Chapter 4 Physiology and Pathophysiology of Nasal Breathing
4.1 Introduction
The nose is not only the gateway between the airways and the environment. One of the main roles of the nose is its respiratory function. During inspiration, the air has to be tempered, humidified, and cleaned. These are important prerequisites for an undisturbed exchange of gas in the lungs. Alveolar air temperature should be of body temperature and 100% humid. Additionally, the air must be cleaned for protection of the lower airways.
The respiratory performance of the nose is an enormous commitment, as, during breathing at rest, 6 liters of air per minute flow at a local speed of up to 20 m/s through it 1 and have to be conditioned and cleaned in the meantime.
In order to fulfil this task, the nose has a specific shape. Sufficient knowledge of the interaction between shape and function of the nose helps the rhinosurgeon to not only improve air passage of the nose, but also to maintain and reconstruct structures that are important for the respiratory function of the nose. On the basis of experiments on fluid dynamics, 2 , 3 we shall now review the structure of the nose from a functional point of view.
4.2 Preconditions for the Respiratory Function of the Nose
The most important precondition for nasal function is an undisturbed passage. High airway resistance consequently leads to mouth breathing and thus bypasses the nose. The reason for high airway resistance is a loss of energy due to friction of the streaming molecules with the wall and between each other. In a narrow section, molecules stream closer together and to the wall, thereby increasing the friction.
Another reason for high resistance is friction caused by turbulence. Turbulent streaming particles flow not only forward but also sideways. Therefore, the molecules frequently hit each other and the wall, releasing kinetic energy. For that reason, strong turbulence provokes high airway resistance.
The resulting high nasal airway resistance can be caused by narrowness as well as a pathologically increased degree of turbulence. Both factors induce airway resistance to varying degrees.
The second precondition for the respiratory function is contact between the streaming particles and the mucosa. In laminar flow (i.e., all particles streaming parallel to the wall), only the particles flowing nearest to the wall have contact with the mucosa. More central streaming particles are neither warmed, nor humidified, nor cleaned. Not until sideways movement arises, as in turbulent flow, do particles close to the wall leave their place and give way to particles moving from the center toward the mucosa. On the other hand, high degrees of turbulence extract too much thermal energy and moisture of the mucosa, causing sicca symptoms. The nasal airflow, therefore, should neither be overly laminar nor intensely turbulent. A well-balanced turbulent behavior as a warrant for sufficient air–mucosa contact is a prerequisite for the respiratory function of the nose.
The mucous surface of the nose is especially enlarged by the turbinates, which provide effective exchange of thermal energy and humidity.
Surgical consequence: As little mucosa reduction as possible!
Contact between air and this large mucous surface is maintained by distribution of the inspired air over the entire cross section of the nasal cavum. Moreover, functional efficiency is increased by deceleration of local flow velocity at the nasal turbinates.
The third precondition is that the mucosa be supplied with thermal energy and fluid for humidification. These prerequisites are fulfilled by the blood circulation and are in coherence with the nasal cycle. The erectile tissue enables the turbinates to cyclicly swell. One side of the nose is in its working phase, with an unimpeded air passage and increased turbulence, conditioning the air. At the same time, the contralateral side, in its resting phase with high airway resistance and low turbulence, stores energy and moisture.
Surgical consequence: One main target of functional rhinosurgery is to create adequate space for the physiological congestion and decongestion of the turbinates during the nasal cycle.
4.3 The Correlation between Shape and Function of the Nose
In order to understand the correlation between shape and function of the nose, Bachmann assigned common structural elements with familiar effects on flow, known from the physics of fluid dynamics, to discrete sections of the nose. Bachmann′s synopsis 4 can be enhanced by additional experiments on fluid dynamics 2 , 3 ( Fig. 4.1 ).

