Abstract
Age-related macular degeneration (AMD) is the most common form of irreversible vision loss in the Western world. AMD is classified into the dry, nonexudative, nonneovascular form and the wet, exudative, and neovascular type. The presence of centrally involving geographic atrophy (GA) in eyes with dry AMD or choroidal neovascularization in eyes with wet AMD represents the advanced form of AMD, which can cause irreversible vision loss. While treatment of wet AMD has evolved over the last decade, there are currently no therapeutic options for dry AMD. Imaging of the choroid, outer retina, and circulation may help us better comprehend the etiology and evaluate therapies for dry AMD. The ability to use imaging to correctly diagnose, monitor disease progression and the efficacy of new therapies, and predict prognosis is therefore very important. Various imaging modalities such as fundus photography, fundus autofluorescence, optical coherence tomography (OCT), OCT angiography, microperimetry, fluorescein angiography, and indocyanine-green angiography can be used in these evaluations and can help characterize drusen subtypes, GA, and choroidal anatomy. The most effective method to evaluate dry AMD may require incorporation of information from multiple imaging modalities to maximize the strength of each method.
Keywords
Age-related macular degeneration, Geographic atrophy, Drusen, Imaging, Fundus photography, Optical coherence tomography, Optical coherence tomography angiography, Fluorescein angiography
Introduction
Age-related macular degeneration (AMD) affects 7–8 million people in the United States (US) with a prevalence of 6.5% in persons aged 40 years and older, and it is the most common cause of blindness in the Western world. In the 1990s, the prevalence of late-stage AMD (comprising atrophic or neovascular forms) was estimated to be 1.6% in the US, with similar prevalence at that time outside of the US with rates of 1.7% in the Netherlands, 1.9% in Australia, and 1.1% in Italy. In 2005–08, the prevalence of the severe dry form of AMD in the US was estimated to be about 0.8% in the National Health and Nutrition Examination Survey.
Increased age, diet, and smoking are the major known risk factors, and inflammatory mediators such as complement factors and vascular endothelial growth factor (VEGF) may play a role in pathogenesis. As the population ages, the prevalence of AMD-related eye disease will increase, which may present a major healthcare burden. AMD is classified into the dry, nonexudative, nonneovascular form and the wet, exudative, and neovascular type. Wet AMD is currently treated with intravitreal injections of anti-VEGF medication to reduce progressive vision loss due to choroidal neovascularization (CNV) and prevent permanent damage from macular scarring. In dry AMD, vitamin supplementation with the Age Related Eye Disease Study (AREDS) 2 formulation has been shown to reduce the risk of developing advanced AMD. Clinical trials are currently evaluating medications that may halt or reverse vision loss due to severe dry AMD.
In the early stages of dry AMD, vision loss is rare. This early stage is characterized in the AREDS study by at least 15 small (<63 μm) yellow subretinal deposits called drusen, less than 20 medium-sized (63–125 μm) indistinct drusen, and/or retinal pigment epithelium (RPE) changes such as hyper- or hypopigmentation. The presence of at least one large (>125 μm) druse, more than 20 medium-sized drusen, and/or noncentrally involving geographic atrophy (GA) represents the intermediate stage of AMD. Larger drusen may then coalesce into drusenoid RPE detachments (RPED), which can progress to be GA, characterized by variable loss of photoreceptors and RPE. The presence of GA involving the central macula or CNV represents the advanced form of AMD, which typically results in decreased vision and loss of fixation.
The long and short posterior ciliary arteries are the major blood supply to the choroid. Modifications of the choroidal anatomy occur in dry AMD, including loss or narrowing of the choriocapillaris layer which becomes more pronounced in later stages of the disease. Furthermore, decreased choroidal blood flow in the fovea has been associated with increased drusen extent, and the distribution of drusen has been shown to be closely related to areas of choriocapillaris dropout. Histopathological analysis has shown that the choriocapillaris becomes less dense, narrowed, retracted away from Bruch’s membrane, widened by the intercapillary pillars, and defenestrated. Inflammatory cells fill the space formerly occupied by choriocapillaris. One study of 325 eyes compared the choroidal thickness between eyes with intermediate AMD, eyes with advanced AMD, and control eyes without AMD. Choroidal thickness was significantly reduced in eyes with advanced AMD compared with control eyes. However, after adjusting for age, no statistical significance was found between the three groups. Analysis demonstrated that choroidal thickness decreased with advancing age, confirming that age is a confounding factor in the debate of whether or not choroidal thinning occurs in AMD.
