Autologous Transplantation of the Retinal Pigment Epithelium and Choroid in the Treatment of Age-Related Macular Degeneration
Antonio López Bola˜os
Rosa Evangelina Garnica Hayashi
In the daily practice of ophthalmology, we encounter diseases whose treatments escape our hands. The physiopathology of these diseases includes damage or atrophy of retinal pigment epithelium (RPE), retinitis pigmentosa, geographic atrophy in age-related macular degeneration (AMD), RPE tears, and disciform scars.
RETINAL PIGMENT EPITHELIUM
There are approximately 3.5 million RPE cells in the adult human eye (1). The RPE cells are located between the neural retina and the vascular choroid and play a critical role in the maintenance of visual function. The functions of the RPE include phagocytosis of shed photoreceptor outer segments, metabolism of retinol, formation of the outer blood-retinal barrier, maintenance of the extracellular matrix, and regulation of ion and metabolite transport.
The RPE cells engulf and degrade shed rod outer segments at a very high rate: one human RPE cell will ingest and degrade about 3000 million discs during a 70 year lifespan. The apical surface of the RPE cells interface with the outer segments of the photoreceptors, while the RPE basal domain is attached firmly to the underlying Bruch’s membrane. The basement membrane of the RPE represents the innermost layer of Bruch’s membrane. RPE cells exhibit regional differences in morphology and function. In the macula, RPE cells are smaller and columnar in appearance, while in the periphery, they are larger and cuboidal.
In RPE cultures, the enzymatic capacity of RPE cells has been shown to be age independent (2), contrary to what may occur in vivo, where with age, degradation of outer segments of the photoreceptors appears to be diminished. With age and pathologic changes in the human eye, degradation of outer segment materials within the phagolysosomes may lead to the formation of lipofuscin granules composed of outer segments of the photoreceptors residue (3,4). In response to injury or certain alterations in the microenvironment, RPE cells, which in situ do not proliferate, may detach from their substratum, migrate, proliferate, and acquire a macrophagelike or fibroblast-like morphology (5,6). These morphologic and functional changes, associated with alterations on gene expression, may be referred to as activation. Activation events include physical trauma with displacement to a new environment, accumulation of intraocular blood, breakdown of the blood-retinal barrier with inflammatory cell infiltration, alteration of components of the extracellular matrix, alterations in choroidal circulation, and diffusion of oxygen to the RPE layer.
RPE shows specific features associated with aging. The proportion of apoptotic human RPE cells increases significantly with age, and these apoptotic cells are confined mainly to the macula (7). Peripheral RPE cells may compensate for the death of macular RPE cells. Furthermore, cells become more irregular in size and shape, deposits of RPE-derived material accumulate in Bruch’s membrane and lipofuscin appears in the cells’ cytoplasm (8, 9, 10, 11, 12 and 13).
ADVANCES IN TREATMENT OF AGE-RELATED MACULAR DEGENERATION
Nowadays, the possibility of rebuilding a damaged retina has been subject of widespread research. The field of AMD treatment has witnessed a number of significant advances.
AMD is a progressive disease of the macula that in its late or advanced stage results in loss of central vision. Visual loss in late AMD is a consequence of one of two processes that cause photoreceptor dysfunction- geographic atrophy or choroidal neovascularization (CNV). In geographic atrophy, there is confluent atrophy of the choriocapillaries and associated RPE. In CNV, an ingrowth of new vessels occurs from the choriocapillaries that breaches Bruch’s membrane and the RPE to invade the retina. These fenestrated and friable new vessels leak serous fluid, lipids, and blood below and into the neural retina, with subsequent fibrous scarring, which defines the late stage of AMD. This scarring is called disciform scar and is generally associated with the loss of neural tissue from the retina. The dysfunction as well as death of photoreceptors is a major contributor to vision loss in AMD. Other types of retinal neuronal cells affected secondarily because of the loss of photoreceptors also lead to visual loss.
