impact resistance and high specific gravity, making it a heavy material. Plastic, also known as hard resin or Columbia resin (CR-39), has become the most common material for spectacles. It is light, impact resistant, and versatile, naturally UV blocking, and can be easily tinted or treated to be scratch resistant. However, plastic has a low index of refraction and a thicker lens is therefore required. A stronger material, polycarbonate, is commonly used in protective wear. Originally made for aerospace and used in helmet visors and space shuttle windshields, this petroleum derivative plastic polymer has a high refractive index and low specific gravity with very high impact resistance. Although these lenses are thin and lightweight, polycarbonate has a relatively low Abbe number, and so high chromatic dispersion results. Polycarbonate is also prone to scratches, making scratch-resistant coating essential.
For high refractive errors, high refractive index materials can be beneficial, although not without costs—both optical and financial. Plastic or glass materials that are made with higher index of refraction (i.e., over 1.6) are lighter and thinner but are also prone to more chromatic aberration and distortion at the periphery of the lens. They also tend to be less impact and scratch resistant.
Monofocal Spectacles
Monofocal spectacles correct for spherical refractive errors such as myopia and hyperopia, astigmatic refractive errors, and for combinations of spherical and astigmatic errors. For patients incapable of any degree of accommodation, for example patients who have undergone cataract extraction, these spectacles bring clear focus to objects at only a defined distance from the eye. For example, monofocal distance spectacles would not bring near objects into clear focus for such a patient. In clinical practice, monofocal lenses are prescribed to young, nonpresbyopic patients for all tasks and for presbyopic patients for limited tasks. For emmetropic presbyopic patients, those with naturally good distance vision but for whom accommodation is inadequate, monofocal reading glasses often are prescribed.
Clinicians prescribing monofocal spectacles specify only three parameters for each eye: the power of the spherical correction, the power of the astigmatic correction, and the orientation (axis) of the astigmatic correction. Although this prescription may compensate for the patient’s refractive error, a number of factors other than these determine whether the patient will tolerate the prescribed lenses. These factors have in common the drawback that image distortion is intrinsic to spectacle correction. Most important, distortions arise from asymmetrical image magnification and may be illustrated in this thought experiment: Imagine an emmetropic patient looking through a telescope at a distant object. The telescope, a Galilean telescope in this case, consists of a plus-power or converging objective (the lens at the large end of the telescope) and a minus-power or diverging eyepiece. For this experiment, let’s imagine that the minus-power eyepiece is a contact lens. Indeed, the telescope would exhibit the same degree of magnification if the eyepiece were a conventional lens or a contact lens. If we further imagine that our patient, rather than being emmetropic, has instead a refractive error equivalent to the contact-lens eyepiece, she or he would experience the same degree of magnification as our original emmetrope. Our ametropic patient is hyperopic. We know this because the correcting lens (i.e., the objective of the telescope) is plus powered and thus we see that hyperopes corrected with plus spectacles experience a degree of image magnification. The case of the myope is identical in every regard except that the “telescope” is now oriented backward and produces object minification.
The magnification produced by spectacles is generally tolerated well by patients as long as its magnitude is similar in each eye. However, asymmetrical magnification of only a few percent can produce symptoms sufficient to make spectacle correction intolerable. The degree of magnification is a function of two variables: the dioptric power of the spectacle lens (equivalent to the telescope’s object lens) and the distance between the spectacle lens and the eye, known as the vertex distance (and equivalent to the length of the telescope’s tube).
The condition of perceived difference in image size between right and left eyes is called aniseikonia. As a general rule, adults who are unaccustomed to large differences in prescriptions between right and left eyes will tolerate no more than 3 or 4 diopters of asymmetry in spectacles. This challenge arises clinically in the case of patients who are developing an asymmetrical increase in myopia from a maturing nuclear cataract (so-called myopic shift) or after cataract surgery if the refractive errors are substantially asymmetrical postoperatively.
Symptoms attributable to asymmetrical magnification are not limited to aniseikonia from asymmetrical spectacles. Asymmetrical magnification also can produce symptoms monocularly. Because the degree of magnification produced by a spectacle lens is dependent partly on the distance between the lens and the eye, the degree of magnification or minification is least at the center of the spectacle lens where the lens is closest to the cornea and increases farther away from the center of the spectacle lens. This produces a type of distortion characteristic to each type of lens.
