Introduction
Refractive surgery procedures—including incisional radial keratotomy (RK), excimer laser photorefractive keratectomy (PRK), and laser in situ keratomileusis (LASIK)—are surgical procedures that cut, photoablate, or otherwise damage the cornea, resulting in a corneal wound healing response. In this chapter, we will review our understanding of the wound-healing response to keratorefractive surgery and discuss the possible cellular and molecular mechanism regulating this process.
Radial Keratotomy
Even though RK is rarely performed today, our understanding of the fundamental mechanism of corneal wound healing after keratorefractive surgery is anchored in the knowledge gained from basic experiments involving RK. RK is a nonpenetrating, incisional injury to the cornea that transects the corneal epithelium and extends into the corneal stroma. Healing after incisional injury therefore requires repair of both the corneal epithelium and the stroma. Initial injury results in a mild inflammatory response and edema around the incisional wounds that may persist for days or weeks after surgery. Within the first day after surgery, there is sliding of the corneal epithelium over the wound margins ( Fig. 2.1A ), followed by formation of an epithelial plug ( Fig. 2.1B ). Proliferating fibroblastic cells adjacent to the epithelial plug then migrate into the wound, replacing the epithelial plug over the next 2 weeks ( Fig. 2.1C ). Under transmission electron microscopy, fibroblastic cells contain dilated endoplasmic reticulum, suggesting the deposition of new connective tissue matrix that is later remodeled to form a linear scar 3 to 6 months after surgery ( Fig. 2.1D ).

Human pathologic tissue specimens have been examined by both light and electron microscopy, providing a varied picture of the wound-healing response compared to that observed in the nonhuman primate. Most striking has been the observation that the epithelial plug may persist in some incisions for 3 to 70 months following RK ( Fig. 2.2 ) or may be absent in other incision sites sampled at the same time points. The presence of an epithelial plug suggests delayed or aborted stromal wound repair that prevents reapposition of the wound margins and complete wound healing.

Wide variations in wound healing among different individuals has also been noted that may explain some of the variation in refractive outcomes in patients with similar baseline characteristics. Healing may also vary not only among individuals but also among individual incisions. Although some histopathologic studies have identified delayed stromal wound healing up to 70 months after surgery, complete wound healing leading to a fine linear scar is thought to be the normal stromal response.
Cellular Mechanism of Refractive Regression After Radial Keratotomy and Role of Corneal Myofibroblast
Decreasing wound gape after RK has been clearly associated with the development of wound fibrosis in the nonhuman primate. Wound fibrosis involves an initial activation and proliferation of stromal keratocytes that line the wound margin during the first days after injury. Fibroblastic cells then migrate into the wound, pushing out the epithelial plug, and form a hypercellular fibrotic mass within the wound margins. Fibroblasts within the wound deposit extracellular matrix, including fibronectin, collagen, and other matrix components that then become organized within the wound as the wound contracts ( Fig. 2.3A–C ). Detailed confocal microscopic studies of full-thickness incisional wounds in the rabbit have shown that cells migrate into the wound as an interconnected meshwork that establishes an interwoven cellular structure throughout the fibrotic tissue ( Fig. 2.4 ). Although fibroblasts are initially randomly organized, during wound contraction the fibroblasts become progressively aligned parallel to the wound margins as the wound contracts ( Fig. 2.4D ).


Studies also have shown that the reorientation of fibroblasts during wound contraction is associated with a change in the organization of contractile actin filament bundles or stress fibers contained within wound-healing fibroblasts ( Fig. 2.5 ). As shown earlier, deposition of extracellular matrix in the wound, particularly fibronectin, is associated with a marked increase in the assembly of filamentous actin (see Fig. 2.3D–F ). Three-dimensional analysis of actin filament assembly in corneal wounds of the rabbit also showed that, during the early phase of wound healing, the organization of actin filament structures is randomly arranged, similar to the orientation of cells ( Fig. 2.5A ). Bundles of actin filaments detected in one cell also appeared to extend through and were contiguous with actin filament bundles in adjacent cells, perhaps through specific cell–cell adherence junctional complexes, suggesting that cells were interconnected throughout the wound and the wound margin, forming a syncytium. As wounds heal and contract, intracellular actin filaments become progressively aligned parallel to the wound margins while at the same time aligning extracellular matrix through mechanical interactions mediated by specific cell–matrix adhesion sites formed to collagen and fibronectin by integral membrane receptors, that is, integrin a2β1 and a5β1, among others ( Fig. 2.5D ). Quantitative analysis of these changes also showed a significant correlation between the reorientation of actin filaments from random to aligned with measured changes in wound gape. This reorientation pattern suggests a unique cellular-based contractile mechanism involved in the generation of tension within the wound. Specifically, actin bundles generate force vectors that run parallel to the axis of the bundles and can be broken down into two vector components: one oriented across the wound and one oriented parallel to the wound. Based on vector analysis, contraction leads to twisting of actin bundles across the wound in response to differences in resistance. This process would result in closure of the wound and a more parallel orientation of the bundles along the long axis of the wound, comparable to tightening a shoelace.

