Purpose
To assess the repeatability of axial biometry of the entire eye using ultra-long scan depth optical coherence tomography (OCT) and to investigate the agreement with IOLMaster measurements (Carl Zeiss Meditec).
Design
Prospective, observational case series.
Methods
There were 37 adult subjects enrolled in group 1 and 12 adult subjects enrolled in group 2. Using ultra-long scan depth OCT, the left eyes of these groups were measured in 2 separate sessions. The images were processed by a manual method and custom-developed automatic software. A model eye was imaged for verification. The subjects in group 2 were imaged using ultra-long scan depth OCT and using the IOLMaster for axial length measurement comparison.
Results
All measured parameters of the model eye matched the geometric parameters. In group 1, there were no significant differences in all measured parameters using automatic and manual segmentation methods ( P > .05, paired t test). The percentage of coefficient of repeatability of segments ranged from 0.3% to 3.9%. The corresponding interclass correlation coefficients ranged from 0.946 to 0.999. The correlation between the results using automatic and manual segmentation methods appeared to be strong ( R 2 = 0.999; P < .05). In group 2, the axial length of the eye measured by the IOLMaster matched the results obtained by ultra-long scan depth OCT with the automatic method ( R 1 2 = 0.987; P < .05) and the manual method ( R 2 2 = 0.988; P < .05).
Conclusions
Automatic axial biometry using ultra-long scan depth OCT successfully measured each segment of the entire eye with good repeatability. With further development of automatic segmentation, ultra-long scan depth OCT seems to be a promising tool in the axial biometry of the entire eye.
Accurate, rapid, and safe axial biometry of the entire eye, including measurements of axial length (AL) and crystalline lens thickness, is important for the power calculation of intraocular lens (IOL) and refractive error research. Many diseases, such as myopia, hyperopia, and glaucoma, can affect the axial biometry of the entire eye. Accommodation induces the dimensional changes of the anterior segment, resulting in altered segments in the eye. Traditional methods for axial biometry include ultrasound and optical low-coherence reflectometry-based devices, such as the IOLMaster (Carl Zeiss Meditec, Dublin, California, USA) and the Lenstar (Haag-Streit USA, Mason, Ohio, USA). These methods use a single axial measurement like the A-scan. Real-time imaging may be difficult with these traditional methods. With advances in optical coherence tomography (OCT), it is now possible to image the entire eye in 2 or 3 dimensions. With the advantages of high-speed scanning and high resolution, full-eye axial biometry may provide an alternative method to axial biometry. However, the precision of full-eye biometry remains untested. The goal of the present study was to determine the repeatability of axial biometry of the entire eye using ultra-long scan depth OCT. Also, we tested and validated our custom software for processing the ultra-long scan depth OCT images and providing axial biometry data of entire eye.
Methods
This protocol was approved prospectively by the Institutional Review Board for Human Research at the University of Miami. The study was in accordance with Health Insurance Portability and Accountability Act regulations. All subjects were treated in accordance with the tenets of the Declaration of Helsinki and gave written informed consent to participate in this study after they were informed about the nature, risks, and possible adverse consequences of the procedure. In this prospective study, there were 37 adult subjects (17 men and 20 women) enrolled in group 1 for ultra-long scan depth OCT measurements. Their mean age was 36.0 ± 14.4 years (range, 19 to 76 years) and their mean refraction was −2.8 ± 1.9 diopters (D; range, +2.00 to −7.00 D). In addition, there were 12 adult subjects (6 men and 6 women) from the group 1 who were also enrolled in group 2 for measurement by ultra-long scan depth OCT and IOLMaster. Their mean age was 35.2 ± 1.9 years (range, 26 to 48 years) and their mean refraction was −2.50 ± 1.9 D (range, −0.25 to −7.00 D). All subjects were recruited from the University of Miami. Exclusion criteria were anterior or posterior segment pathologic features; systemic disease; or history of laser treatment, trauma, or eye surgery.
Subjects in group 1 were imaged twice with ultra-long scan depth OCT. The parameters included central corneal thickness (CCT), anterior chamber depth (ACD), lens thickness (LT), vitreous length (VL), and AL. Subjects in group 2 were imaged with ultra-long scan depth OCT and the IOLMaster (Carl Zeiss Meditec, Dublin, California, USA) for the AL measurement. The measurements with both instruments were obtained twice at 1 visit. All measurements were performed on the left eyes. OCT imaging was conducted by the same researcher (Y.S.).