Spectacles to correct myopia employ minus-powered lenses. These lenses produce minification and, because the periphery of the image is minified to a greater extent, produce a sort of distortion in which the periphery of the image is rendered at a smaller scale than the center. This is referred to as “barrel distortion.” Such distortion is readily observed when looking into a convenience store mirror, when looking at one’s self in a mirrored sphere like a ball bearing, or viewing a scene through a fisheye lens.
Similarly, plus-powered spectacles for the correction of hyperopia produce distorted images in which the periphery is more magnified than the center. This “pin-cushion distortion” also may be observed when using a magnifying glass ( Fig. 2.4.1 ).

Perhaps the most disturbing sort of asymmetrical magnification induced by spectacle lenses is the meridional magnification intrinsic to astigmatic lenses. In this case the meridian of highest plus power produces relative magnification compared with the meridian of highest minus power. The net result is to produce images that are short and fat, tall and skinny, or magnified obliquely. This is especially disturbing when, as is often the case, astigmatic axes are oblique and at mirror-image orientations in the two eyes. Oppositely oriented meridional magnification may be misinterpreted as distortion in depth perception and can make spectacles intolerable even if the right and left lenses are of equal dioptric power.
Bifocals
Traditional bifocal and trifocal lenses have been largely displaced by progressive lenses. Still, these older forms remain in wide use and in some clinical settings have advantages over the newer progressive designs. Bifocal lenses are distinguished by discrete segments for distance and for near with an abrupt transition between the two ( Fig. 2.4.2 ). Unlike monofocal lenses, the prescriptions for which are ground into the back surface of the lens, bifocal segments are applied to the front surface. Bifocal designs take a number of forms, and the appropriate choice of bifocal segment is a function of the lens to which it is applied.

All bifocal segments are plus-dioptric powers. As such, they may be thought of as portions of a larger plus-powered lens. The round-type bifocal would then derive from the top of a plus-powered lens and the flat-top from the bottom of a plus-powered lens. This is highly significant because each type of bifocal adds a certain degree of prism to the bottom of the spectacles and produces a certain amount of discontinuity at the line of transition between the distance and near portions of the lens. The discontinuity of the image produced at the line between the distance and the near portion of the lens is called “image jump.” The degree of image jump is a function of the power of the reading add and of the distance between the optical center of the reading add and the top of the reading segment. By Prentice rule, discussed earlier in this volume, the amount of prism induced at the top of the bifocal segment is proportional to the distance between the optical center of the segment and the top of the segment. Round-top bifocals have the largest distance between these two points and therefore will produce the largest image jump for any given bifocal power. Traditional flat-top bifocals have only a small distance between the optical center and the top of the bifocal segment and produce only a very small amount of image jump.
Although image jump may be annoying to patients, it is not the chief determinant in choosing the appropriate bifocal segment design. Rather, the bifocal design is chosen in an effort to minimize the total amount of prismatic power induced at the bottom of the patient’s spectacle. All spectacles, even those without bifocal segments, demonstrate prismatic power for all locations away from the optical center. The amount of prismatic power at the bottom of the spectacle lens is important because the shift in the image induced by this prism may cause patients to miss obstacles on the ground or misjudge the location of curbs or steps. It is advantageous to choose a bifocal design that will incorporate a prism of opposite orientation to that already existing in the patient’s spectacles. In the case of hyperopic patients who are wearing spectacles that already incorporate a base up prism in the bottom portion of the lens, the appropriate bifocal choice would be one that demonstrates a base down prism ( Figs. 2.4.3 and 2.4.4 ). This is necessarily a round-top bifocal segment. Such a choice will minimize the total prismatic power at the bottom of the patient’s spectacles and make it less likely that the patient will miss obstacles in his path. Keep in mind that this is the appropriate choice despite the fact that round-top bifocals produce more substantial image jump than flat-top type bifocals.

