KEY CONCEPTS
- •
Moderate and advanced stages of keratoconus are readily recognized, but the identification of milder or subclinical forms of this disease sometimes remains challenging.
- •
The advent of refractive surgery and the development of new treatment modalities for patients with corneal ectasia increased the need for the diagnosis and characterization of ectatic corneal diseases.
- •
Multimodal refractive imaging including Placido-disk based corneal topography, Scheimpflug corneal tomography, segmental tomography with epithelial thickness, and Bowman’s layer characterization with optical coherence tomography (OCT) and very-high-frequency ultrasound (VHF-US) enhances the characterization of corneal shape and structure.
- •
Clinical corneal biomechanical assessment further augments the ability to diagnose keratoconus and ectatic corneal diseases.
- •
Artificial intelligence has been used to develop new indices that improve the diagnostics for ectatic corneal disorders.
- •
The quest for diagnosis evolved to the characterization of the intrinsic susceptibility for corneal ectasia development and progression.
Introduction
Corneal biomechanics has emerged as an important topic for research and development in ophthalmology because of the many potential applications. , The need to enhance diagnosis to recognize milder forms of the keratoconus (KC) and ectatic corneal diseases (ECDs) and identify the intrinsic predisposition for ectasia progression has gained significance because of refractive surgery and the development of new treatment modalities.
There has been a paradigm change associated with the management of ECDs, which was restricted to spectacles, rigid contact lenses, or penetrating keratoplasty. The advent of novel treatment modalities, such as corneal collagen cross-linking (CXL) and intrastromal corneal ring segments (ICRS), both with an underlying biomechanical mechanism, has established diverging situations on when, why, and how to proceed with surgery on these patients. Such considerations demonstrate the requirement for individualized treatment planning, which should comprise a precise evaluation of patient needs, advanced imaging with advanced geometric characterization, biomechanical assessment, and environmental factors such eye rubbing.
Clinical Applications of Corneal Biomechanics
Knowledge of corneal biomechanics provides a substantial contribution to the diagnosis, staging, and prognosis of KC and other corneal ectatic conditions. , , It has also become important for the detection of earlier stages of ectatic disease (subclinical KC), boosted by the development of new treatment modalities such as CXL and ICRS. In the setting of refractive surgery, the investigation of corneal biomechanical properties has become a critical part of the screening process to recognize patients at higher risk of developing iatrogenic ectasia after laser vision correction (LVC), along with improving the predictability and efficacy of these elective procedures.
Biomechanical principles have been applied in several other clinical conditions, including correcting intraocular pressure (IOP) measurements, , planning and following up corneal collagen CXL treatments, and other treatment modalities such as ICRS, which can be combined in different approaches to manage KC patients. , ,
The introduction of Placido-disk based corneal topography increased the ability to identify ECD in earlier stages, even before the development of clinical signs or visual symptoms. , However, the limitations of this technology are shown by the occurrence of post-refractive keratectasia despite normal anterior curvature maps and cases with suspicious topographic patterns that proceeded with LCV, based on advanced corneal imaging, resulting in documented stable outcomes.
Further developments in corneal diagnostic imaging technologies allowed for segmental or layered tomographic three-dimensional (3D) characterization with epithelial , and Bowman’s layer regularity characterization and thickness mapping. While corneal epithelial thickness was initially assessed by digital very-high-frequency ultrasound (VHF-US), advances in corneal and anterior segment optical coherence tomography (OCT) allowed for such evaluation in a rapid examination without the necessity of a water bath. The assessment of the corneal surface evolved into 3D corneal tomography, with 3D reconstruction of front and back corneal surfaces with a full-thickness map. Studies have demonstrated higher sensitivity of the tomographic approach to identify subclinical (or fruste) disorder in eyes with relatively normal topographic maps from patients with the fellow eye showing clinical ectatic disease. , The association with ocular biometry and ocular wavefront measurements can further improve the detection ability.
The current understanding is that the pathophysiology of ectatic diseases is related to a primary biomechanical abnormality, with architecture and morphologic instability as secondary events. , Beyond shape analysis, clinical biomechanical assessment has been promising as the ultimate tool (before genetic analysis is available) for enhancing the overall accuracy for identifying mild forms of ECDs. ,
Corneal imaging should evolve further to integrate devices such as Scheimpflug tomography, OCT segmental tomography, ocular wavefront and biometry, and corneal biomechanical assessment. Considering the vast amount of generated data, the conscious use of artificial intelligence plays a major role in taking greater advantage of such information for clinical decisions.