The ultra-long scan depth OCT system was improved from our previous prototype of long scan depth OCT by adding a switchable reference arm for improving the scan depth range. The improvement included the implementation of a complementary metal–oxide–semiconductor camera and a 4-mirror switchable reference arm for enhancing the scan speed and scan depth. The system consisted of 5 key elements: a light source, reference arm, sample arm, spectrometer, and computer. The light source was a superluminescent diode (IPSDD0808; InPhenix, Livermore, California, USA). Its center wavelength was 840 nm and the full width at half of the maximum bandwidth was 50 nm. The measured axial resolution was 7.7 μm. The measured scan depth was 12.57 mm. The power of incident light on the corneal surface of the human eye was 1.25 mW, which was less than the American National Standards Institute Z136.1 cutoff value for safety. The beam was split into the sample arm and the reference arm with a 50:50 fiber coupler. From information derived from our previous prototypes, we incorporated a special switchable reference arm in the OCT system to increase the signal-to-noise ratio and the effective imaging depth. There were 4 mirrors in the reference arm. A galvanometer, controlled by a computer, turned the light among the 4 mirrors in the reference arm, which increased the scan depth. This approach also was used in scanning the anterior segment and retina by Ruggeri and associates, who used a 3-mirror switchable reference arm. In our prototype, the 2 images from the first 2 mirrors were used to scan the anterior segment with the 0-delay lines placed at the top of the cornea and the posterior surface of the crystalline lens, similar to the setup used in our previous study. Overlaying these 2 images resulted in the enhancement of image quality. The third and fourth mirrors were set to scan the vitreous and retina. The optical path difference between first mirror and third mirror was 25.14 mm. The optical path difference between third mirror and fourth mirror was 12.57 mm. The total scan depth was 37.71 mm, which was sufficient to image the entire eye without gaps ( Figure 1 ). The sample arm was mounted on a modified slit-lamp microscope. The x-y galvanometer pair scanned the horizontal and the vertical meridians. The target was a white Snellen letter E on a black background displayed by the liquid crystal display screen, which was 10 cm from the tested eye. The spectrometer comprised 4 parts: a collimating lens ( f = 50 mm; OZ Optics, Ottawa, Canada), a 1800-lines/mm volume holography transmission grating, an image enlargement lens with a focal length of 240 mm ( f = 240 mm; Schneider Optice, Hauppauge, New York, USA), and a line array complementary metal–oxide–semiconductor camera with a high scan speed of up to 70,000 A-lines/second (Basler Sprint spL4096-140k; Basler AG, Ahrensburg, Germany). We set the exposure time at 44 μs and the scan speed to 17,500 A-lines/second to scan the entire eye. The image size obtained with each mirror was 2048 × 4096 pixels per scan segment, and the reconstructed image was 2048 × 12,288 pixels.
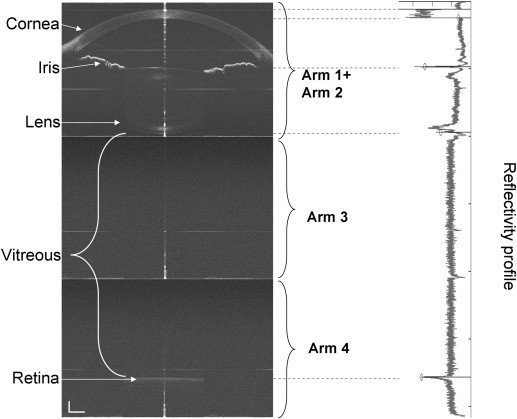
One model eye (OEMI-7; Ocular Instruments, Inc, Bellevue, Washington, USA; Figure 2 ) was imaged with ultra-long scan depth OCT to validate OCT full-eye biometry. The model eye was made from polymethyl methacrylate with a cornea, crystalline lens, and retina. The anterior chamber and vitreous chamber were filled with saline solution. The anterior segment, included cornea, anterior chamber, and crystalline lens, was detachable from the posterior segment. The geometry of the model eye was measured using a digital caliper (Tresna, Guilin Guanglu Measuring Instrument Co Ltd, Guangxi Province, China) and a digital thickness gauge (ID-C1012EBS; Mitutoyo Corp, Takatsu-ku, Kawasaki, Kanagawa, Japan). CCT (A in Figure 2 , Right) and the length from the anterior of the cornea to the posterior of the crystalline lens (C in Figure 2 , Right) were measured using the digital thickness gauge, LT (B in Figure 2 , Right) was measured using the digital caliper, then ACD was calculated as C − (A + B). The length of the model eye (E in Figure 2 , Right) was measured using the digital caliper, the length between the posterior of the model eye and the apex of the retina (D in Figure 2 , Right) was measured using the digital thickness gauge, then AL was calculated as E − D. VL was calculated as AL − (CCT + ACD + LT). Using the refractive index of polymethyl methacrylate (1.485 at a wavelength of 840 nm) and saline solution (1.336 at a wavelength of 840 nm), axial biometry of the model eye was determined with ultra-long scan depth OCT. The measurement obtained with ultra-long scan depth OCT highly correlated with the geometry of the model eye with regard to all measured parameters ( Figure 3 ).