The actual functional area of the nose is its center, which contains the largest mucosal surface. There the nose serves its respiratory function.
4.3.1 Inspiration
The nasal vestibule, the internal ostium (isthmus nasi), and the anterior cavum are upstream of the functional area ( Fig. 4.1a ). This part is termed the inflow area. The posterior cavum, including the choana and the nasopharynx, is located downstream of the functional area. This part is named the outflow area.
The nasal vestibule has the shape of a bend with a decrease in cross-sectional area like a nozzle ( Fig. 4.1a ). The first effect is to direct the lateral and bottom-up approach of air toward the functional area of the nose ( Fig. 4.2a ). Therefore, the correct bearing of the vestibule to the cavum is of great importance. This is the case if the angle between nose and lip is 90 to 100 degrees. A downward rotated vestibule (nasolabial angle < 90 degrees) directs inspired air toward the upper cavum ( Fig. 4.2b ). The lower turbinate has no contact with the air and thus is not available for respiratory function. An oversized nasolabial angle (> 100 degrees) guides the air through the lower nose while not ventilating the upper parts ( Fig. 4.2c ).

Surgical consequence: Preservation and reconstruction of a nasolabial angle between 90 and 100 degrees should be aspired to in functional rhinosurgery.
The constriction of the cross-sectional area from the outer to the inner ostium of the nose produces a nozzle effect in the vestibule ( Fig. 4.1a ). The degree of turbulence is reduced in a nozzle. This effect is important because the inspired air has to pass through the narrowest part of the nose, the ostium internum, next. In this narrow sector, turbulent flow leads to very high flow resistance. Fig. 4.3 shows the flow in inspiratory direction in a nose model. Due to the forward movement of air molecules parallel to the wall during laminar flow, a sharp border between the flowing color particles and the flowing medium can be seen. During turbulence, additional sideward movements lead to a mixing of color and medium and thereby yield a diffuse coloring. In Fig. 4.3 , laminar flow in the narrowest part of the nose, the isthmus, is shown. In the region of turbinates, flow is turbulent.

Expansion of the ostium internum reduces or suspends the nozzle effect of the vestibulum. Consequently, the flow entering the cavum nasi is turbulent (“ballooning phenomenon”).
Surgical consequence: In surgery of the inner ostium, the expansion must not be made too great.
In the inspiratory direction, the internal ostium is of a concave shape 5 ( Fig. 4.1 ). As is known from fluid dynamics, the effect of a concave opening on flow course is the same as a concave lens acting on beams of light. 2 Thus, a diverging of the flow lines within the functional area is achieved, which has a positive impact on flow distribution all over the cross-sectional area of the functional area. Fig. 4.4 shows the flow in a nose model without a vestibule. The diverging flow lines after perfusion of the inner ostium can be seen.

Surgical consequence: Due to the fact that at its upper area the inner nostril is made of the caudal margin of the triangular cartilage, it is important to perform surgical procedures in the area of the inner nostril in such a way that when making resections at the caudal margin of the triangular cartilage the concave shape of the ostium internum is maintained.
As a result of the expansion of the cross-sectional area from the ostium internum to the beginning of the concha region, the anterior cavum is shaped and acts like a diffuser ( Fig. 4.1a ). In a diffuser, the local flow velocity is reduced and turbulence arises. Both effects are important preconditions for sufficient mucous membrane contact with the flowing particles in the functional area. The slowing of the flow as well as the arising turbulence is dependent on the dimensions of cross-sectional area expansion in the diffuser. With growing expansion of the cross-sectional area, the degree of turbulence increases, while the local flow velocity decreases.
There is a mechanism in the nose to regulate such effects. The expansion of the cross-sectional area alters with the swelling of the septum′s erectile body and the erectile head of the inferior turbinate ( Fig. 4.5 ). When swelling is at a minimum (right side in Fig. 4.5 ), the expansion of the cross section is great. This corresponds to the working phases in the nasal cycle. The flowing air becomes increasingly turbulent, while the local flow velocity decreases. These are the preconditions for the warming up, moistening, and cleaning of the air. During the resting phase, the increase of the cross-sectional area diminishes due to the swelling of the septum′s erectile body and the head of the inferior turbinate (left side in Fig. 4.5 ). The predominantly laminar flow allows the mucous membrane to accumulate thermal energy and moisture.

