Complications in Endoscopic Skull Base Surgery
Introduction
Recent technologic advances and a greater understanding of the intricate anatomy of the human skull base have fostered exponential growth of endoscopic skull base surgery (ESBS) to treat a large variety of benign and malignant conditions of the skull base. Over the past decade, neurosurgeons and otolaryngologists, working in accord, have transformed ESBS from its initial alternative role to that of standard of care for many pathologic processes involving the skull base.
As with any other paradigm shift in medicine, ESBS has faced some criticism and disbelief. Cerebrospinal fluid (CSF) leaks, nasal flora contamination and risk of postoperative infections, inability to comfortably reach or fully resect complex lesions and the steep learning curve necessary to master these techniques were but a few of the obstacles that the emerging field had to overcome. Slowly but consistently, most of these issues were addressed and resolved. Recent outcome studies have demonstrated that, although not perfect, endoscopic techniques are safe and effective when practiced by experienced hands in carefully selected patients.1–4 Nonetheless, as in traditional skull base surgery, the complex nature of skull base anatomy and diversity of diseases that affect the region leads to a certain degree of risk of surgery-related morbidity and mortality.
The aim of this chapter is to provide an overview of the complications related to ESBS. As with any surgical procedure, potential for treatment-related or disease-related complications exists in all phases of patient care (i.e., presurgical, intraoperative, and postoperative). Although discussion of presurgical complications is beyond the objectives of this chapter, consideration of potential treatment-related complications begins with the initial patient encounter. Likewise, risk reduction strategies and prevention of intraoperative and postoperative complications begin in the preoperative phase.
Scope of the Problem
The established complication rate for skull base surgery is variable because of the heterogeneity of conditions treated and the various approaches necessary to access lesions of the skull base. Most contemporary literature regarding surgical resection of malignant skull base tumors reports complication and mortality rates ranging from 30 to 50% and 0 to 7%, respectively.5,6 A recent international multi-institutional study aimed at identifying predictors of morbidity and mortality related to craniofacial resection for a variety of skull base lesions identified respective rates of 36.3% and 4.7% in a study population of 1,193 patients. In that study, the presence of comorbid medical conditions was a significant predictor of mortality, and comorbid medical conditions, previous radiation treatment, dural invasion, and brain invasion were significant predictors of postoperative complications.5
Note
The overall complication rate related to ESBS is not as well established, but appears comparable or even favorable to traditional techniques.1
While most of the same hurdles of traditional surgical approaches hold for ESBS, there are some differences, mainly because the sinonasal cavity serves as the corridor. In addition, ESBS is still in its infancy relative to traditional approaches. Techniques and protocols are not universal among the community of physicians treating skull base diseases. Furthermore, within institutions, the complication rates will undoubtedly fluctuate as techniques are mastered. In a recent review, Kassam et al1 reported on 800 ESBS cases performed for a variety of skull base diseases. Barring CSF leaks, the overall rate of complications related to ESBS was 9.3%. In their review, there was an overall CSF leak rate of 15.9%. However, as noted above, the postoperative CSF leak rate was dynamic, and with the introduction of pedicled flaps, the CSF leak rate was brought to less than 5%.7
Complication Avoidance
Note
Comprehensive complication management starts with risk reduction and avoidance.
First and foremost, the surgical team should be appropriately trained in both endoscopic and traditional open techniques. Although otolaryngologists are generally comfortable with endoscopic endonasal surgery, challenges arise when first attempting two-surgeon, three-handed or four-handed surgery. Accordingly, teams should mature by progressing from less complex to more complex cases as experience leads.
Anatomic Corridors in Endoscopic Endonasal Approaches
Endoscopic endonasal approaches may be organized as modules based on anatomical corridors in the sagittal and coronal planes:
Sagittal plane modules use corridors medial to the lamina papyracea and internal carotid artery (ICA) and include transcribriform, transplanum, transtuberculum, transsellar, transclival, and transodontoid approaches.
Coronal plane approaches are divided into anterior, mid, and posterior modules.
Anterior Plane Modules
Anterior plane modules provide both extraconal and intraconal access to the orbits. Orbital decompression for Grave disease is one example of an extraconal approach. Intraconal approaches generally use the corridor between the medial and inferior rectus muscles to address lesions medial to the optic nerve.