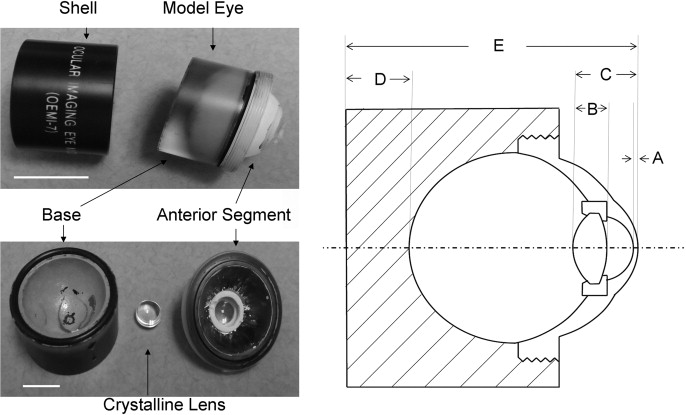
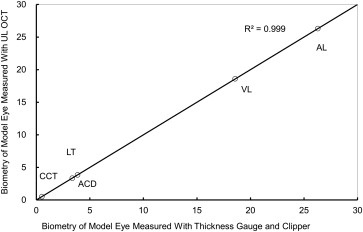
Custom software was developed to register automatically the two images obtained with the first 2 mirrors and to create an overlaid image. The common features of the iris and the anterior segment of the crystalline lens were used to register these 2 images. A full-eye image was reconstructed subsequently ( Figure 1 ). After removal of the central specular reflex (50 A-scans), hundreds of A-scans in the center were used to create a longitudinal reflectivity profile. The software automatically searched the peaks corresponding to each boundary, which were marked on the reflectivity profile. Inspection of the detected boundary location was conducted by comparing the peak locations and the reconstructed 2-dimensional image ( Figure 1 ). Corrections were made if the detected peak did not correspond to the boundary in the image. After the locations of the boundaries were confirmed by the inspection, the apparent distances of the central cornea, anterior chamber, crystalline lens, and vitreous chamber were measured along the central axis. At a wavelength of 840 nm, the refractive indices of the cornea, aqueous humor, crystalline lens, and vitreous were 1.387, 1.342, 1.408, and 1.341, respectively. Using the refractive index, the geometrical lengths of the central corneal anterior chamber, lens, and vitreous were calculated. The AL of the entire eye was the sum of the geometrical lengths of the cornea, aqueous, lens, and vitreous. The software saved all measurements into an Excel spreadsheet (Microsoft, Redmond, Washington, USA).
To validate software-generated results, the manual method for defining the boundaries was performed in Adobe Photoshop CS (Adobe, San Jose, California, USA) by obtaining the boundary pixel in each image. The marking was taken on the right side of the central specular reflex. The 2 images obtained with the first 2 mirrors were registered manually and were overlaid. Manual markings then were made on the overlaid image. The location of each boundary then was determined and used to calculate full-eye biometry with the set of refractive indices used in the software.
A statistical software package (SPSS for Windows version 17.0; SPSS, Inc, Chicago, Illinois, USA) was used for descriptive statistics and data analysis. All data were presented as the mean ± standard deviation. A paired t test was used, and P < .05 was considered a significant difference between 2 methods. The coefficient of repeatability (CoR) was defined as 2 standard deviations (SDs) of the difference between 2 measurements made by the same observer in different sessions for the same subject. The percentage of CoR was calculated as CoR divided by the average of each pair of measurements. The interclass correlation coefficients were calculated. Bland-Altman plots were used to determine the agreement between the 2 measurements of entire eye axial biometry.
