Fluorescein angiography (FA) is a diagnostic technique that uses intravenous or oral fluorescein dye to allow the sequential visualization of blood flow simultaneously through retinal, choroidal, and iris tissue. Since its introduction, it has been an invaluable aid in the diagnosis, management, and treatment of chorioretinal diseases.
Key Features
- •
Intravenous or oral dose of fluorescein sodium dye administered.
- •
Sequential fundus photographs obtained by using a camera equipped with appropriate exciting and absorbing filters or light sources taking advantage of the inherent fluorescent properties of the dye.
- •
Reflected light captured on either film or as digital images.
- •
Interpretation of images critically dependent on an understanding of ocular anatomy in both health and disease.
- •
Indocyanine green dye, administered intravenously, can be an important adjunct to the diagnosis of chorioretinal disease.
Indocyanine green angiography (ICGA) is a diagnostic technique that exploits indocyanine green (ICG) dye’s infrared fluorescence and biochemical properties to adequately portray the characteristics of the choroidal circulation, aiding in the diagnosis of diseases affecting the choroid, such as idiopathic polypoidal choroidal vasculopathy, exudative age-related macular degeneration (AMD), and inflammatory diseases, among others.
Indocyanine green angiography (ICGA) is a diagnostic technique that exploits indocyanine green (ICG) dye’s infrared fluorescence and biochemical properties to adequately portray the characteristics of the choroidal circulation, aiding in the diagnosis of diseases affecting the choroid, such as idiopathic polypoidal choroidal vasculopathy, exudative age-related macular degeneration (AMD), and inflammatory diseases, among others.
Key Features
- •
Intravenous injection of indocyanine green dye.
- •
Serial photographs taken with digital imaging system to capture emission for dye.
- •
Interpretation of resultant images critically dependent on understanding of retinal and choroidal anatomy in health and disease.
Fundus autofluorescence (FAF) is a noninvasive retinal imaging modality used in clinical practice to map out density of lipofuscin within the retinal pigment epithelium (RPE).
Key Features
- •
Intrinsic fluorescence emitted by lipofuscin within the RPE after being excited with short to medium wavelength visible light.
- •
Photographs can be taken with a scanning laser ophthalmoscope (SLO) or standard/wide-field fundus camera.
- •
Interpretation of resultant images helps to detect and track changes in lipofuscin accumulation, which corresponds to the health and function of the RPE.
Fluorescein Angiography
Introduction
Fluorescein angiography (FA) relies on the special fluorescent property of sodium fluorescein (SF)—defined as the ability of certain molecules to emit light of longer wavelength when stimulated by light of shorter wavelength. After stimulation, electrons return to their base energy level, emitting energy in the form of electromagnetic waves producing visible light. The dye has a narrow spectrum of light absorption, with the maximum peak at 490 nm (485–500 nm, blue visible spectrum). Emission (fluorescence) occurs in the yellow-green spectrum with a wavelength of 530 nm (520–535 nm).
A stimulation source transmits light energy to the patient’s retina using either a flash/filter or laser in the 485–500 nm range. The energy is then either reflected back by the retina as blue light, or absorbed by the SF and emitted back as green light. A capturing device (camera) uses a green filter (520–535 nm) to selectively save the fluorescent image onto film or a digital surface.
Purpose of the Test
In normal individuals, the SF molecule freely crosses the wall of highly permeable capillaries (choriocapillaris) but remains within the lumen of retinal and larger choroidal vessels because a good percentage circulates through the blood unbound to plasma proteins. This makes FA the ideal study for evaluating retinal circulation, its vascular architecture, and the status of the inner and outer blood–retinal barrier. Information from the FA can also be used to study the choroidal circulation and retinal pigment epithelial (RPE) cells. Vascular diseases, such as diabetic retinopathy, central serous chorioretinopathy, venous occlusive disease, and choroidal neovascularization secondary to age-related macular degeneration (CNV-AMD), can be clearly demonstrated with FA. These images are used to select the appropriate therapeutic approach, guide treatment, and assess therapeutic results.