Chronic Biomechanical Failure of the Cornea (Keratectasia)
Chronic biomechanical failure occurs when a tissue fatigues over time due to chronic low-grade stress. Susceptibility to fatigue is a common phenomenon in biological tissues, which may fail in response to stress loads in a time-dependent fashion. However, a healthy balance exists in the cornea between extracellular matrix damage and subsequent wound repair, which prevents chronic biomechanical failure of the stroma proper via keratocyte-directed repair processes.
In composite structures, two types of chronic biomechanical failure occur during fatigue: delamination and interfiber fracture. Delamination is the separation of two adjacent plies (lamellae) from each other, whereas the interfiber split is the development of a full-thickness interfibrillar matrix crack in a single ply. When this chronic biomechanical failure process progresses, the structure usually thins to measurable or detectable levels, and fibril fracture—defined as a direct, acute full-thickness breakage of collagen fibrils in a ply—may occur in Bowman’s layer and also in the Descemet membrane. , Bulging or protrusion usually occurs after thinning has already started but may progress in severity; this is particularly important for identification of the earliest changes related to ectasia.
Keratectasia therefore is an outcome of a nonspecific chronic biomechanical failure response by the cornea to various stresses. Some degree of slippage related to delamination and interfiber fracture can occur as part of a partial biomechanical response, but may not proceed to full-blown keratectasia. Each cornea has a unique ability to withstand internal stress, but it may be overwhelmed by additional stressors, such as eye rubbing, increasing IOP level, corneal-weakening procedures (Laser-Assisted in Situ Keratomileusis (LASIK) or photorefractive keratectomy surgery), and, possibly, external nocturnal eye pressure, as occurs with face-down positioning in sleep apnea. If progressive chronic biomechanical failure ensues, structural failure of the cornea may develop, with loss of shape. Loss of corneal shape ultimately may lead to reduced refractive function and reduced optical clarity.
KC is the most common ECD. By definition, KC is a bilateral (but typically asymmetric) and progressive corneal dystrophy. The most accepted hypothesis is that it occurs as a combination of a genetic predilection and environmental influences, such as eye rubbing and ocular trauma. , There is a consensus that KC is indeed a bilateral disease, but also that secondary ectasia may be caused by a purely mechanical process, such as after refractive surgery with tissue removal or due to excessive eye rubbing, and this may occur in only one eye.
Ocular Response Analyzer
In vivo evaluation of corneal biomechanical response first became accessible with the launch of the Ocular Response Analyzer (ORA; Reichert Ophthalmic Instruments, Buffalo, NY) in 2005. , The ORA is a noncontact tonometer (NCT) with a collimated air puff to indent a central 3 to 6 mm area of the apical cornea. A sophisticated electro-optical system monitors the bidirectional movement of the cornea across its reflection of an infrared beam. , , After the air pulse is triggered, the cornea deforms in an inward direction (ingoing phase), passing through a first applanation instant when the pressure (P1) is recorded. At first applanation, the air pump obtains a signal to shut off; the inertia in the piston permits the pressure to continue to grow so that the air pulse has a Gaussian configuration. The peak of the air pressure pulse is influenced by P1, making it a key parameter for each ORA measurement. As the air pressure continues to grow, the cornea adopts a concave shape. The outgoing phase is initiated as the air pressure declines, permitting the cornea to return to its original shape progressively. During the outgoing phase, the cornea passes through a second applanation, when the pressure of the air pulse (P2) is recorded. The pressure-derived parameters generated by the standard ORA software are corneal hysteresis (CH) and corneal resistance factor (CRF; Fig. 6.1 ). CH is the difference between the P1 and P2 values, whereas CRF is calculated according to the formula:

a[P1 – 0.7P2] + d
where a and d are calibration and regression constants, and the P2 coefficient of 0.7 was empirically determined to maximize correlation with central corneal thickness. ,
Although studies have reported CH and CRF to be lower in KC compared with healthy corneas, a substantial overlap in the distributions of both parameters was detected so that the sensitivity and specificity for KC diagnosis are quite weak. Additional research found more precise ectasia identification when examining the ORA waveform signal and using new parameters related to the deformation response of the cornea during the NCT measurement. Fig. 6.2 demonstrates the importance of evaluating the waveform signal when examining corneal properties in a 51-year-old patient who underwent bilateral LASIK. These properties were determined with the ORA, and it detects a nonectatic post-LASIK right eye and an ectatic post-LASIK left eye. It is important to note that both eyes had similar hysteresis but dramatically different signal characteristics.

More recently, the integration of these new parameters with tomographic data demonstrated improved accuracy in the detection of mild or early ectatic disease. Moreover, the waveform-derived parameters were found to document corneal biomechanical changes after CXL procedures in KC, while CH and CRF did not detect significant differences.