Midcoronal and Posterior Coronal Planes
The midcoronal and posterior coronal planes are divided into seven anatomical zones based on their relationship to the ICA. The “infrapetrous” and “suprapetrous” planes referred to in this discussion pertain to the plane to the petrous ICA, not necessarily the petrous bone. The seven anatomical zones are as follows:
Zone 1: the anterior petrous apex.
Zone 2: the midbody of the petrous bone below the level of the horizontal segment of the petrous carotid.
Zone 3: the suprapetrous region consisting of the quadrangular space. The quadrangular space is defined medially by the paraclival ICA, inferiorly by the horizontal segment of the petrous ICA, laterally by the second division of the trigeminal nerve, and superiorly by the course of the sixth cranial nerve within the cavernous sinus. Through this approach the Meckel cave and the gasserian ganglion can be reached.
Zone 4: the superior lateral cavernous sinus, representing the region through which the oculomotor (III), trochlear (IV), first division of the trigeminal (V), and abducens (VI) nerves traverse.
Zone 5: the transpterygoid/infratemporal space with direct access to the middle fossa.
Zone 6: the region of the condyle, the paramedian region located immediately lateral to the inferior third of the clivus and foramen magnum. It is anterolaterally bound by the eustachian tube and fossa of Rosenmuller, which mark the parapharyngeal ICA laterally. Superiorly it has its limit on the petroclival synchondrosis. Lesions in this region can involve the hypoglossal canal.
Zone 7: the region lateral to the parapharyngeal ICA. The approach for this region extends along the floor of the maxillary sinus and contains the lateral pterygoid plate and attached soft tissue. Most importantly, this region contains the jugular foramen posteriorly.8–11
A systematic approach to training, based on graduated mastery of approaches with progressive levels of difficulty has been recommended ( Table 10.1 ).4 The levels of difficulty depicted in Table 10.1 are important, as this stratification has significant implications on the potential complications that one may encounter in the perioperative period.
Principles of Endoscopic Endonasal Surgery
The specific techniques germane to endoscopic endonasal skull base surgery have been described extensively in the literature and will not be detailed in this chapter.3,4,8–13 However, there are certain key principles that should be consistently adhered to:
Choosing the correct corridor and creating sufficient room for unhindered three-handed or four-handed dissection
Meticulous dissection of the tumor or lesion
Minimizing trauma to surrounding neurovascular structures
Adequate reconstruction of the skull base to separate the intracranial and sinonasal cavities.
Note
The sinonasal cavity should be respected, as inadvertent mucosal trauma may lead to unnecessary sinonasal morbidity.
Surgical Planning
Beyond surgical training, the surgical team should incorporate a regular, standardized approach to surgical planning. Preoperative imaging should be obtained in advance of the procedure. In our program, we review imaging as a team at least weekly. Likewise, any available pathology should be reviewed. When not contraindicated by prolapse of meninges or tumor vascularity, neoplasms involving the sinonasal cavity should be biopsied either in the office or operative suite before definitive resection. This allows the surgeon to best inform the patient of the expected extent of resection, expected morbidity and potential risks based not only on size and location of tumor, but on pathology. Referral to appropriate medical services and/or anesthesiology allows for risk stratification and medical optimization.
Note
In cases where significant blood loss is anticipated, blood products should be made available.
Intraoperative Steps
Depending on the pathology, extent of disease, planned procedure, and medical comorbidities, further steps may be taken to avoid, or at least optimally treat, complications or intraoperative hurdles. Computer-assisted stereotactic navigation is widely used in ESBS. It is important to review the available imaging studies to ensure that they were performed with the appropriate protocol for use with these systems when needed.
Neurophysiologic monitoring, including somatosensory evoked potentials and surveillance of cranial nerves at risk during dissection, is used routinely at our institution. In addition to feedback regarding nearby cranial nerves, neurophysiologic monitoring of somatosensory evoked potentials may serve as a general indicator of cortical perfusion during management of carotid artery injury.
In cases where the carotid artery is encased in tumor, or where extensive dissection around the artery is necessary, preoperative imaging, including magnetic resonance angiography or computed tomography angiography, can be performed to gain an understanding of the intracranial vasculature. These studies can be used for computer-assisted stereotactic navigation. Additionally, angiography provides an accurate anatomical assessment of tumor vascularity, intracranial circulation and collateral circulation. However, to best determine if there is sufficient collateral flow to withstand occlusion of the ICA, angiography balloon occlusion xenon computed tomography is recommended.14
Intraoperative Complications
Note
The majority of intraoperative complications and the major sources of morbidity in skull base surgery relate to intraoperative neural or vascular injury. As might be expected, the incidence of neural and vascular complications is higher in more complex cases, specifically Level IV and V procedures.