Properties of Sodium Fluorescein Dye
SF (sodium resorcinolphathalein) is a yellow-red, synthetic salt dye that is most commonly used to evaluate flow patterns of subterranean waters, as a cosmetic and pharmacological color, and as a labeling agent in protein research. It has a molecular weight of 376.7 kilodaltons (kDa). Once injected to the bloodstream, approximately 80% of the dye becomes bound to plasma proteins (particularly albumin), and the rest remains unbound. The dye is metabolized by the liver and kidneys and is eliminated in the urine within 24–36 hours of injection. Its most important property for ophthalmological purposes is its fluorescence. It has a narrow spectrum of absorption and excitation that makes the FA technique feasible (see “ Introduction ”). SF dye is readily available and produced commercially in aliquots of 2–3 mL of 25% or 5 mL of 10% sterile aqueous solution.
Procedure
A good quality FA is highly dependent on a high-resolution fundus camera, a skilled photographer, and a clear view to the retina.
The spherical refractive error of the patient is corrected by simultaneously focusing the cross-hairs in the eyepieces reticule and the fundus. The focusing wheel is used only for fine focus. Most cameras are equipped with a joystick to help align the camera to the patient’s eye. Proper alignment gives even illumination of the fundus, whereas misalignment results in peripheral and central artifacts in the images. This can be ameliorated with careful lateral movements of the joystick. Variable amounts of magnification can usually be selected, depending on the system, and this should be tailored to the pathology being examined.
Pharmacological mydriasis is usually required for most commercially available equipment, but there are a few that do not require it. Before starting the infusion of the dye, a set of baseline red-free images are taken using a green filter. Green light provides excellent contrast and enhances the visibility of the retinal vasculature and vitreous–retinal interface. It is particularly useful in assessing retinal hemorrhages, drusen, epiretinal membranes, and exudates.
The dye is typically injected in the antecubital vein with a 21-gauge butterfly needle in a rapid but controlled infusion (≈ 1 mL/sec) to maximize the contrast of the early filling phase of the angiography. Although there is no evidence of increased side effects when using higher concentrations of the dye, many practitioners prefer to use a smaller volume of a more concentrated solution. The two preferred doses are 2.5 mL of a 20% solution and 5 mL of a 10% solution. If the patient is a newborn or premature baby, a 10% solution at a dose of 0.1 mL/kg followed by an isotonic saline flush is recommended. Infusion of the dye can be done from the left or right antecubital vein without changing the times or image qualities. If the patient has undergone mastectomy with lymph node dissection, the dye should probably not be injected in the ipsilateral arm because of the risk of altered lymphatic flow.
Extravasation of the dye should be avoided, as infiltration is painful and may rarely lead to tissue necrosis. A timer is started after injection of the dye, and image acquisition should begin immediately to capture initial choroidal and retinal filling. Photographs are usually taken at 4-second intervals, beginning 15 seconds before injection and continuing with a tapered frequency for 10–20 minutes. However, the timing and the interval between exposures should be adjusted on the basis of the pathology that is being studied. For instance, a choroidal neovascular membrane leaks profusely early in the study; therefore, photographs should be taken with more frequency at the beginning of the study to capture the details of the membrane.
Complications
FA is an invasive test, and despite being deemed generally safe, it is not free of adverse reactions, ranging from mild to severe. Mild reactions are defined as transient and resolve spontaneously without treatment. Most commonly these are nausea (approximately 3%–15%), vomiting (up to 7%), sneezing, inadvertent arterial injection, and pruritus. Moderate adverse reactions resolve with medical intervention. These include urticaria, angioedema, syncope, thrombophlebitis, pyrexia, local tissue necrosis, and nerve palsy. Severe reactions require intensive intervention, and the patients may have poor recovery. These reactions include laryngeal edema, bronchospasm, anaphylaxis, hypotension, shock, myocardial infarction, pulmonary edema, hemolytic anemia, cardiac arrest, tonic-clonic seizures, and death. The incidence of adverse reactions has been described in the report on a multicenter, collaborative study ( Table 6.6.1 ). The overall incidence of complications is estimated to be 3%–20%. Although not considered a complication, the yellowing of skin, most commonly in fair-skinned individuals, may lead to photosensitivity, and patients should be cautioned about exposure to ultraviolet rays. Recently, a case of extensive jaundice following fluorescein was reported. Patients should also be advised about possible darkening of urine for 24–48 hours after the study.