The disciform scars are classified anatomically as subneurosensory retinal, sub-RPE, or combined. Subneurosensory retinal lesions are defined by the absence of the RPE interposed between the lesion and the remaining retinal tissue.
There are no preventive treatments, however, there is some evidence that antioxidant vitamin and mineral supplementation can slow down the progression of the disease in people with moderate AMD.
In the beginning of the 1980’s, the Macular Photocoagulation Study Group reported favorable outcomes for thermal laser photocoagulation in a small proportion of eyes with welldelineated (or classic) small extrafoveal and juxtafoveal CNV lesions, but less favorable outcomes in patients with subfoveal CNV lesions due to the likelihood of immediate and severe vision loss from the laser treatment (14, 15 and 16). In the 1990’s, introduction of photodynamic therapy with verteporfin expanded the therapeutic options available to patients with subfoveal CNV, offering them the possibility of exudative AMD treatment. However, the treatment is not accompanied with improvement in visual acuity and can also cause some degree of retinal damage (17).
The role of surgery in the treatment of CNV-complicated AMD remains controversial. Although submacular removal of CNV was not favored in the randomized submacular surgery trials, patients who underwent hemorrhagic CNV showed significantly reduced risk of severe visual acuity loss and contrast sensitivity loss compared to the control patients after 24 months of follow-up (18,19). Subfoveal membranectomy almost always results in the removal of the native RPE along with the choroidal neovascular complex in AMD eyes and leads to the development or progression of atrophy of the subfoveal choriocapillaris.
Other surgical treatments for AMD include pneumatic displacement of submacular blood with or without intravitreal or
subretinal injection of recombinant tissue plasminogen activator and translocation of the macula to an extramacular and healthier RPE area (Machemer 1993). Macular translocation seems to stabilize and sometimes even improve visual acuity (20,21). However, full macular translocation bears a considerable risk of proliferative vitreoretinopathy (PVR), diplopia, and macular edema.
subretinal injection of recombinant tissue plasminogen activator and translocation of the macula to an extramacular and healthier RPE area (Machemer 1993). Macular translocation seems to stabilize and sometimes even improve visual acuity (20,21). However, full macular translocation bears a considerable risk of proliferative vitreoretinopathy (PVR), diplopia, and macular edema.
Given the recent number of anti-VEGF therapies that have demonstrated efficacy in studies, the repertoire of treatments for neovascular AMD has significantly expanded. Bevacizumab, a full-length recombinant humanized monoclonal antibody against VEGF has been effective in AMD treatment (22, 23 and 24). Pegaptanib and ranibizumab have also been shown to be effective in large clinical trials. However, there are some patients who do not respond favorably to such anti-VEGF treatment (25,26).
For patients with geographic atrophy in AMD, RPE tears, or disciform scars, the treatment available is still under discussion. Currently, studies are investigating the effect of RPE transplantation.
Transplantation of RPE in AMD was first performed by Algvere et al. (27,28) by using heterologous fetal RPE. Unfortunately, all eyes developed macular edema, most likely as a result of host-graft rejection. Visual acuity declined and foveal fixation was lost in all patients. Iris pigment epithelium (IPE) was easily accessible; however, its functionality has not been established. Although the short-term results have been promising, there have been no long-term benefits. This led to the assumption that the transplantation of an intact monolayer is required to achieve a functional transplant.