The Corvis ST
The Corvis ST (Oculus, Wetzlar, Germany) is also an NCT that includes an ultra-high-speed (UHS) Scheimpflug camera for pachymetry and biomechanical assessment of the cornea. During measurement, similar to what happens in the ORA examination, the cornea deforms inward and outward while passing through two applanation moments. Nevertheless, the Corvis ST has two essential differences from the ORA. First, in place of using the reflection of the infrared beam to monitor the distortion of the cornea, it utilizes a UHS Scheimpflug camera that acquires 140 horizontal 8-mm frames throughout 33 ms. This approach allows a more comprehensive assessment of the deformation process in two dimensions. Also, unlike the ORA, the Corvis ST yields a fixed maximal peak pressure for the air puff in every examination.
The Corvis ST calculates corneal deformation parameters based on the dynamic inspection of the corneal response ( Table 6.1 ). By way of air pressure, the cornea begins to deform in the backward direction. Whole eye motion is instantaneously initiated with a slow linear increase in the same backward direction and then increases just after the cornea reaches maximum deformation. Dynamic corneal response (DCR) parameters thereby either include or subtract the whole eye motion. The parameters described as “deformation” are those in which entire eye motion is included, while the “deflection” parameters take into account and subtract the backward motion of the eye. The deformation amplitude (DA) indicates the displacement of the corneal apex in the anterior-posterior direction, and the maximum value is determined at the highest concavity (HC) instant. The DA ratio 1 or 2 mm is the central deformation divided by an average of the deformation 1 to 2 mm at either side of the center, with a maximum value near the first applanation. Applanation lengths (AL) and corneal velocities (CVel) are recorded during ingoing and outgoing phases. The radius of curvature at the highest concavity (curvature radius HC) is also documented, and the integrated inverse radius is the reciprocal of the radius (analogous to curvature) during the concave state of the cornea. It should be noted that a greater concave radius (lower concave curvature) is associated with higher resistance to deform or a stiffer cornea. Thus the higher the integrated inverse radius and maximum inverse radius (greater concave curvature), the less resistance to deformation and lower corneal stiffness. Corneal thickness, the standard “Goldmann-correlated” IOP, and a biomechanically corrected IOP (bIOP) are recorded as well. ,
Corvis ST Parameters | |||
---|---|---|---|
First applanation | The first applanation of the cornea during the air puff (in milliseconds). The length of the applanation at this instant is shown in parenthesis (in millimeters). | ||
Highest concavity | The moment that the cornea assumes its maximum concavity during the air puff (in milliseconds). The length of the distance between the two peaks of the cornea at this instant appears in parenthesis (in millimeters). | ||
Second applanation | The second applanation of the cornea during the air puff (in milliseconds). The length of the applanation at this moment appears in parenthesis (in millimeters). | ||
Maximum deformation | The amount (in millimeters) of the maximum cornea deformation during the air puff. | ||
Wing distance | The length of the distance between the two peaks of the cornea at this moment (in millimeters). | ||
Maximum velocity (in) | Maximum velocity during the ingoing phase (in meters per second). | ||
Maximum velocity (out) | The maximum velocity during the outgoing phase (in meters per second). | ||
Curvature radius, normal | The cornea in its natural-state radius of curvature (in millimeters). | ||
Curvature radius, highest concavity | The cornea radius of curvature at the time of maximum concavity during the air puff (in millimeters). | ||
Cornea thickness | Measurement of the corneal thickness (in millimeters). | ||
Integrated inverse radius | The inverse of the radius of curvature during the concave phase of the deformation. | ||
Deformation amplitude ratio 1 or 2 mm | The central deformation divided by an average of the deformation 1 or 2 mm at either side of the center with a maximum value just prior to first applanation. | ||
IOP | Measurement of the intraocular pressure (in millimeters of mercury [mmHg]). | ||
bIOP | Biomechanically corrected IOP. |
The initial generation measurement parameters of the Corvis ST offered a performance analogous to that obtained by the pressure-derived ORA data for discriminating healthy and KC eyes. , However, the more substantial details of the DCR from the Scheimpflug camera enabled the development of new parameters that are less influenced by IOP than the original DCR parameters, which revealed a greater capacity to detect the onset of ectatic disease. The parameters which are indicative of corneal stiffness relate to the shape of the deformation, rather than the depth.
In 2014 a multicenter international investigation group was created to increase knowledge about Corvis ST technology with a distinct focus on the examination of ECD. , , One of the outcomes of this collaborative work was the Vinciguerra screening report, which provides correlations of normative values and bIOP. It was established throughout a limited-element parametric study that uses central cornea thickness and age in addition to deformation response parameters to decrease the effect of stiffness on IOP estimates. , Fig. 6.3 shows the Vinciguerra screening report and Fig. 6.4 shows DCR of a 16-year-old patient with forme fruste of KC (FFKC).