This model of wound contraction implies an active, intracellular, muscle-like contractile mechanism. Darby et al. and Gabbiani originally described such a contractile mechanism for dermal wound-healing myofibroblasts, which have prominent microfilament bundles containing electron-dense structures similar to the ultrastructural organization of smooth muscle cells and express a smooth muscle–specific alpha-isoform of actin (α-SMA). Studies of corneal wound-healing fibroblasts have identified similar features to the dermal myofibroblast in that cells have prominent microfilament bundles with electron-dense structures and show in vitro contractile responses to smooth muscle agonists ( Fig. 2.6 ). Corneal wound-healing myofibroblasts also uniquely express smooth muscle–specific α-SMA and show a temporal appearance based on expression of α-SMA protein that coincides with the wound contractile response, that is, maximally expressed at the beginning of wound contraction (day 14) and disappearing by day 28 at the end of wound contraction ( Fig. 2.7 ).


Taken together, these studies indicate that, during the process of partial incisional wound healing after RK, adjacent corneal stromal cells become activated, proliferate, and differentiate to corneal myofibroblast-like cells. These cells invade the wound as an interconnected and interwoven syncytium that is randomly organized within the wound. During the invasion of the wound, cells synthesize and organize extracellular matrix, collagen types I and III, and fibronectin through integral membrane receptors by exerting force mediated through a smooth muscle–like contractile mechanism. Myofibroblast contraction then assists in both the invasion of the myofibroblast syncytium into the wound and the later contraction of the wound by applying mechanical force to the extracellular matrix and adjacent cells, leading to a reorientation of cells and matrix parallel to the long axis of the wound margins.
Excimer Laser Refractive Surgery
Trokel et al. were the first to demonstrate precise etching of the cornea using 193-nm ultraviolet radiation from the excimer laser. At the correct power, excimer radiation produces “ablative photodecomposition” of organic polypeptides by breaking molecular bonds and producing smaller volatile fragments through direct photochemical interaction without heating the adjacent tissue. Studies have shown that fluency thresholds above 40 mJ/cm 2 lead to photoablation of the corneal tissue, leaving adjacent tissue completely intact except for a 0.2-µm-thick, electron-dense, surface condensation referred to as a pseudomembrane ( Fig. 2.8 ).

Wound-Healing Response Following Excimer Laser Photorefractive Keratectomy
Corneal repair following PRK has been evaluated extensively using histopathologic and ultrastructural techniques in nonhuman primates, rabbits, and human pathologic tissue samples. These studies show that photoablation specifically damages (1) the central corneal epithelium and underlying epithelial basement membrane; (2) adjacent corneal keratocytes; and (3) the corneal stroma. They further show that photoablation results initially in the directed migration toward the wound of activated epithelial cells, fibroblasts, and macrophages, which participate in removal of damaged tissue, replacement of epithelial cells and keratocytes, and synthesis of new basement and stromal extracellular matrix. The major histopathologic findings following PRK are noted next.
Epithelium
Epithelial resurfacing is, for the most part, a rapid process requiring migration of the epithelium to resurface the cornea over the first 2 to 7 days, depending on the size ( Fig. 2.9 ). Reestablishment of the corneal epithelial surface, however, requires formation of both a new epithelial basement membrane and reformation of epithelial hemidesmosomes. Primate studies show attempts to re-form the epithelial basement membrane by 7 days after injury with focal areas of basement membrane distinguishable by transmission electron microscopy. Immunochemical detection of basement membrane matrix, collagen type IV and type VII, and laminin indicates their presence in the underlying stroma; yet, they do not appear to show a uniform distribution immediately below the corneal epithelium in the basement membrane zone. Long-term studies indicate that the basement membrane is abnormal and not completely re-formed by 18 months after surgery in primates, raising concern as to whether a normal basement membrane is ever reestablished. It is important to note that a failure to reestablish a normal epithelial basement membrane may contribute to epithelial breakdown, which may occur in as many as 3% of eyes after PRK.