Peyman et al. (29) first suggested translocation of peripheral choroid and RPE. Others later demonstrated the clinical feasibility of the new technique (30, 31
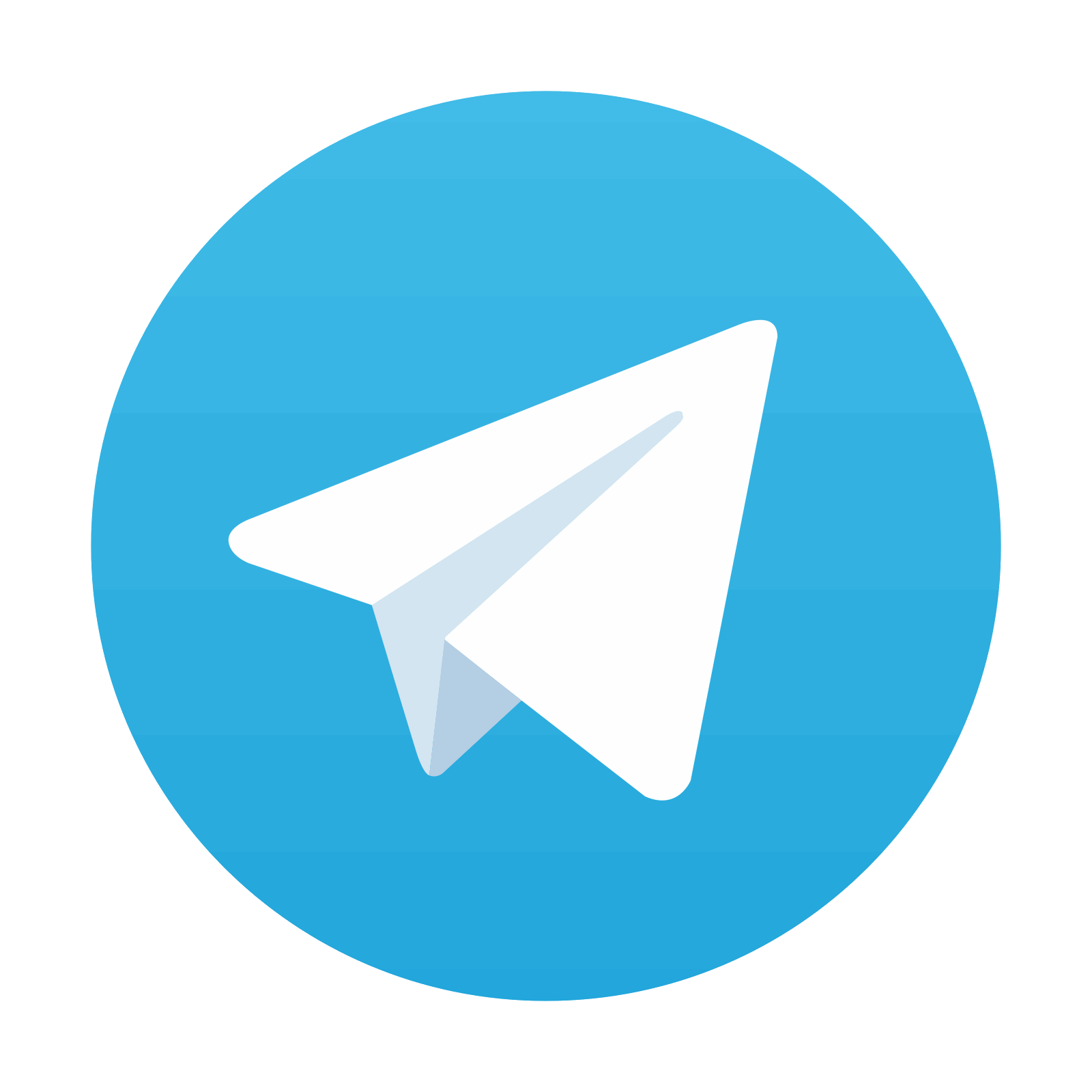
