Artificial Vision
Nancy Kunjukunju
Hirokazu Sakaguchi
Motohiro Kamei
Hugo Quiroz-Mercado
At one time, it was believed that visual loss was permanent once the neural retina or optic nerve had been damaged. There were two events that modified this way of thinking. In 1968, a visual prosthesis was evaluated in a blind patient (1), and in 1974 an experiment was conducted in which a patient with no light perception vision could perceive light after an electrode was used to stimulate the visual cortex (2). At present there are a number of groups working on different approaches to developing a visual prosthesis for the blind.
Dobelle developed an approach that stimulated the visual cortex intracranially (2, 3, 4 and 5). In experiments conducted by Dobelle et al. and Norman et al., using either implanted surface electrodes or intracortical microelectrodes, cortical cells were activated thus stimulating the visual cortex (2, 3, 4 and 5). This type of electrical stimulation of the visual cortex requires intracranial surgery and as such, there is the potential for a high rate of complications such as CNS infection, epilepsy, and disturbance of the blood flow to the optic nerve.
On the other hand, Santos reported that 30% of the ganglion cells and approximately 80% of the inner nuclear layer cells remain histologically intact after death of photoreceptors in the eyes with severe visual loss due to retinitis pigmentosa (RP) (6). Other groups, therefore, have approached the development of a visual prosthesis stimulating those remaining inner retinal neurons. One such ophthalmological approach involves the use of subretinal electrodes and has been investigated by Chow et al. (7, 8 and 9) and Zrenner et al. (10,11) (Figs. 58-1 and 58-2). Using this method, lost photoreceptor function is replaced by a subretinal microphotodiode array (MPDA) that activates the remaining retinal network. Another approach used by certain groups such as Eckmiller et al. (12,13), Humayan et al. (14,15), Rizzo et al. (16,17) and Walter et al. (18) investigates the use of an epiretinal device to stimulate ganglion cells from an implanted microelectrode array from the vitreal side of the retina (Fig. 58-3). Another viable option is approaching the retinal prosthesis from within the suprachoroidal space. Sakaguchi and Tano et al. reported regarding the suprachoroidal transretinal stimulation system (STS) in which an electrode upon insertion into the suprachoroidal space elicits an electrical evoked potential (EEP) through transretinal stimulation (19), and Fujikado and Tano et al. also reported the effect of this system in clinical trial (20).
There are advantages and disadvantages to each method of electrical stimulation. Implanted cortical electrodes can treat blindness with complete atrophy of the retina or optic nerve, but requires intracranial procedures and may result in limited spatial perception. Retinal stimulation (Fig. 58-4), in contrast, utilizes the preexisting signal-processing network along the proximal visual pathways and is expected to have higher resolution. The visual field through an epiretinal electrode for instance, has a visual angle of 10 degrees (21). However, the area of visual field that will be reconstructed by a small (several millimeter square or in diameter) electrodes array is limited. Retinal stimulation involves predictable retinal damage due to the chronic direct attachment of an implant array. Moreover, the epiretinal or subretinal electrode necessitates that sufficient bipolar cells or ganglion cells remain in the small area around the electrode to elicit a response to electrical stimulation.
At present, there are five clinical trials underway to evaluate the efficacy of visual restoration through retinal prosthesis (22). Second Sight Medical Products, Inc has a 16-electrode epiretinal device that has been implanted in six subjects in the United States for over five years (23). A second-generation device has 60 electrodes and is currently in phase 2/3 of a clinical trial. In Germany, Intelligent Medical Implants GmBH has implanted four patients with a 49-electrode epiretinal array (24). The fourth clinical trial involves another epiretinal implant, the EPI RET3, which is a 25-electrode array that was implanted for four weeks in six blind patients (25). In the fifth clinical trial a 1550-MPDA and a 4 × 4 electrode array from Retina Implant AG, Reutlingen, Germany, was implanted in the subretinal space of eight patients (26).