Reaction | Incidence | |
---|---|---|
Mild | Nausea, vomiting sneezing | 0%–5% (based on 87% of respondents) |
Urticaria | 1 : 82 | |
Moderate | Syncope | 1 : 337 |
Other | 1 : 769 | |
Overall | 1 : 63 | |
Respiratory | 1 : 3800 | |
Cardiac | 1 : 5300 | |
Severe | Seizures | 1 : 13900 |
Death | 1 : 221781 | |
Overall | 1 : 1900 |
Previous efforts to relate the procedure technique, dye concentrations, and rate of infusion and volume with the incidence of adverse reactions have been inconclusive. Although in vivo skin tests remains the most reliable diagnostic tool for the diagnosis of immunoglobulin E–mediated allergy to SF (including severe cases of anaphylaxis), it is not particularly effective in predicting mild adverse reactions (ARs) because they are not attributable to immunological mechanisms. Special attention should be paid to patients with reported previous mild or moderate ARs during the study because the rate of recurrence is high (48%–68%), and the study should be avoided, if possible.
The American Academy of Ophthalmology Preferred Practice Patterns states that each angiographic facility should have in place an emergency protocol to minimize risk and manage complications. Regular stocking and updating of medications is needed, as well as regular training of photographers and supporting staff to recognize signs and symptoms of anaphylaxis. An emergency kit should be available on site and should include an airway bag, intravenous equipment, automated external defibrillator, oral or intramuscular antihistamines, and autoinjectors of epinephrine. The protocol should be posted in a prominent place and be visible to everybody.
Although package inserts from most brands of SF dye state that its use should be avoided during pregnancy, especially during the first trimester, data from several series and animal studies have not been able to identify a higher rate of birth abnormalities or complications (regardless of SF concentration: 10% or 25%). It is therefore reasonable to perform FA on pregnant patients when vision is threatened by potentially blinding diseases. Nonetheless, most clinicians prefer to wait until after delivery. Nursing mothers are discouraged from breastfeeding for at least 24–48 hours after FA.
Several technological advances have taken place since the introduction of film angiography:
- •
Confocal scanning laser ophthalmoscope (CSLO) as the energy source: The main benefit of switching to CSLO instead of a traditional cobalt blue flashbulb is that the exact laser wavelength can be selected to generate the peak emission of light of the SF dye. This means a significant increase of the signal-to-noise ratio in each examination. Despite the fact that the retina receives a higher emission of light energy with this modality, the toxic threshold is not exceeded because the energy is emitted only for 0.1–0.7 microseconds. This enables high-speed acquisition of images and short movies, allowing a dynamic evaluation of the blood flow through the retinal and choroidal vessels. The wavelength of the laser can be tuned or combined to acquire images with different dyes simultaneously (SF and indocyanine green). The procedure is more comfortable for the patient because there is no bright flash ( Fig. 6.6.1 ) .
Fig. 6.6.1
Fluorescein Angiography (FA) and Indocyanine Green Angiography (ICGA) Images Taken Simultaneously in the Same Patient.
(A) FA image. (B) ICGA image, in which, in addition to the normal fluorescence of the retinal vessels, the deep choroidal vessels are visualized.
(Courtesy Jans Fromow-Guerra, MD: Associate Professor. Asociación para Evitar la Ceguera en México, IAP. México DF.)
- •
The change of film to digital images: The development of high-resolution (high-definition [HD]) cameras along with computers with higher storage capacity has allowed digital equipment to mostly supplant traditional film equipment. Digital images can have a similar or greater resolution than that of a traditional film-based one (4096 × 2736 pixels). The coupling of CSLO with pinhole cameras effectively blocks scattered light as well as details outside the optical focus. As a result, greater detail in the capillary network becomes visible. And, finally, a digital image enables real-time correction of gain, exposure, and focus, as well as instant visualization, which means better image quality. It also makes the examination and manipulation of the images easier, allows for the rapid transmission of data by electronic means, and eliminates the need for physical storage space.