Keratocyte Apoptosis
Histologic studies of primate corneas have shown that, immediately after surgery, there is a marked reduction in the number of keratocytes in the anterior 40 µm of the stroma, suggesting keratocyte cell death ( Fig. 2.10 ). Studies have shown that keratocytes undergo a process of programmed cell death, or apoptosis, following injury to the epithelium. Apoptosis is characterized by shrinkage of keratocytes, membrane blebbing with formation of membrane-bound bodies, condensation and fragmentation of the chromatin, and DNA fragmentation, which can be detected by terminal deoxynucleotidyl transferase dUTP nick end labeling (TUNEL). Apoptosis is thought to be mediated by release of cytokines released from damaged epithelium, particularly interleukin-1 alpha (IL-1α) or FAS ligand.

There are varying views regarding the importance of apoptosis to corneal wound healing following injury. As keratocyte apoptosis is one of the initial events to occur after epithelial injury, it is generally thought to play an important role in the downstream activation of the wound response. Some investigators suggest that activation of stromal cells and the development of stromal fibrosis may be initiated by keratocyte apoptosis and that effective management of corneal scarring may involve modulation of the apoptotic response. In support of this approach is the observation that LASIK surgery produces markedly less apoptosis than PRK and does not generally develop haze or wound fibrosis. Others suggest that apoptosis results in the programmed removal of dead keratocytes and results in substantially less inflammatory response than when keratocytes are allowed to undergo a necrotic process. As such, keratocyte apoptosis may actually decrease the development of haze and fibrosis, as seen following simple scrape injury, which does not lead to wound fibrosis.
Stromal Repair
Based on general studies of corneal wound healing, it is presumed that, after PRK, stromal cells become activated and then proliferate and migrate toward the wound during the first week after injury. At 7 days, there is an increase in the number of keratocytes adjacent to the wound; the number dramatically increases over the next 3 weeks. Cells within this region appear fibroblastic with extensive rough endoplasmic reticulum, indicating active synthesis of new extracellular matrix ( Fig. 2.11 ). This region also exhibits extensive vacuolation adjacent to the epithelium and within the anterior stroma. The origin of the vacuoles, whether extracellular or intracellular, is not clear, nor is the relevance to wound healing. Interestingly, it is at this phase of the wound-healing response that the greatest degree of clinically observable corneal haze or clouding is detected in primates, suggesting that haze may be associated with either the dramatic increase in the number of fibroblastic cells or the deposition of new extracellular matrix.

Over time, there is a decrease in the number of fibroblasts within the wound area. This progresses toward normal by 9 months, although the number of fibroblasts remains slightly increased in number and activity up to 18 months. The amount of new extracellular matrix or fibrotic tissue deposited during wound healing has been evaluated in rabbit corneas by staining the wound bed immediately after surgery with the fluorescent compound dichlorotriazinyl aminofluorescein (DTAF), which covalently binds to the host stroma and serves as a marker of the original corneal tissue. Using this technique, Tuft et al. have shown that there is virtually no difference between the amount of new fibrotic matrix deposited after PRK compared to lamellar keratectomy wounds in the rabbit. Even more important, immunohistochemical studies in primate corneas show no difference in the types and distribution of extracellular matrix proteins (collagen types VII, III, VI, keratan sulfate, fibrinogen, fibronectin, and laminin) that are deposited between PRK and manual keratectomy ( Figs. 2.12 and 2.13 ). As in the subepithelial region after PRK, there is a disorganized layer of type III collagen and the absence of normal keratin sulfate for at least 1 month after PRK. Subsequent deposition of fibronectin and laminin in the wound bed after reepithelialization suggests a close association between the increased numbers of anterior stromal keratocytes present and the wound-healing response (see Figs. 2.12 and 2.13 ). A widely held theory is that the deposition or loss of noncorneal associated matrix proteins such as type III collagen and keratin sulfate proteoglycans is responsible for the loss of corneal transparency. Corneal haze may also be due to the deposition of abnormal fibrillar collagen, which has larger collagen diameters, increased interfibril spacing, and more random orientations.