Results
All of the parameters from the images obtained with ultra-long scan depth OCT were processed successfully with the automatic and manual methods. In group 1, there were no significant differences in the measured parameters between automatic and manual methods and between the first and second measurement ( P > .05; Table ; Figure 4 ). There was a strong correlation between the results using automatic and manual segmentation methods ( R 2 = 0.999; P < .05; Figure 5 ). The difference between the 2 measurements for each parameter was plotted using the Bland-Altman plot ( Figure 6 ). The CoRs of CCT, ACD, LT, VL, and AL were 0.021, 0.067, 0.071, 0.084, and 0.075 mm, respectively, using the automatic method ( Table ). The percentage of CoR ranged from 0.3% to 3.9%, and interclass correlation coefficients ranged from 0.946 to 0.999 ( Table ). Using the manual method, the CoR ranged from 0.011 to 0.073 mm, and the corresponding percentage of CoR ranged from 0.3% to 2.0% ( Table ). The interclass correlation coefficients ranged from 0.986 to 0.999 ( Table ). In group 2, AL measured by ultra-long scan depth OCT was 25.441 ± 1.071 mm with the automatic segmentation method, 25.444 ± 1.073 mm with the manual segmentation methods, and 25.342 ± 1.096 mm with the IOLMaster. There was a significant difference of 0.09 mm between 2 repeated measurements ( P < .05). The results from both automatic and manual methods correlated with the results measured by the IOLMaster ( R 1 2 = 0.987 and R 2 2 = 0.988, respectively; P < .05; Figure 7 ).