- •
Wide and ultra-wide angle of view: Traditionally, the standard angle of view was 30–50° with a 2.5 magnification, making the evaluation of peripheral retinal pathology difficult. Contact lens systems increased the angle of view to up to 160°. A new ultra-wide field system that uses a rotating ellipsoidal mirror with two conjugate focus points combined with a scanning laser ophthalmoscope creates an ellipsoidal surface capable of focusing light rays emanating from the peripheral retina, (up to 200°) ( Fig. 6.6.2 ). Ultra-wide field angiography has shown to be useful for identification and management of peripheral pathology in various diseases, including diabetes, sickle cell anemia, posterior/panuveitis, and pediatric retinal disease, among others.
Fig. 6.6.2
Example of Ultra-Wide Field Fluorescein Angiography (FA) Images (200° Angle View).
Mid-phase angiogram in a patient with proliferative diabetic retinopathy. Note the neovascularization elsewhere (NVE) along the distance inferotemporal arcades with surrounding areas of nonperfusion and mild leakage from the large vessels. Small areas of punctate hyperfluorescence represent multiple microaneurysms in all quadrants.
Interpretation of Results
Normal Fluorescein Angiogram
The dye first enters the eye in the short posterior ciliary arteries 10–15 seconds after injection in patients with normal circulation. The dye is then visualized in the choroid and optic nerve head. This initial filling is dependent on the cardiovascular condition and age of the patient as well as the speed of injection. The choroidal circulation is seen initially as the choroidal flush—a mottled and patchy fluorescence created as dye fills the choriocapillaris. The patchy appearance is created as separate lobules of the choriocapillaris fill sequentially. As dye leaks from the choriocapillaris during the early phases of the angiogram, Bruch’s membrane is stained, and choroidal vasculature detail is obscured. A cilioretinal artery is seen simultaneously with the fluorescence of the choroidal circulation in 10%–15% of patients.
One to three seconds after choroidal filling, the retinal circulation begins to fluoresce (at 11–18 seconds). The retinal arterial system should fill completely in about 1 second. The early arteriovenous phase is characterized by the passage of fluorescein dye through the central retinal arteries, the precapillary arterioles, and the capillaries, and the late arteriovenous phase is characterized by the passage of dye through the veins in a laminar pattern. During the late arteriovenous phase, maximal fluorescence of the arteries occurs, with early laminar filling of the veins. Laminar filling of veins is caused by the preferential concentration of unbound fluorescein along the vessel walls. Several factors are responsible for the laminar pattern of venous filling; these include the more rapid flow of plasma along the vessel wall as well as the higher concentration of erythrocytes in the central vascular lumen.
Maximal fluorescence is achieved in the juxtafoveal or perifoveal capillary network after 20–25 seconds. The normal capillary-free zone, or foveal avascular zone, is approximately 300–500 µm in diameter. A dark background to this capillary-free zone in the macula is created by blockage of choroidal fluorescence by both xanthophyll pigment and a high-density of RPE cells in the central macula. This phase of angiography has been termed the peak phase because maximal fluorescence of the capillaries and enhanced resolution of capillary detail occurs ( Fig. 6.6.3 ). The management of microvascular diseases of the retinal capillaries, such as diabetic macular edema, requires excellent peak phase imaging.

The first pass of fluorescein through the retinal and choroidal vasculature is complete after 30 seconds. The recirculation phases, characterized by intermittent mild fluorescence, follow. After approximately 10 minutes, both the retinal and choroidal circulations generally are devoid of fluorescein. Many normal anatomical structures continue to fluoresce during late angiography, such as the disc margin and optic nerve head. The staining of Bruch’s membrane, choroid, and sclera is more visible in patients who have lightly pigmented RPE.
Abnormal Fluorescein Angiography
The terms hypofluorescence and hyperfluorescence are used in the interpretation of fluorescein angiograms. Hypofluorescence is a reduction or absence of normal fluorescence ( Box 6.6.1 ), whereas hyperfluorescence is increased or abnormal fluorescence ( Box 6.6.2 ).