As a result of external nose deformations and septal deviations, the diffuser is often deformed. In such cases, the diffuser cannot fulfill its turbulence-regulating function.
Surgical consequence: One task of functional rhinosurgery is the reconstruction and/or maintenance of the diffuser. The important structures regulating turbulences (head of the inferior turbinate, septum′s erectile body) must be preserved.
In terms of flow physics, the region of turbinates is a slitlike space that increases mucous membrane surface area. A slitlike space is important in order to keep the flow path of particles close to the mucous membrane. An important precondition for the flow distribution over the entire cross-sectional area is a constant slit-like space. If partial or total resection of the turbinates in a small section is done, then a wider space is created. The flow yields to the narrow portion of the cavum and instead flows exclusively through this wide portion following the principle of least resistance (compare Figs. 4.3 and 4.6 ). This induces a decrease in the respiratory function of the nose because in the area of the healthy respiratory mucous membrane there is hardly any flow left.

Surgical consequence: In functional rhinosurgery, large volumes should not be formed but instead a continuous slitlike space ought to be created.
Due to a decrease in cross-sectional area, the posterior cavum works like a nozzle ( Fig. 4.1a ). A similar function exists in the vestibule, and turbulence is thus decreased here also. This is important as now the air must perfuse the bronchial and alveolar pathway with the least flow resistance possible.
In the inspiratory direction, the choana is a convex opening ( Fig. 4.1a ). A convex opening leads to converging streamlines. This constricts the air streamline and prepares it for the following more narrow breathing pathway.
The nasopharynx is an almost rectangular bend ( Fig. 4.1a ) which redirects the air flowing out of the nose to the lower breathing pathways.
4.3.2 Expiration