Introduction
Age-related macular degeneration (AMD) affects 7–8 million people in the United States (US) with a prevalence of 6.5% in persons aged 40 years and older, and it is the most common cause of blindness in the Western world. In the 1990s, the prevalence of late-stage AMD (comprising atrophic or neovascular forms) was estimated to be 1.6% in the US, with similar prevalence at that time outside of the US with rates of 1.7% in the Netherlands, 1.9% in Australia, and 1.1% in Italy. In 2005–08, the prevalence of the severe dry form of AMD in the US was estimated to be about 0.8% in the National Health and Nutrition Examination Survey.
Increased age, diet, and smoking are the major known risk factors, and inflammatory mediators such as complement factors and vascular endothelial growth factor (VEGF) may play a role in pathogenesis. As the population ages, the prevalence of AMD-related eye disease will increase, which may present a major healthcare burden. AMD is classified into the dry, nonexudative, nonneovascular form and the wet, exudative, and neovascular type. Wet AMD is currently treated with intravitreal injections of anti-VEGF medication to reduce progressive vision loss due to choroidal neovascularization (CNV) and prevent permanent damage from macular scarring. In dry AMD, vitamin supplementation with the Age Related Eye Disease Study (AREDS) 2 formulation has been shown to reduce the risk of developing advanced AMD. Clinical trials are currently evaluating medications that may halt or reverse vision loss due to severe dry AMD.
In the early stages of dry AMD, vision loss is rare. This early stage is characterized in the AREDS study by at least 15 small (<63 μm) yellow subretinal deposits called drusen, less than 20 medium-sized (63–125 μm) indistinct drusen, and/or retinal pigment epithelium (RPE) changes such as hyper- or hypopigmentation. The presence of at least one large (>125 μm) druse, more than 20 medium-sized drusen, and/or noncentrally involving geographic atrophy (GA) represents the intermediate stage of AMD. Larger drusen may then coalesce into drusenoid RPE detachments (RPED), which can progress to be GA, characterized by variable loss of photoreceptors and RPE. The presence of GA involving the central macula or CNV represents the advanced form of AMD, which typically results in decreased vision and loss of fixation.
The long and short posterior ciliary arteries are the major blood supply to the choroid. Modifications of the choroidal anatomy occur in dry AMD, including loss or narrowing of the choriocapillaris layer which becomes more pronounced in later stages of the disease. Furthermore, decreased choroidal blood flow in the fovea has been associated with increased drusen extent, and the distribution of drusen has been shown to be closely related to areas of choriocapillaris dropout. Histopathological analysis has shown that the choriocapillaris becomes less dense, narrowed, retracted away from Bruch’s membrane, widened by the intercapillary pillars, and defenestrated. Inflammatory cells fill the space formerly occupied by choriocapillaris. One study of 325 eyes compared the choroidal thickness between eyes with intermediate AMD, eyes with advanced AMD, and control eyes without AMD. Choroidal thickness was significantly reduced in eyes with advanced AMD compared with control eyes. However, after adjusting for age, no statistical significance was found between the three groups. Analysis demonstrated that choroidal thickness decreased with advancing age, confirming that age is a confounding factor in the debate of whether or not choroidal thinning occurs in AMD.
Choroidal Imaging of Dry Age-Related Macular Degeneration
Fundus Photography
Color or red-free fundus photographs ( Figs. 6.1–6.5 ) provide a view of the retina similar to clinical examination. Typical drusen in AMD are yellow deposits between the RPE and Bruch’s membrane. Drusen are the earliest sign of dry AMD and are made up of lipids, resulting from the incomplete disposal of shed photoreceptor discs and cellular waste products. There are several types of drusen with different levels of risk. Drusen can be small, hard, round, distinct, and scattered far apart from each other. This type may not create vision problems for a long time and may not even be an indication of macular degeneration. Drusen can become larger, softer, and closer together. Their edges are less distinct. When drusen are at this stage, there is a greater risk for developing wet AMD and secondary vision loss. These drusen can also disrupt the layers of the retina and lead to RPED.





