FUNCTION OF AQUEOUS HUMOR
Aqueous humor was originally thought to be stagnant. It was not until 1921 that Seidel proved that the aqueous was, indeed, circulating. Using a needle, Seidel connected a reservoir containing a blue dye to a rabbit eye. When the reservoir was lowered, clear fluid from the anterior chamber entered the tubing; when the reservoir was raised, the dye entered the eye and eventually appeared in the blood of the episcleral venous plexus. Seidel concluded that aqueous humor must be continuously formed and drained. Two decades later, Ascher showed that aqueous humor enters the venous system at the limbus through the aqueous veins and first flows alongside the bloodstream in a laminar fashion before mixing completely with the blood in the veins. Ashton studied neoprene casts of Schlemm’s canal and the aqueous veins and demonstrated a direct connection between these two structures. From the work of the last half century it is clear that aqueous humor is a relatively cell-free, protein-free fluid that is formed by the ciliary body epithelium in the posterior chamber. It then passes between the iris and the lens, enters the anterior chamber through the pupil, and exits the eye at the anterior chamber angle through the trabecular meshwork, Schlemm’s canal, and the aqueous veins. In the anterior chamber, the aqueous humor is subject to thermal currents because of the temperature difference between the iris and the cornea; aqueous rises close to the warmer iris and descends close to the cooler cornea. This convection current may easily be seen clinically when there are cells or pigment in the anterior chamber, and explains the relatively inferior location of pigment deposition (Krukenberg spindle) and keratic precipitates on the inner surface of the cornea.
During its passage through the eye, the aqueous humor serves a number of important functions. It serves in lieu of a vascular system for the normally avascular structures of the eye, including the cornea, lens, and trabecular meshwork. It brings to the internal eye essential nutrients, such as oxygen, glucose, and amino acids, and removes metabolites and potentially toxic substances, such as lactic acid and carbon dioxide. Aqueous humor provides the proper chemical environment for the tissues of the anterior segment of the eye and provides an optically clear medium to allow good visual function. It inflates the globe and maintains intraocular pressure (IOP), both of which are important for the structural and optical integrity of the eye. In many species, including humans, aqueous humor contains a very high concentration of ascorbate, which may act to scavenge free radicals and protect the eye against the effects of ultraviolet and other radiation. Under adverse conditions (e.g., inflammation, infection), it facilitates cellular and humoral immune responses. During inflammation, the rate of aqueous humor formation decreases, and its composition is altered to permit accumulation of immune mediators ( Box 2-1 ).
Brings oxygen and nutrients to cells of lens, cornea, iris
Removes products of metabolism and toxic substances from those structures
Provides optically clear medium for vision
Inflates globe and provides mechanism for maintaining intraocular pressure
High ascorbate levels protect against ultraviolet-induced oxidative products, e.g., free radicals
Facilitates cellular and humoral responses of eye to inflammation and infection
Several risk factors probably contribute to damaging the optic nerve with its resultant visual loss in glaucoma. Intraocular pressure that is too high for the continued health of the nerve is universally accepted as one of the most important of those risk factors. Therefore the study of those elements that contribute to the creation, maintenance, and variation of IOP is material to the understanding of the pathophysiology of this disease. Aqueous formation (F), facility of outflow (C), and episcleral venous pressure (P v ) are the major intraocular determinants of IOP. These factors are related to one another by the Goldmann equation:
P O = F / C + P v
F = ( P O – P v ) C
F = ( P O – P e ) C + U
ANATOMY OF THE CILIARY BODY
STRUCTURE
The ciliary body is the portion of the uveal tract that lies between the iris and the choroid ( Fig. 2-1 ). On cross-section, the ciliary body has the shape of a right triangle. It is attached anteriorly to the scleral spur, creating a potential space (supraciliary space) between itself and the sclera. The iris inserts into the short anterior side of the ciliary body, leaving a narrow width of ciliary face visible on gonioscopy between the peripheral iris and the scleral spur. The lens is attached to the ciliary body by the zonules, which separate the vitreous compartment posteriorly from the aqueous compartment anteriorly ( Fig. 2-2 ). The iris in turn divides the aqueous space into the posterior and anterior chambers. The junction of the iris, sclera, and cornea is called the anterior chamber angle.