CCT | ACD | LT | VL | AL | |
---|---|---|---|---|---|
Manual method | |||||
Measure 1 (mm) | 0.531 ± 0.032 | 3.156 ± 0.280 | 3.887 ± 0.330 | 17.193 ± 1.057 | 24.767 ± 1.039 |
Measure 2 (mm) | 0.530 ± 0.032 | 3.157 ± 0.284 | 3.888 ± 0.331 | 17.188 ± 1.067 | 24.763 ± 1.047 |
Difference (mm) | 0.001 ± 0.005 | 0.001 ± 0.015 | 0.001 ± 0.018 | 0.005 ± 0.037 | 0.004 ± 0.036 |
CoR (mm) | 0.011 | 0.029 | 0.037 | 0.073 | 0.072 |
CoR % | 2.0 | 0.9 | 0.9 | 0.4 | 0.3 |
ICC | 0.986 | 0.999 | 0.998 | 0.999 | 0.999 |
Automatic method | |||||
Measure 1 (mm) | 0.529 ± 0.032 | 3.155 ± 0.276 | 3.898 ± 0.345 | 17.181 ± 1.064 | 24.763 ± 1.036 |
Measure 2 (mm) | 0.528 ± 0.031 | 3.157 ± 0.271 | 3.884 ± 0.339 | 17.189 ± 1.062 | 24.757 ± 1.033 |
Difference (mm) | 0.002 ± 0.030 | 0.002 ± 0.033 | 0.014 ± 0.035 | 0.008 ± 0.042 | 0.006 ± 0.037 |
CoR (mm) | 0.021 | 0.067 | 0.071 | 0.084 | 0.075 |
CoR % | 3.9 | 2.1 | 1.8 | 0.5 | 0.3 |
ICC | 0.946 | 0.993 | 0.995 | 0.999 | 0.999 |
Average manual measurement vs. average automatic measurement | |||||
Manual method (mm) | 0.531 ± 0.032 | 3.156 ± 0.281 | 3.888 ± 0.330 | 17.190 ± 1.062 | 24.765 ± 1.043 |
Automatic method (mm) | 0.529 ± 0.032 | 3.156 ± 0.273 | 3.891 ± 0.342 | 17.185 ± 1.063 | 24.760 ± 1.034 |
P value (paired t test) | .079 | .466 | .373 | .271 | .232 |
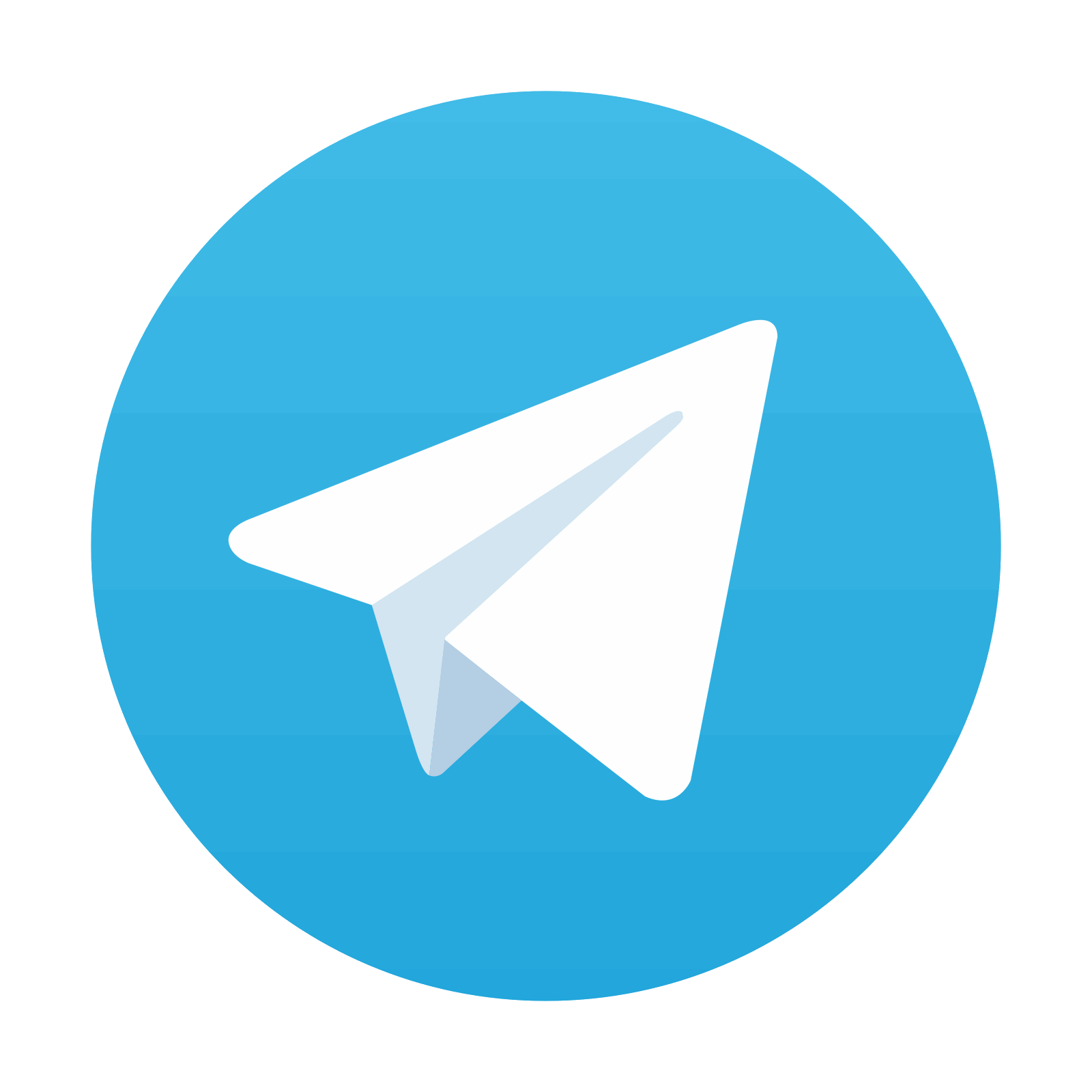
Stay updated, free articles. Join our Telegram channel

Full access? Get Clinical Tree
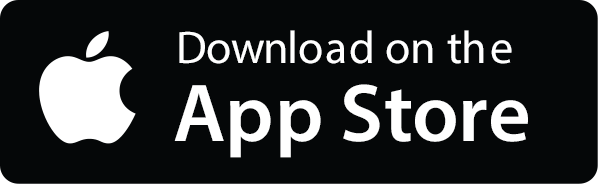
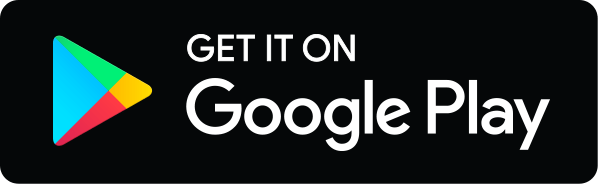