- •
Hypofluorescence: Hypofluorescence can be categorized into blockage (masking of fluorescence) or vascular filling defects. Blocked fluorescence can provide clues as to the level of the blocking material, such as vitreal, retinal, or subretinal. Only structures or material anterior to the area of fluorescence can block fluorescence. Blocked retinal fluorescence may be caused by any element that diminishes the visualization of the retina and its circulation ( Fig. 6.6.4 ). Blockage of retinal fluorescence also may localize the pathology to the inner retina. The retinal circulation is unique in that the large retinal vessels and precapillary first-order arterioles lie in the nerve fiber layer, whereas the capillaries and postcapillary venules are located in the inner nuclear layer. Flame-shaped hemorrhages are superficial and block all retinal vascular fluorescence, whereas deeper dot or blot hemorrhages (or intraretinal lipid) block capillary fluorescence but do not block larger superficial vessels. Fluorescence may also be blocked by melanin (scars, melanoma, nevus), lipofuscin deposits (Stargardt disease and Best disease), hemorrhage (diabetic retinopathy), and serosanguinous fluid beneath the RPE (CNV-AMD). Vascular filling defects produce hypofluorescence because of the reduced or absent perfusion of tissues. Retinal vascular filling defects can involve large-, medium-, or small-caliber vessels. Capillary nonperfusion manifests as vascular filling defects and is typically seen in common ischemic disease processes, such as diabetic retinopathy and venous occlusive disease ( Fig. 6.6.5 ). Choroidal vascular filling defects are more difficult to visualize because the native RPE prevents adequate visualization of the choroidal circulation. In general, occlusive diseases that involve isolated, larger choroidal vessels manifest as sectoral, wedge-shaped areas of hypofluorescence. Systemic diseases, including malignant hypertension, toxemia of pregnancy, giant cell arteritis, and lupus choroidopathy, produce zones of hypofluorescence secondary to focal choroidal nonperfusion. Vascular filling defects of the optic nerve head may be noted by fluorescein angiography. Ischemic optic neuropathy manifests as sectoral or complete optic disc hypofluorescence, whereas other atrophic or hereditary anomalies of the optic nerve head have diffuse hypofluorescence.
Fig. 6.6.4
Blockage.
Fluorescein angiography (FA) image from a patient with idiopathic macular telangiectasia. In this late-phase angiogram, intraretinal pigment plaques are blocking (arrow) the background choroidal fluorescence. Note the significant leakage from the telangiectatic vessels worse temporally as well as staining of the scleral crescent around the optic nerve.
(Courtesy Michael Bono, CRA, COT Rocky Mountains Lions Eye Institute, Denver, CO.)
Fig. 6.6.5
Vascular Filling Defect.
Ultra-wide field fluorescein angiography (FA) image of an inferior hemiretinal vein occlusion in the late phase. There are areas of significant nonperfusion with a large area noted temporal macula (arrow). Note the blockage from heme, leakage from large and small vessels in the macula and periphery.
(Courtesy Hoang Nguyen, COT Rocky Mountains Lions Eye Institute, Denver, CO.)
- •
Hyperfluorescence : Hyperfluorescence is defined as an abnormal presence of fluorescence or an increase in normal fluorescence in the FA. It can be secondary to increased transmission of choroidal fluorescence caused by a window defect created by an area with a decreased or absent RPE that allows a clear view of the underlying choroidal fluorescence ( Fig. 6.6.6 ). The most frequent cause of hyperfluorescence is leakage of dye from the intravascular space into the extravascular space. In this case a localized, diffuse hyperfluorescent spot increases in both size and intensity as the study progresses ( Fig. 6.6.7 ). When the dye leaks into an anatomical space (cysts, subretinal space, sub-RPE space) it is called pooling. In this case, the boundaries of the hyperfluorescence are more defined, and the speed of appearance depends mostly on the cause ( Fig. 6.6.8 ). Finally, staining refers to the deposition of dye within involved tissue and occurs in both normal (optic nerve and sclera) and pathological states (drusen, disciform scars).
Fig. 6.6.6
Window Defect.
Fluorescein angiography (FA) image from a patient with an advanced case of Stargardt disease. The picture shows increasing fluorescence caused by atrophy, noted since the early phases of the angiogram.
(Courtesy Valentina Franco-Cardenas, MD: International Retina Fellow, University of California Los Angeles (UCLA).)
Fig. 6.6.7
Leakage.
Fluorescein angiography (FA) image from a patient with proliferative sickle cell retinopathy. Ultra-wide field angiogram demonstrates progressive leakage of dye from the three peripheral sea-fan neovascularization temporally. Note the peripheral nonperfusion (arrow) as well as the blockage from old subhyaloid heme (chevron) below the neovascularization (A–C).