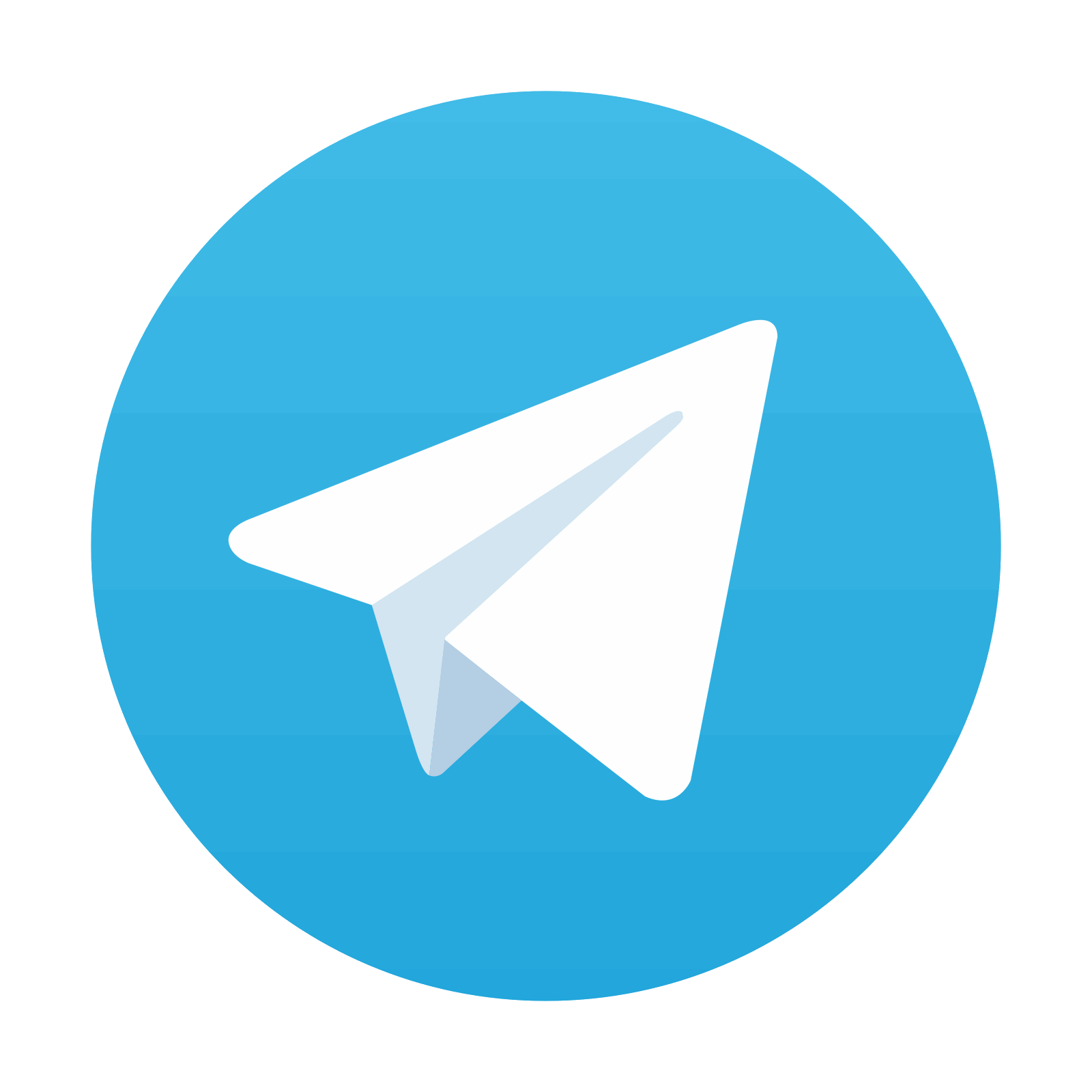
Stay updated, free articles. Join our Telegram channel

Full access? Get Clinical Tree
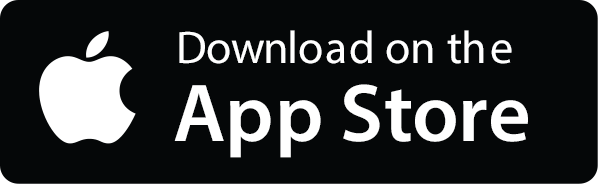
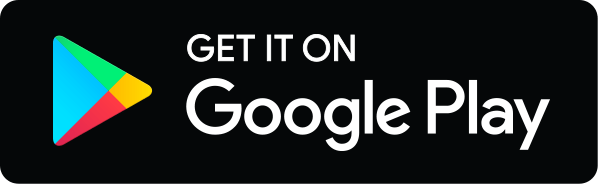