EPIRETINAL PROSTHESIS
In animal models, epiretinal stimulation was shown to reproducibly elicit neural responses in the retina. In humans, preliminary tests of epiretinal electronic stimulation showed that patients were able to identify a crude shape and there was no persistence of the image, either a letter or box shape, pursuant to stimulation (14,15 and 27).
The epiretinal prosthesis (Fig. 58-3) is composed of extraocular and intraocular components. The external component consists of a lightweight camera built into spectacles, pocket batteries and a small pager-sized processing unit. The camera is used to capture and digitize images from the external environment. These images are then transformed into patterns of electrical stimulation that can excite inner retinal neurons. Information and power are relayed from the external portion to an internal receiver/stimulating microelectronic chip and microelectrode array (28).
There are advantages and disadvantages to the epiretinal approach. The advantages include (a) the vitreous acts as a sink for heat-dissipation from the microelectronic device, (b) the implantable portion of the device has little microelectronics, (c) the wearable or extraocular portion of the device allows easy upgrades without needing subsequent surgery and (d) electronics allow user and doctor full control over every electrode parameter and digital signal processing involved in imaging objects. Disadvantages include (a) the techniques that necessitate prolonged adhesion of the device to the inner retina using retinal tacks, (b) a wire connecting extraocular and intraocular components forms a permanent track between the vitreous cavity to the outside, which may raise a possibility of late infection and detachment of the electrodes from the retina associated with the eye movement, and (c) the large amount of current required to stimulate target bipolar cells using an epiretinal device and be the close proximity of this current to target cells (28).
The Intraocular Retinal Prosthesis (IRP) developed in conjunction with Second Sight Medical Products, Inc. converts
external images that are captured via camera into a pixilated image (15). The processed information is transmitted into the eye by magnetic coils in the form of controlled electrical pulses. A transscleral cable delivers the electrical stimulation pattern to an internal array of 16 platinum microelectrodes, ranging from 250 to 500 μm. The pulsed information transmitted to the microelectrodes on the array stimulates viable inner retinal neurons. Temporal to the fovea, the array is attached to the inner retinal surface via a tack that is inserted into the sclera (29). Canine retina implanted with the epiretinal prosthesis showed no evidence of rejection and examination of the retinal tack showed minimal effect on the retina (30).
external images that are captured via camera into a pixilated image (15). The processed information is transmitted into the eye by magnetic coils in the form of controlled electrical pulses. A transscleral cable delivers the electrical stimulation pattern to an internal array of 16 platinum microelectrodes, ranging from 250 to 500 μm. The pulsed information transmitted to the microelectrodes on the array stimulates viable inner retinal neurons. Temporal to the fovea, the array is attached to the inner retinal surface via a tack that is inserted into the sclera (29). Canine retina implanted with the epiretinal prosthesis showed no evidence of rejection and examination of the retinal tack showed minimal effect on the retina (30).