Hard drusen appear as yellowish-white pin-point lesions, while soft drusen are larger and may appear less distinct or confluent. Soft drusen range in size from 63 to 1000 μm in diameter. In contrast to hard and soft drusen, basal laminar drusen (cuticular drusen) are clusters of 50–75 μm subretinal or sub-RPE deposits and subretinal drusenoid deposits (reticular pseudodrusen) are light gray interconnected collections just above the RPE that may be much more extensive than typical drusen. Reticular pseudodrusen may be very subtle and harder to appreciate using ophthalmoscopy or color fundus photograph. However, scanning laser ophthalmoscopy with near-infrared reflectance demonstrates subtle changes in gray-scale tones, making reticular pseudodrusen appear darker than surrounding retina. Cuticular drusen and reticular pseudodrusen tend to be located more peripherally at the arcades compared to drusen that accumulate in the foveal region.
Different light filters aid in differentiating the drusen subtypes. Blue light filters aid in detecting reticular pseudodrusen as the subjacent RPE absorbs blue light, thus making a dark background in contrast to the reticular pseudodrusen. Conversely, in red light, soft drusen appear more obvious. Using a red-free filter can help to differentiate drusen from exudates and hemorrhage, as blood appears dark using the red-free filter, while drusen and RPE loss remain lighter in hue.
As the RPE is a darkly pigmented layer, loss of the RPE in GA can be seen as lighter areas in the macula. The deeper choroidal vessels may be more easily visualized beneath areas of GA. GA can look similar to age-related choroidal atrophy (ARCA) in which the choriocapillaris and choroidal vessels are lost and the remaining vessels appear to enlarge, but a few key differences can distinguish the two diseases. GA is usually well defined, and the associated RPE loss allows the underlying choroidal vessels to be more easily visible. In contrast, ARCA shows generalized choroidal thinning.
Fundus Autofluorescence
Fundus autofluorescence (FAF) imaging ( Figs. 6.2, 6.4, and 6.5 ) is based on the preexisting fluorescent properties of ocular pigments in the retina. It generates images based on the distribution of lipofuscin in the RPE and is thus a sensitive marker for RPE cell dysfunction. Lipofuscin contains A2E, which is the major natural fluorophore in the RPE. Excessive lipofuscin deposition is considered pathologic and is associated with visual loss. The intensity of FAF corresponds to the accumulation of lipofuscin, which increases with aging, RPE cell dysfunction, or an abnormal metabolic load on the RPE. Some of the causes of hyperautofluorescence include sub-RPE drusen, excessive RPE lipofuscin accumulation, and retinal thinning, producing a window defect over RPE, AMD, or the occurrence of fluorophores anterior or posterior to the RPE cell monolayer. Causes of hypoautofluorescence signal may include RPE loss or atrophy, intraretinal fluid, reduction in RPE lipofuscin density, fibrosis, or the presence of luteal pigment.
Specific patterns of FAF have been characterized in dry AMD. Areas of well defined hyperautofluorescence have been shown to be clinically correlated with drusen and can be seen adjacent to GA. The edges of soft drusen tend to fluoresce greater than the center of the druse. FAF may image reticular pseudodrusen earlier than fundus photography, revealing a dark reticular pattern often located near the arcades.
In regions of GA, FAF will show hyperautofluorescence early in the disease course followed by a sharply demarcated hypoautofluorescence. The early hyperautofluorescence of GA is hypothesized to be due to dying RPE-releasing lipofuscin. Sometimes, a rim of dying RPE can also be hypoautofluorescent. Therefore, the size of GA on FAF imaging may differ from what is observed clinically or via color fundus photography, although FAF is considered slightly more reliable for detecting GA, particularly in cases of low contrast, adjacent depigmentation, and with small areas of GA. Near-infrared autofluorescence may yield slightly more information that FAF alone, showing focal areas of increased intensity in the transition zone of GA not seen with FAF, which may be associated with thickening of the RPE.
Different classifications have been proposed in the literature to describe features of FAF in AMD. Bindewald et al . described eight phenotypic patterns of FAF changes in early AMD (normal, minimal change, focal increased, patchy, linear, lacelike, reticular, or speckled), and further defined four FAF patterns adjacent to GA: focal, banded, patchy, or diffuse. In a study by Holz et al ., specific patterns of FAF in eyes with GA were compared with rates of atrophy enlargement. GA growth was slowest in eyes with no abnormal autofluorescence or a focal pattern and fastest in eyes demonstrating a diffuse or banded pattern. The pattern of autofluorescence was the greatest independent risk factor for GA progression evaluated in their study. Furthermore, larger areas of hyperautofluorescence focally in the 500-μm margin around areas of GA have been found to be associated with an increased rate of GA growth. This may have some value as a prognostic marker for progression of GA.