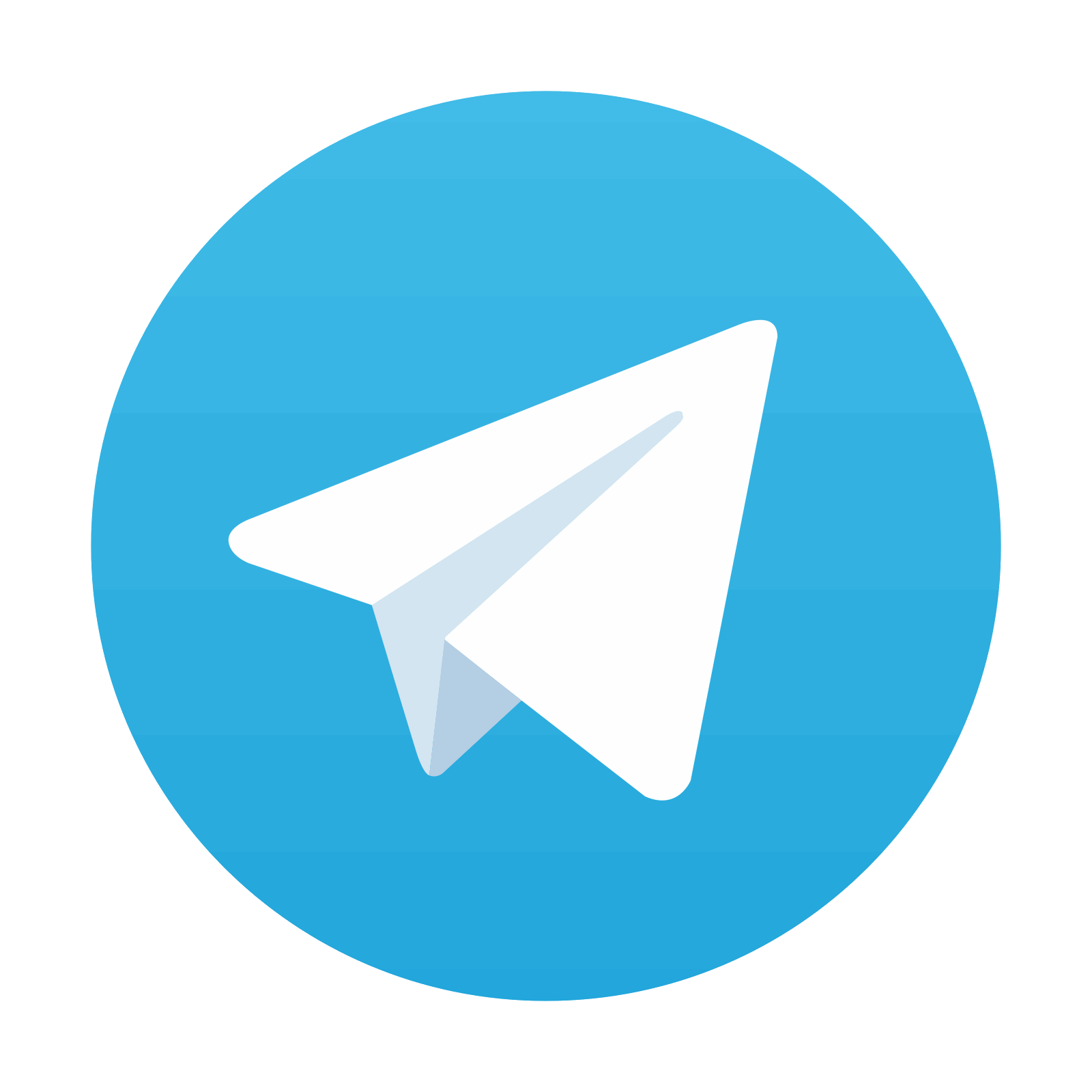
Stay updated, free articles. Join our Telegram channel

Full access? Get Clinical Tree
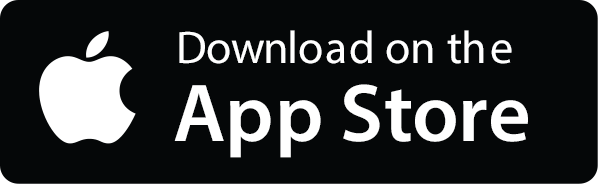
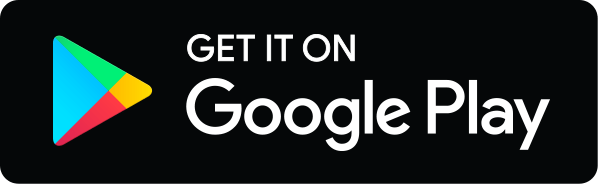