The ciliary body is composed of muscle, vascular tissue, and epithelium. The ciliary muscle consists of three separate muscles – the longitudinal (meridional), the oblique (radial or intermediate), and the circular (sphincteric). The longitudinal muscle attaches anteriorly to the scleral spur and trabecular meshwork and posteriorly to the suprachoroidal lamina, with some fibers connecting to the choroid and sclera as far posteriorly as the equator of the globe. When the longitudinal muscle contracts, it pulls open the trabecular meshwork and Schlemm’s canal. The circular muscle fibers run parallel to the limbus. When these fibers contract they relax the zonules, allowing the lens to change shape. The radial muscle connects the longitudinal and circular muscles. The function of the radial muscle is not entirely clear, but it is postulated that contraction of the radial fibers may widen the uveal trabecular spaces. It is possible that some or all of the insertions of these ciliary muscle tendons are into an elastic fibrillar network which makes the resultant actions difficult to sort out.
The ciliary body runs from the scleral spur to the ora serrata, a distance of approximately 6 mm (see Fig. 2-1 ). The posterior portion of the ciliary body has a relatively flat inner surface and is named the pars plana. The anterior portion of the ciliary body has approximately 70 to 80 radial ridges (the ciliary processes) on its inner surface and is named the pars plicata ( Fig. 2-3 ). The ciliary processes are approximately 2 mm in length, 0.5 mm in width, and 0.9 mm in height. The surface area of the pars plicata is estimated to be 5.7 cm 2 in rabbits and 6 cm 2 in humans. Thus the pars plicata has a large surface area (approximately five times the surface area of the corneal endothelium) for both active fluid transport and ultrafiltration.

Because of the invagination of the embryonic optic vesicle, the inner surfaces of both the pars plana and the pars plicata are lined by two layers of epithelium – an outer pigmented layer that is continuous with the retinal pigment epithelium, and an inner non-pigmented layer that is continuous with the retina ( Fig. 2-4 ). The two layers of the epithelium have their apical surfaces in apposition.


ULTRASTRUCTURE OF THE CILIARY PROCESSES
Each ciliary process is composed of a central core of stroma and capillaries covered by a double layer of epithelium (see Fig. 2-4B ). The capillary endothelium is thin and has tiny fenestrae that face toward the pigmented ciliary epithelium. The capillary endothelium is surrounded by a basement membrane that contains mural cells (pericytes).
The vascular tissue is surrounded by a thin stroma composed of ground substance, collagen fibrils, and occasional wandering cells. The ground substance contains mucopolysaccharides, protein, and a solute of plasma.
The pigmented epithelium is composed of low cuboidal cells with numerous cytoplasmic melanin granules ( Fig. 2-5 ). This layer is separated from the stroma by an atypical basement membrane, a continuation of Bruch’s membrane containing collagen and elastic fibers. The function of the pigmented epithelium is not entirely clear. The basal portion of this layer has a great number of infoldings and mitochondria, suggesting a role in active metabolic processes. The cytoplasm and cell membrane of the pigmented epithelial cells stain for the presence of carbonic anhydrase.

The non-pigmented epithelial layer is composed of columnar cells, which are separated from the aqueous humor by a basement membrane. The non-pigmented ciliary epithelium has the morphologic features of a tissue involved in fluid transport, including extensive infoldings in the basal and lateral membranes, numerous mitochondria, well-developed rough endoplasmic reticulum, and tight junctions connecting adjacent apical cell membranes (see Fig. 2-5 ). In addition, sodium-potassium adenosine triphosphatase (Na + -K + ATPase) is found near the lateral infoldings of the membranes. Rows of vesicles are seen near the free surface of the epithelium and were called pinocytotic vesicles in the past. It now appears that these vesicles are a fixation artifact.
The potential space between the two epithelial layers is called the ciliary channel. One group of investigators postulates that aqueous humor is secreted into this space, particularly after stimulation of the system with various agents, such as β-adrenergic agonists. This theory requires further study.