Optical Coherence Tomography
Optical coherence tomography (OCT) ( Figs. 6.1–6.5 ) is a valuable imaging modality to detect and characterize features of dry AMD, including differentiation of drusen subtypes and to evaluate GA and choroidal features. Drusen are rounded subretinal deposits on OCT B scans between the RPE and Bruch’s membrane with varying heights and variable degrees of continuity with adjacent drusen. Meanwhile, reticular pseudodrusen are seen between the external limiting membrane and the RPE, and cuticular drusen have a characteristic “sawtooth” pattern of triangular or spheroid deposits. RPE overlying cuticular drusen may be rarefied, increasing light penetration through that area, and adjacent RPE may be thickened causing signal attenuation below. Reduced photoreceptor density and photoreceptor abnormalities have been described overlying drusen using OCT.
Total drusen volume can be calculated by automated algorithms on OCT devices. Volume may therefore be easily monitored as drusen number increases or decreases due to progression of GA. OCT and the total drusen volume can also be used for classification purposes and predicting disease prognosis.
In addition, on OCT B scans, pigment migration may be seen adjacent to areas of irregular RPE. In more advanced stages, absent RPE and increased light penetration through the missing RPE represent areas of GA, often occurring perifoveally progressing into the fovea in later disease. Overlying and adjacent loss of the photoreceptors and ellipsoid layer can be seen, suggesting that this loss occurs prior to loss of the RPE. Underlying choriocapillaris degeneration can also be apparent.
Choroidal thickness is typically decreased in eyes with dry AMD compared with normal eyes, with a tendency toward reduced choroidal thickness with increasing stage of disease. Both Sattler’s and Haller’s layer thicknesses are reduced compared to eyes without AMD. Similarly, drusen load and choroidal thickness have been shown to be inversely correlated. It has been hypothesized that the decreased choriocapillaris thickness reduces metabolic support to the RPE and lowers its ability to metabolize byproducts, therefore promoting drusen formation. While choroidal thickness can be evaluated using spectral domain (SD)-OCT, it is easier to visualize using enhanced depth imaging (EDI)-OCT and swept source (SS)-OCT. EDI-OCT provides greater detail of the choroidal anatomy by displacing the zero delay line (point of maximum signal) from the standard position at the posterior vitreous to deeper in the tissue toward the choroid. Similarly, SS-OCT offers enhanced visualization of the choroid and the choroidoscleral interface as compared with SD-OCT. This is because the longer SS-OCT wavelength and decreased fringe washout of the SS-OCT system provide better penetration through pigmented tissue such as the RPE. Eyes with reticular pseudodrusen have been shown to demonstrate even thinner choroids than in eyes with early AMD. Reduced choroidal thickness may be important as it has been correlated with increased rates of GA progression.
En face OCT provides a view of the retina and choroid that follows the natural contour of the retinal layers. While traditional OCT provides structural information as opposed to functional (blood flow), the choroidal vessels can be visualized as hyporeflective cords beneath the RPE on en face OCT. The choriocapillaris appears as a dense granular network with individual capillaries difficult to distinguish. Sattler’s layer demonstrates relatively tightly arranged interlacing strands of medium-sized choroidal vessels compared with the larger loosely packed vessels of Haller’s layer with larger stromal regions between the vessels. In eyes with AMD, areas of choriocapillaris dropout are apparent beneath drusen, and a decreased overall density of the choriocapillaris network is often apparent. Likewise, in AMD eyes demonstrating choroidal thinning, the densities of the innermost layers of the choroidal vasculature are more readily decreased compared with Haller’s layer. In a study by Sohrab et al ., en face OCT was used to visualize the choroid in 58 eyes with either no disease, early AMD, or RPD. All 33 eyes with RPD and 5 of 11 eyes (45%) with early AMD had no visible choriocapillaris and instead demonstrated larger vessels that resembled Sattler’s layer. Eyes with thinner choroids tended also to have decreased vascular density. Eyes with early AMD had decreased vascular density compared with controls, and eyes with RPD had increased vascular density compared with controls. En face OCT can also be used to detect and quantify GA. A study by Pillotto et al . used automated segmentation of the outer retina using a reference point 25 μm above Bruch’s membrane and of the choroid using a reference point 50 μm below Bruch’s membrane to evaluate GA in comparison with FAF imaging. Decreased signal in the outer retinal slab and increased signal in the choroid slab correlated to GA. Intraoperator and interoperative agreement was excellent using en face OCT at both segmentations. FAF measurements of GA correlated well with measurements at both segmentation levels but more highly with measurements at the outer retinal segmentation.