Fig. 6.6.8
Pooling .
Color picture and fluorescein angiography (FA) sequence from a patient with central serous chorioretinopathy. (A) & (C), Small arrowheads delineate an area of neurosensory detachment of the macula with pooling of the dye in the late phase of the study. (B) & (C), Large white arrows indicate areas of retinal pigment epithelium leakage of fluorescein.
(Courtesy Valentina Franco-Cardenas, MD: International Retina Fellow, University of California Los Angeles [UCLA].)
Blocked Retinal Fluorescence
- •
Media opacity
- •
Vitreous opacification (hemorrhage, asteroids hyalosis, vitritis)
- •
Subhyaloid hemorrhage.
- •
Intraretinal pathology (hemorrhage [vein occlusion], edema)
Blocked Choroidal Fluorescence
- •
All entities that cause blockade retinal fluorescence.
- •
Outer retinal pathology (lipid, hemorrhage, xanthophyll)
- •
Subretinal pathology (hemorrhage, lipid, melanin, lipofuscin, fibrin, inflammatory material)
- •
Subretinal pigment epithelium pathology (hemorrhage)
- •
Choroidal pathology (nevus, melanoma)
Vascular Filling Defects
Retina
- •
Occlusion or delayed perfusion
- •
Central or branch artery occlusion
- •
Capillary nonperfusion (diabetes, vein occlusion, radiation, etc.)
- •
Atrophy or absence of retinal vessels
Choroid
- •
Occlusion of large choroidal vessels or choriocapillaris (sectoral infarct, malignant hypertension, toxemia, lupus, choroidopathy, renal disease)
- •
Atrophy or absence of choroidal vessels or choriocapillaris (choroideremia, acute multifocal placoid pigment epitheliopathy)
Optic Nerve
- •
Occlusion (ischemic optic neuropathy)
- •
Atrophy or absence of tissue (coloboma, optic nerve pit, optic nerve hypoplasia, optic atrophy)
Pseudo-fluorescence
Autofluorescence
Transmitted Fluorescence
- •
Geographic atrophy
- •
“Bull’s eye” maculopathy
- •
Macular hole
- •
Atrophic chorioretinal scar
- •
Drusen
Abnormal vessels
R etina
- •
Angioma; Wyburn–Mason syndrome
- •
Cavernous hemangioma
- •
Vascular tumor
- •
Retinoblastoma
C horoid
- •
Melanoma
- •
Choroidal neovascularization
- •
Choroidal hemangioma
O ptic N erve
- •
Peripapillary vascular loops
Leakage
R etinal V essels
- •
Venous occlusive disease
- •
Frosted angiitis
- •
Phlebitis
N eovascularization
- •
Diabetes retinopathy
- •
Radiation retinopathy
- •
Sickle cell retinopathy
Pooling:
Neurosensory detachment
- •
Central serous chorioretinopathy
- •
Optic nerve pit
- •
Best disease
Subretinal neovascularization
Retinal pigment epithelium detachment
- •
Serous
- •
Fibrovascular
Staining
- •
Staphyloma
- •
Disc
- •
Sclera
- •
Chorioretinal scar
Introduction
Fluorescein angiography (FA) relies on the special fluorescent property of sodium fluorescein (SF)—defined as the ability of certain molecules to emit light of longer wavelength when stimulated by light of shorter wavelength. After stimulation, electrons return to their base energy level, emitting energy in the form of electromagnetic waves producing visible light. The dye has a narrow spectrum of light absorption, with the maximum peak at 490 nm (485–500 nm, blue visible spectrum). Emission (fluorescence) occurs in the yellow-green spectrum with a wavelength of 530 nm (520–535 nm).
A stimulation source transmits light energy to the patient’s retina using either a flash/filter or laser in the 485–500 nm range. The energy is then either reflected back by the retina as blue light, or absorbed by the SF and emitted back as green light. A capturing device (camera) uses a green filter (520–535 nm) to selectively save the fluorescent image onto film or a digital surface.