Patients implanted with the epiretinal device were able to discriminate between visual precepts created by different electrodes based on position; they also had the ability to distinguish levels of brightness based on various levels of current (31
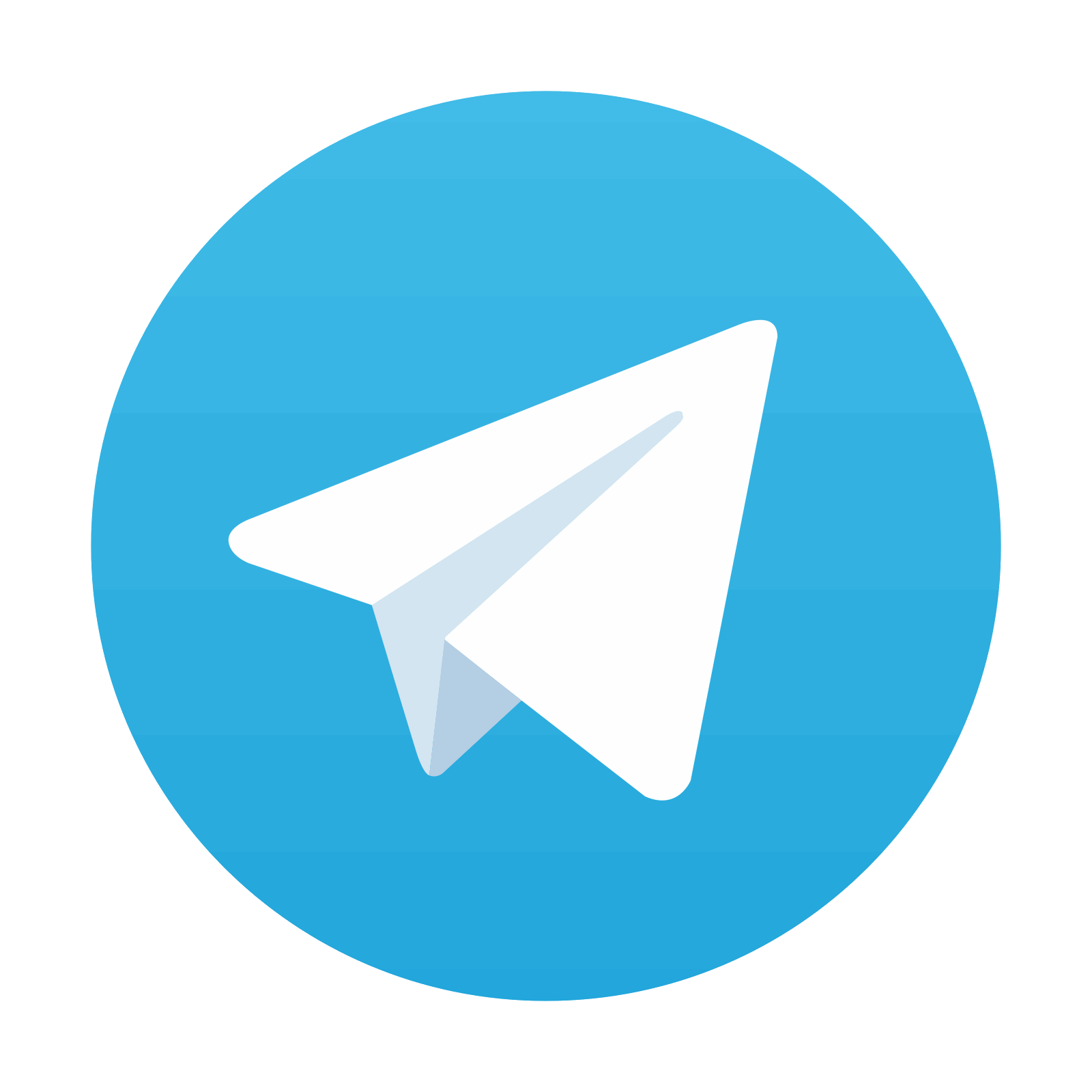
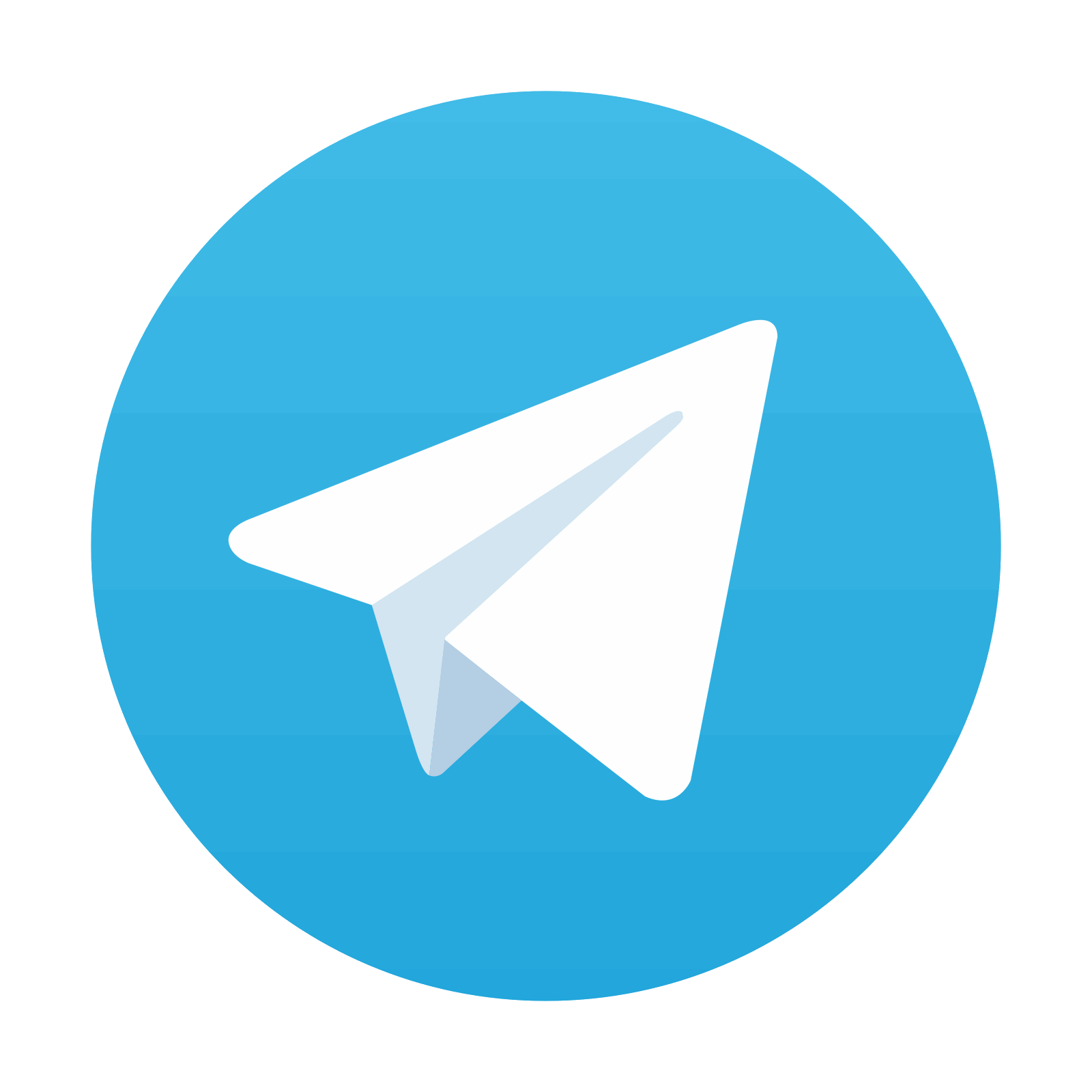
Stay updated, free articles. Join our Telegram channel

Full access? Get Clinical Tree
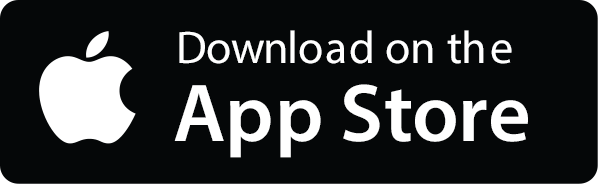
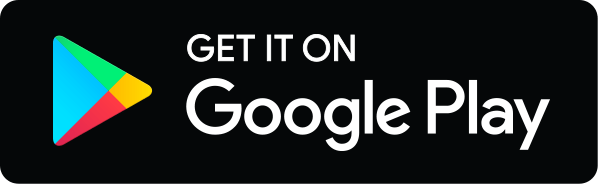