Adjacent cells within each epithelial layer and the apical surfaces of the two layers are connected by gap junctions, puncta adherentia, and desmosomes. Gap junctions are low-resistance pathways that provide electrical coupling of cells and facilitate transport of ions and other molecules from one cell to another. Some feel that these gap junctions allow the two kinds of epithelial cells to act as a functional syncitium. Evidence from studies in knock-out mice suggest that connexin43, a major component of the gap junction, is required for aqueous production and mice bred to exclude this component do not produce normal amounts or quality of aqueous humor. Puncta adherentia and desmosomes are structural supports between cell membranes. The non-pigmented ciliary epithelial cells are also joined at their apical membranes by tight junctions (zonulae occludentae), which are thought to be an important component of the blood–aqueous barrier. Tracers injected into the ciliary body pass through the stroma and the clefts between the pigmented epithelial cells until they reach the apical cell membranes of the non-pigmented ciliary epithelium, where they are restricted by the tight junctions. However, these tight junctions are permeable to low molecular weight polar solutes.
There is considerable evidence that aqueous humor is produced in the anterior portion of the ciliary processes. The anterior portion of the non-pigmented ciliary epithelium has morphologic features that indicate active fluid transport, including increased basal and lateral interdigitations, numerous mitochondria, and a well-developed rough endoplasmic reticulum. The epithelium is supplied by a rich capillary network with numerous fenestrations. With a gonioprism, systemically administered fluorescein can be observed entering the posterior chamber at the tips of the ciliary processes. Finally, the non-pigmented ciliary epithelium, especially in the region of the lateral interdigitations, shows evidence of abundant Na + -K + ATPase, considerable activity for glycolytic enzymes, and a high rate of incorporation of labeled sulfate into macromolecules, such as glycolipids and glycoproteins.
Many nerve terminals are seen in the connective tissue adjacent to the pigmented epithelium, but they do not appear to penetrate the basal lamina and reach the non-pigmented epithelium. These terminals appear to arise from sympathetic and parasympathetic fibers. In addition, the non-pigmented ciliary epithelial cells have β-adrenergic and cholinergic receptors. The function of these receptors and nerve terminals is not clear.
VASCULAR SUPPLY
The long posterior ciliary arteries arise as trunks from the ophthalmic artery, pierce the globe near the optic nerve, and run forward to the ciliary body, where they anastomose to form the major arterial circle ( Fig. 2-6 ). The anterior ciliary arteries also contribute to the major circle, but to a lesser extent than the long posterior ciliary arteries. Several pre-capillary arterioles branch from the major arterial circle to supply each ciliary process. These arterioles have sphincters that may play a role in regulating blood supply and aqueous humor formation. The pre-capillary arterioles break up into plexuses of tortuous vessels within each ciliary process. The vessels collect and flow into choroidal and pars plana veins, which then flow into the vortex system. Some drainage also occurs via the intrascleral veins to the episcleral veins.

The ciliary body has a very high blood flow, estimated to be 81 μl/min in the monkey eye and, by calculation, 154 μl/min in humans. In cats, venous blood from the anterior uvea is only 4–5% less saturated with oxygen than is arterial blood. This indicates that oxygen consumption is not a limiting factor in aqueous humor formation under normal conditions. The rate of plasma flow to the ciliary processes in rabbits is at least 50 μl/min. If the rate of aqueous production is assumed to be 2–4 μl/min, the formation of aqueous humor removes only 4–8% of the volume of plasma available to the ciliary processes. Thus a modest reduction in the rate of plasma flow to the ciliary processes might not be expected to decrease aqueous humor formation substantially. However, animal models do indicate that aqueous humor production falls if ciliary blood flow is reduced by more than 30%. In fact, brimonidine, an α-adrenergic agonist commonly used for glaucoma treatment, may effect a reduction in aqueous formation by causing vasoconstriction of the ciliary body arterial supply.