Optical Coherence Tomography Angiography
OCT angiography (OCTA) ( Fig. 6.2 ) is a novel imaging modality that employs faster imaging speeds than traditional OCT B scans to create an angiographic map without utilizing intravenous contrast dye. OCTA software compares consecutive OCT B scans taken at a given cross-section of the retina, assuming that areas of signal difference (decorrelation) between scans represent movement due to erythrocytes in the retinal vasculature. En face segmentation provides high-resolution imaging of the retinal and choroidal circulation with corresponding structural B scans. Either an SD or a swept-source platform can be used. In theory, the longer wavelength of SS may allow for increased signal penetration through the RPE and thus deeper imaging into the choroid. The choriocapillaris of a normal eye appears as a dense homogenous net of fine capillaries in the macula.
In dry AMD, macular choriocapillaris density as seen on the en face OCT angiogram decreases. These alterations may occur generally or focally beneath areas of drusen. Choriocapillaris changes become more marked with increasing disease severity. In eyes with GA, near-complete atrophy of the underlying choriocapillaris is seen with the margins of loss approximately delineating the area of atrophy. Larger choroidal vessels can be seen pushed up into the plane of the decreased choriocapillaris. A study by Palejwala et al . demonstrated that CNV can be detected using OCTA in some eyes that appear to have dry AMD on standard SD-OCT and fluorescein angiography (FA). This demonstrates OCTA’s potential utility in monitoring AMD disease activity.
Microperimetry
Microperimetry provides functional and structural evaluations of the retina concurrently by measuring the fixation patterns and light differential threshold over retinal loci. Macular sensitivity is reduced over large drusen and pigment abnormalities and correlated with abnormalities seen on FAF. This functional reduction can be seen even when no measurable vision loss had occurred. Furthermore, low microperimetry values in eyes with AMD were associated with disruption of the ellipsoid layer.
Fluorescein Angiography
Dye-based angiography is more commonly used to look for leakage due to CNV in the wet form of AMD. However, changes in dry AMD can be appreciated when FA is performed. Delayed choroidal filling is demonstrated on FA. Some soft drusen stain in later frames on FA, although this is variable depending on the overlying RPE and composition of the drusen. Reticular pseudodrusen may show minimal hypo- or isofluorescence. In contrast, cuticular drusen hyperfluoresce most markedly. This is in part because of significant RPE thinning at their apexes and therefore decreased melanin in the path of light allowing for greater light emission. Therefore, cuticular drusen have pinpoint hyperfluorescence centrally. The thickened RPE surrounding the cuticular drusen may show as darker, thereby highlighting them even more. In areas of GA, the RPE loss causes a window defect showing hyperfluorescence in the transit phase with visualization of choroidal vessels below.
Indocyanine-Green Angiography
Prior to OCTA, indocyanine-green angiography (ICGA) was one of the only imaging modalities to visualize the choroidal circulation in vivo. Using ICGA, hard drusen may hyperfluoresce after 2–3 min, while soft drusen hypo- or isofluoresce. Choroidal vascular permeability can be seen in the late phase of ICGA in eyes with polypoidal choroidal vasculopathy and central serous chorioretinopathy. CNV can appear as hyperlucent plaques. Area dilution analysis using ICGA has demonstrated significantly delayed and heterogenous choroidal filling, particularly perifoveally in eyes with dry AMD vs normal controls.