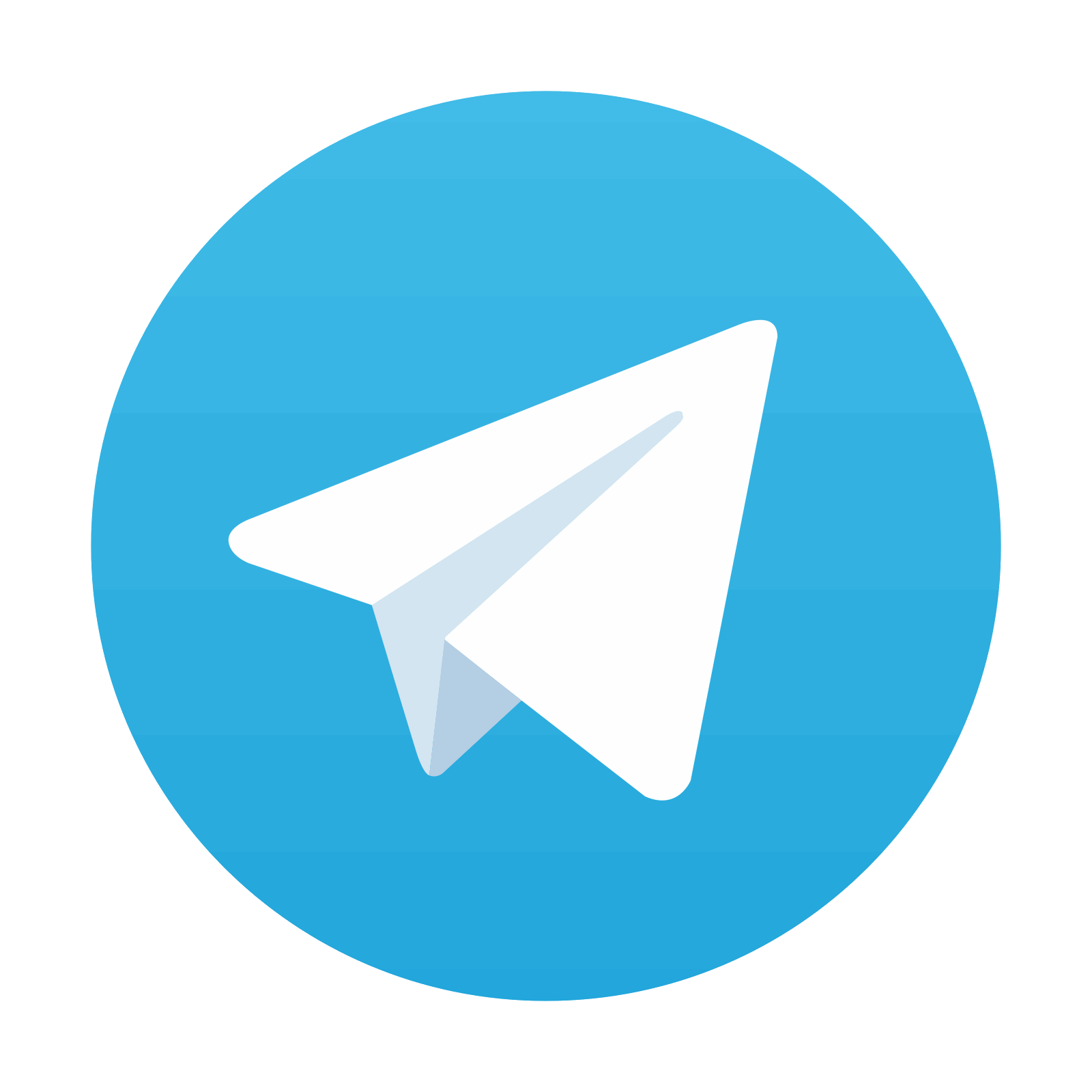
Stay updated, free articles. Join our Telegram channel

Full access? Get Clinical Tree
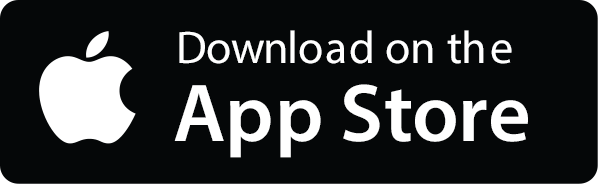
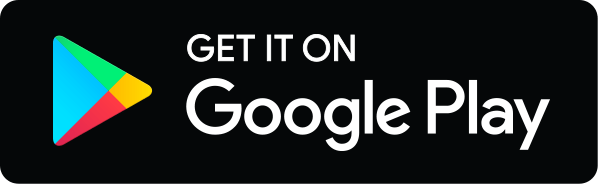