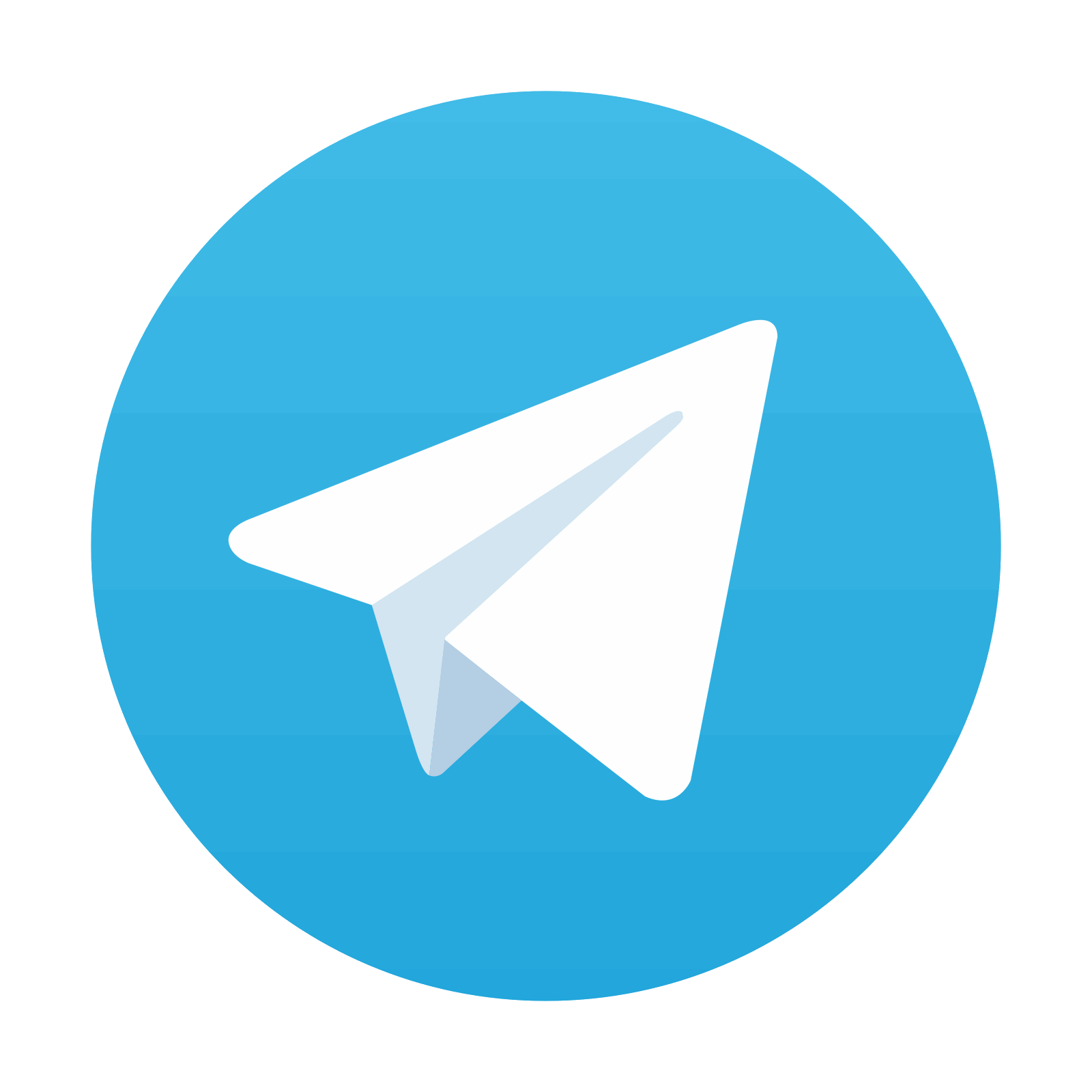
Stay updated, free articles. Join our Telegram channel

Full access? Get Clinical Tree
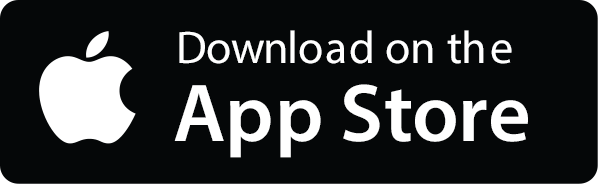
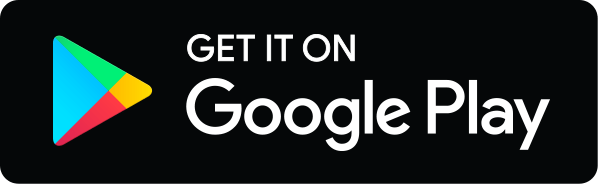