In order to keep enough thermal energy and moisture available for the respiratory function of the nose, it is important that the nasal mucous membrane regains energy and moisture from the airflow, which is 37°C and has 100% humidity on expiration. Lacking this process of regaining energy, the nose could keep up its respiratory function only over a short time period. Accordingly, sufficient contact between airstream and mucous membrane is necessary during expiration as well.
In the expiratory direction ( Fig. 4.1b ), the posterior portion of the nose with the choana and the nasopharynx becomes the inflow area. It is equipped with similarly shaped functional elements as the inflow area in the inspiratory direction.
Due to its bending effect, the nasopharynx redirects the air emerging from the lower airways to the functional area. A nozzle effect is not required, as there is no constriction in the expiratory inflow area.
In this direction, the choana is a concave opening, which promotes diverging of streamlines.
With its increase in cross-sectional area, the posterior cavum has a diffuser effect and therefore leads to a slowdown in flow velocity and increases turbulence. With this, mucous membrane contact of the flowing particles is ensured.
The actual exchange of energy and moisture takes place in the area of turbinates, but now in the direction from the air to the mucous membrane.
For the expiration flow, the anterior cavum becomes a nozzle, which leads to a decrease in turbulence. With it, laminar flow can perfuse the narrowest opening, the ostium internum.
In expiration direction, the internal ostium is of concave shape, which promotes the converging of streamlines. This reduces the broad airstream to the smaller size of the cross-sectional area of the vestibule.
The bending effect of the vestibule causes the air to be blown out of the nose in a narrow beam in a lateral downward direction during expiration.
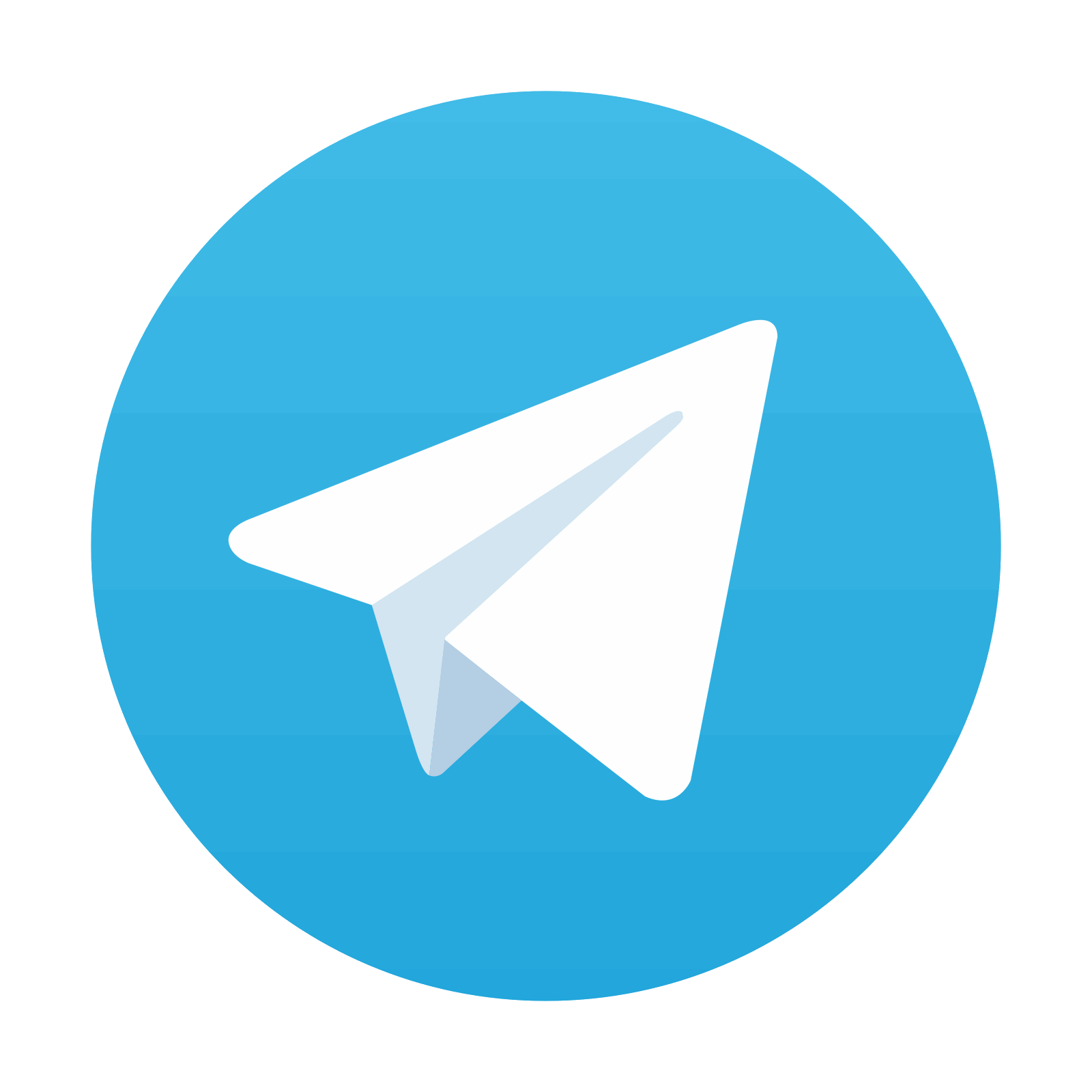
Stay updated, free articles. Join our Telegram channel

Full access? Get Clinical Tree
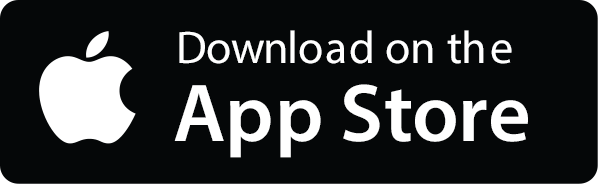
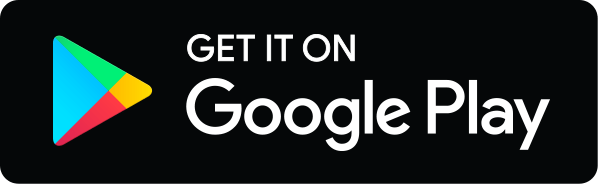