Purpose of the Test
In normal individuals, the SF molecule freely crosses the wall of highly permeable capillaries (choriocapillaris) but remains within the lumen of retinal and larger choroidal vessels because a good percentage circulates through the blood unbound to plasma proteins. This makes FA the ideal study for evaluating retinal circulation, its vascular architecture, and the status of the inner and outer blood–retinal barrier. Information from the FA can also be used to study the choroidal circulation and retinal pigment epithelial (RPE) cells. Vascular diseases, such as diabetic retinopathy, central serous chorioretinopathy, venous occlusive disease, and choroidal neovascularization secondary to age-related macular degeneration (CNV-AMD), can be clearly demonstrated with FA. These images are used to select the appropriate therapeutic approach, guide treatment, and assess therapeutic results.
Properties of Sodium Fluorescein Dye
SF (sodium resorcinolphathalein) is a yellow-red, synthetic salt dye that is most commonly used to evaluate flow patterns of subterranean waters, as a cosmetic and pharmacological color, and as a labeling agent in protein research. It has a molecular weight of 376.7 kilodaltons (kDa). Once injected to the bloodstream, approximately 80% of the dye becomes bound to plasma proteins (particularly albumin), and the rest remains unbound. The dye is metabolized by the liver and kidneys and is eliminated in the urine within 24–36 hours of injection. Its most important property for ophthalmological purposes is its fluorescence. It has a narrow spectrum of absorption and excitation that makes the FA technique feasible (see “ Introduction ”). SF dye is readily available and produced commercially in aliquots of 2–3 mL of 25% or 5 mL of 10% sterile aqueous solution.
Procedure
A good quality FA is highly dependent on a high-resolution fundus camera, a skilled photographer, and a clear view to the retina.
The spherical refractive error of the patient is corrected by simultaneously focusing the cross-hairs in the eyepieces reticule and the fundus. The focusing wheel is used only for fine focus. Most cameras are equipped with a joystick to help align the camera to the patient’s eye. Proper alignment gives even illumination of the fundus, whereas misalignment results in peripheral and central artifacts in the images. This can be ameliorated with careful lateral movements of the joystick. Variable amounts of magnification can usually be selected, depending on the system, and this should be tailored to the pathology being examined.
Pharmacological mydriasis is usually required for most commercially available equipment, but there are a few that do not require it. Before starting the infusion of the dye, a set of baseline red-free images are taken using a green filter. Green light provides excellent contrast and enhances the visibility of the retinal vasculature and vitreous–retinal interface. It is particularly useful in assessing retinal hemorrhages, drusen, epiretinal membranes, and exudates.
The dye is typically injected in the antecubital vein with a 21-gauge butterfly needle in a rapid but controlled infusion (≈ 1 mL/sec) to maximize the contrast of the early filling phase of the angiography. Although there is no evidence of increased side effects when using higher concentrations of the dye, many practitioners prefer to use a smaller volume of a more concentrated solution. The two preferred doses are 2.5 mL of a 20% solution and 5 mL of a 10% solution. If the patient is a newborn or premature baby, a 10% solution at a dose of 0.1 mL/kg followed by an isotonic saline flush is recommended. Infusion of the dye can be done from the left or right antecubital vein without changing the times or image qualities. If the patient has undergone mastectomy with lymph node dissection, the dye should probably not be injected in the ipsilateral arm because of the risk of altered lymphatic flow.
Extravasation of the dye should be avoided, as infiltration is painful and may rarely lead to tissue necrosis. A timer is started after injection of the dye, and image acquisition should begin immediately to capture initial choroidal and retinal filling. Photographs are usually taken at 4-second intervals, beginning 15 seconds before injection and continuing with a tapered frequency for 10–20 minutes. However, the timing and the interval between exposures should be adjusted on the basis of the pathology that is being studied. For instance, a choroidal neovascular membrane leaks profusely early in the study; therefore, photographs should be taken with more frequency at the beginning of the study to capture the details of the membrane.