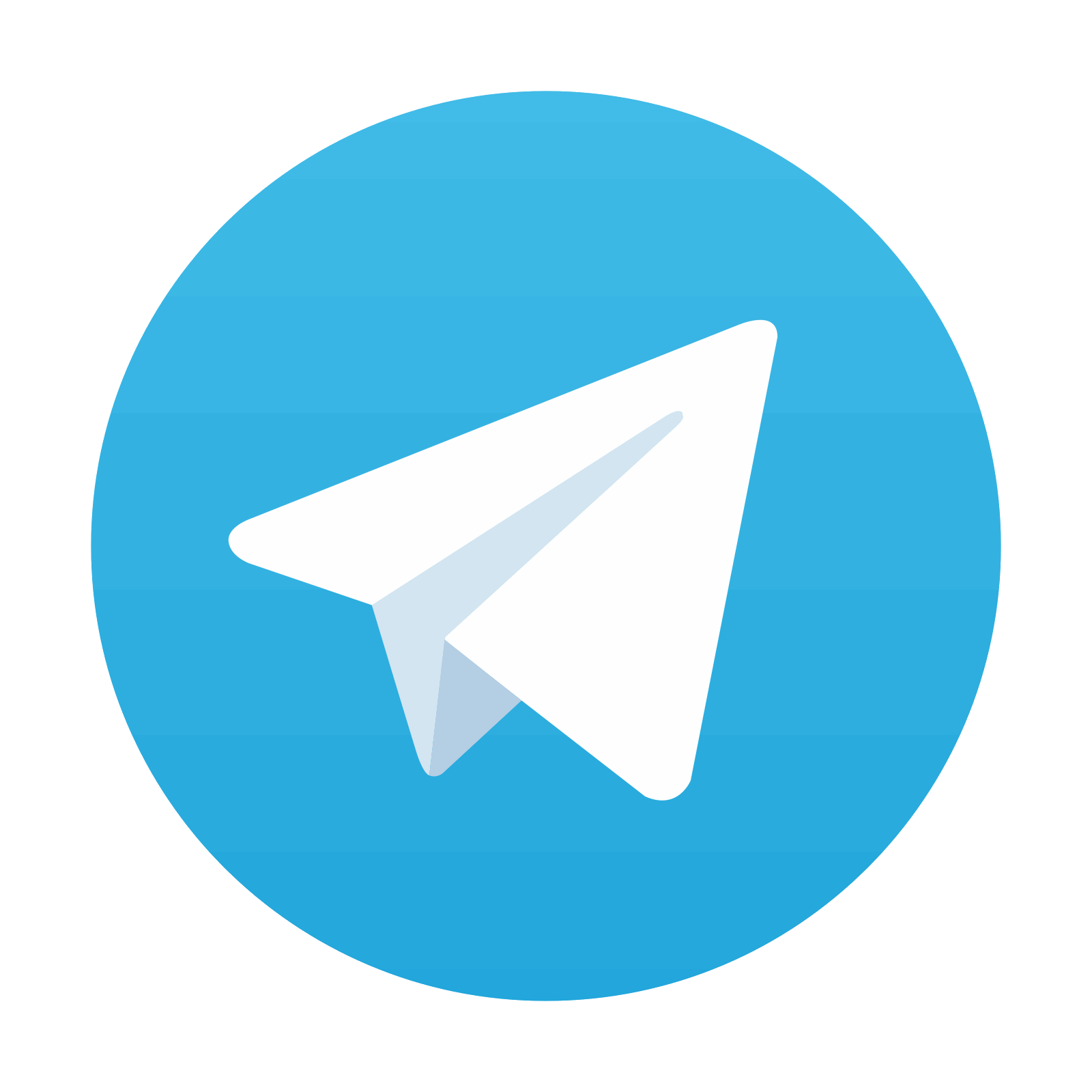
Stay updated, free articles. Join our Telegram channel

Full access? Get Clinical Tree
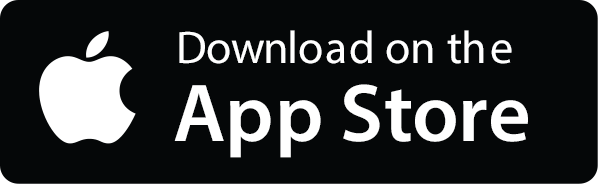
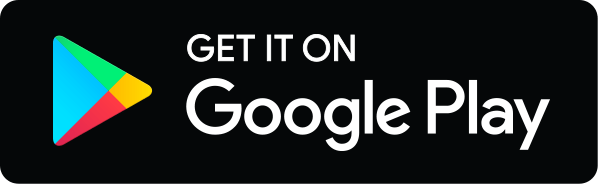