Bleeding and Vascular Injury
Intraoperative bleeding remains one of the most significant challenges to safe resection of skull base tumors. As in any approach, the location, size, and vascularity of the tumor, the length of the case, and any systemic factors leading to a bleeding diathesis contribute to blood loss. In ESBS, the sinonasal corridor may present a significant factor in overall blood loss because of the vascularity of sinonasal mucosa.
Bleeding Classification
In general, bleeding may be categorized based on two factors; the source (venous or arterial) and the rate (low flow or high flow):
Low-flow venous bleeding is most commonly encountered as diffuse mucosal oozing, whereas an example of high-flow venous bleeding is that which occurs from the cavernous sinus.
Low-flow arterial bleeding occurs from small vessels, such as perforating vessels, whereas high-flow arterial bleeding includes medium to large vessels, such as the sphenopalatine or internal maxillary arteries, as well as the ICA.
Generally, this categorization will allow the surgeon to determine the most appropriate method to achieve hemostasis. However, the choice is also influenced by factors such as the type of tissue (mucosa, bone, or tumor); the proximity of neurovascular structures; and the area (extradural or intradural) of dissection.12
Low-Flow Venous Bleeding
Low-flow venous oozing is commonly encountered during the dissection and development of the sinonasal corridor. In our experience, warm saline irrigation is quite effective in addressing this type of bleeding. A recommended temperature for irrigation is 40 to 42°C.12 Especially during lengthy cases, it is important to keep an account of the irrigation used to maintain an accurate assessment of blood loss. For persistent focal low-flow venous bleeding, or in some cases of more brisk venous bleeding, such as that which occurs from focal cavernous sinus exposure, application of hemostatic agents, such as Floseal (Baxter International Inc.; Deerfield, IL, USA) or Avitene (Ethicon Inc., Johnson and Johnson; Somerville, NJ, USA), is frequently successful.

Arterial Bleeding
Although the rate of arterial bleeding is generally proportional to the size of the vessel, the neurologic consequences of arterial damage are not necessarily size dependent. This fact underscores the need to respect arterial vessels of all sizes, practicing meticulous dissection with judicious use of cautery ( Fig. 10.1 ).
Small arterial damage is generally encountered during dissection of tumor from the surrounding neurovascular bed. In appropriately selected patients in whom there is no significant cortical cuff or critical neurovascular structure (i.e., optic nerve) between the tumor and sinonasal corridor, endoscopic endonasal approaches allow resection of tumor with minimal brain retraction or dissection. Nonetheless, the interface between the tumor and the underlying neurovascular stroma must be respected and dissection at the interface should be meticulous. In the anterior cranial fossa, frontal polar arteries are encountered during dissection of tumors such as olfactory neuroblastoma or olfactory groove meningioma. Similarly, in the middle and posterior cranial fossae, small perforating vessels supplying the brainstem and optic chiasm must be preserved to prevent potentially catastrophic deficits.15
In the aforementioned review of 800 cases, Kassam et al1 reported a total of seven major intraoperative vascular complications that encompassed 0.9% of cases. In one patient, avulsion of a P1 perforator resulted in severe, but transient dysphasia. There were three patients with significant permanent deficits resulting from intraoperative vascular injury: one patient was rendered quadriplegic by a pontine bleed, one patient developed hemiparesis following an internal maxillary artery laceration, and one patient developed unilateral lower limb paresis following a frontopolar avulsion. In one patient, an ophthalmic artery avulsion occurred, but there were no sequelae as the patient was already blind in that eye.
In general, injury to small or medium-sized vessels, such as those mentioned here, are encountered during exposure or dissection of the lesion. Control of bleeding from these vessels depends not only on the flow rate, but also on adjacent anatomy. Bipolar electrocautery is quite effective and efficient. When appropriate, it is the authors’ method of choice. However, thermal damage to adjacent structures may preclude the use of bipolar electrocautery. In this situation, application of hemostatic agents, such as Floseal or Avitene are efficacious, especially when the surrounding anatomy is amenable to the application of gentle pressure to these agents to form a “seal” at the site of the defect.