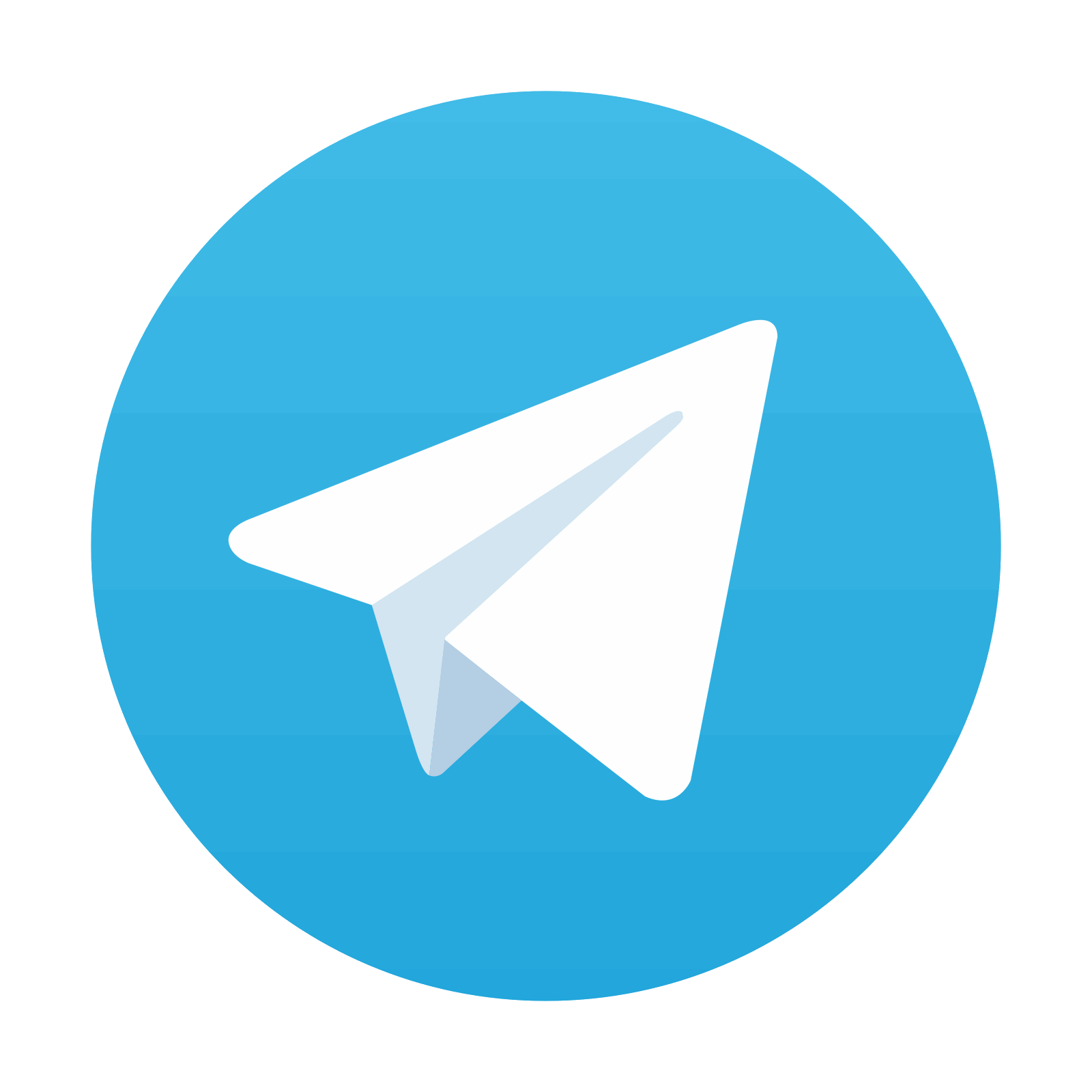
Stay updated, free articles. Join our Telegram channel

Full access? Get Clinical Tree
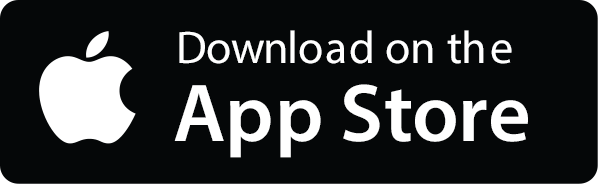
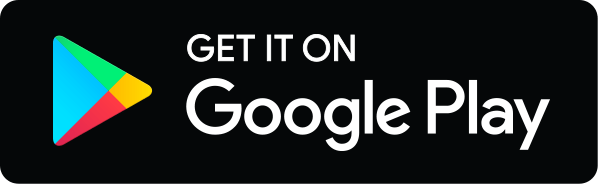