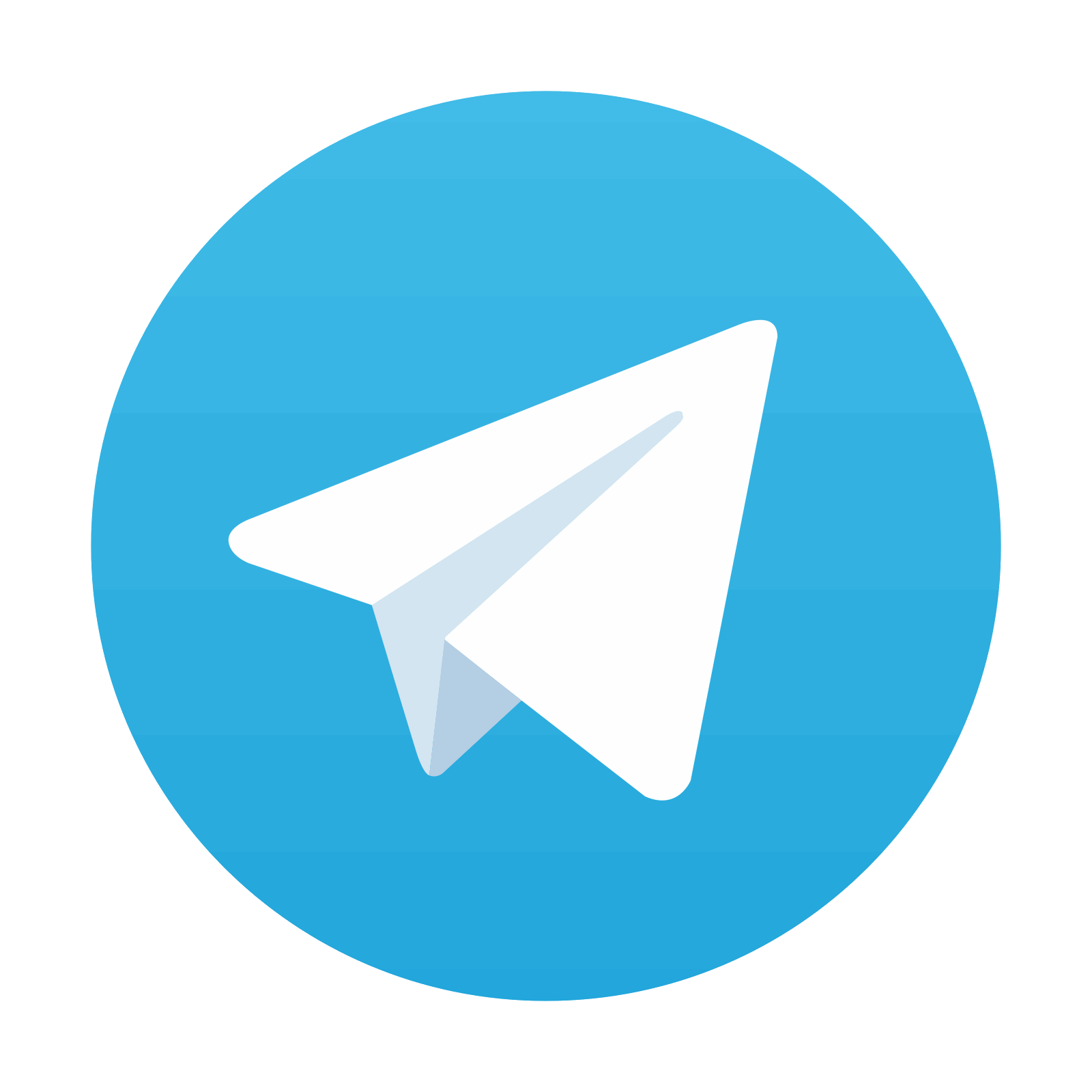
Stay updated, free articles. Join our Telegram channel

Full access? Get Clinical Tree
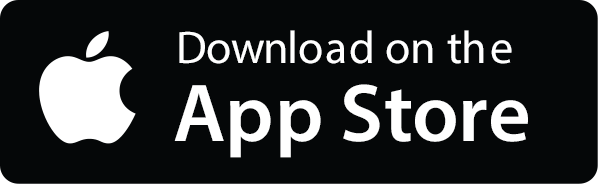
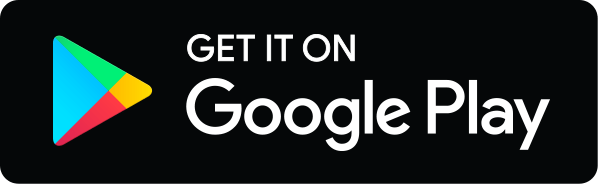