The ciliary processes of many species, including primates, appear to have limited autoregulation of their blood supply. In general, the vascular responses of the ciliary processes are similar to those of the choroid but dissimilar to those of the iris and retina. Vasodilation of the ciliary blood vessels is seen following administration of carbon dioxide, application of prostaglandins, paracentesis, and parasympathetic stimulation. Vasoconstriction in the anterior uveal tract vessels occurs after stimulation of α-adrenergic nerves.
MECHANISM OF AQUEOUS FORMATION
The formation of aqueous humor is a complex process that is difficult to study. Probes that could measure composition or cellular processes themselves may disrupt normal function so it is difficult to extrapolate from data derived this way. Work in anesthetized animals adds the further artifact that both the anesthetic agent and the reduced blood flow may alter normal physiologic processes. Finally, work in excised preparations of ciliary body or cell cultures remove the tissue under study from the influences of normal blood flow and other body homeostatic mechanisms.
What is known has been derived from studies in experimental animals, excised tissues, cell culture, where possible, human studies, and from certain assumptions. Aqueous formation has several important processes that normally happen simultaneously: these include ultrafiltration and simple diffusional exchange of water and solutes between the plasma from blood flowing through the ciliary processes and the stroma of the ciliary processes. It has been known for a long time that aqueous humor is not a simple dialysate of plasma; the concentrations of many of the elements of the aqueous humor differ from those that would be expected if ultrafiltration and passive diffusion were the only processes. The Gibbs-Donnan equilibrium describes the concentration of substances in a dialysate; Table 2-1 contrasts the actual concentration of various solutes in the aqueous humor with that found in a simple dialysate. Active transport of substances from this dialysate of plasma then occurs first into the cells of the pigmented epithelium, then across the pigmented epithelium into the non-pigmented epithelium and finally from the non-pigmented epithelium into the posterior chamber. Water seems to be pulled along by osmotic forces. The fluid is further changed by diffusional exchange and active transport of substances out of the eye as it bathes other tissues, such as the lens, cornea, iris, and trabecular meshwork. Each of these processes involved in aqueous formation will now be discussed.
Substance | Aqueous humour concentration (plasma concentration) | Concentration in dialysate (plasma concentration) |
---|---|---|
Na + | 0.96 | 0.945 |
K + | 0.955 | 0.96 |
Mg ++ | 0.78 | 0.80 |
Ca ++ | 0.58 | 0.65 |
Cl 2 | 1.015 | 1.04 |
HCO 3 2 | 1.26 | 1.04 |
Glucose | 0.86 | 0.97 |
ULTRAFILTRATION
More than twice the weight of the ciliary processes themselves (or about 150 ml) of blood flows through the ciliary processes each minute. As blood passes through the capillaries of the ciliary processes, about 4% of the plasma filters through the fenestrations in the capillary wall into the interstitial spaces between the capillaries and the ciliary epithelium. The process by which a fluid and its solutes cross a semipermeable membrane under a pressure gradient (e.g., capillary blood pressure) is called ultrafiltration. In the case of the ciliary body, fluid movement is favored by the hydrostatic pressure difference between the capillary pressure and the interstitial fluid pressure (IOP) and is resisted by the difference between the oncotic pressure of the plasma and the aqueous humor.
The rate of protein leakage through the vessel walls into the tissue space of the ciliary processes is relatively low. However, the ciliary epithelial layers are even less permeable to the passage of colloids into the posterior chamber. Thus the colloid concentration in the tissue space of the ciliary processes is approximately 75% of that in plasma. The high concentration of colloids in the tissue space of the ciliary processes favors the movement of water from the plasma into the ciliary stroma but retards the movement of water from the stroma into the posterior chamber. Although a few investigators have postulated that ultrafiltration is responsible for the majority of aqueous humor formation, it is unlikely that the hydrostatic pressure difference between the ciliary capillaries and the posterior chamber can overcome the large oncotic pressure differential. Furthermore, a theory that proposes a predominant role for ultrafiltration does not explain why active ion transport inhibitors such as ouabain are capable of reducing aqueous humor formation by 70–80%. Thus ultrafiltration helps to move fluid out of the capillaries into the stroma but alone is insufficient to account for the volume of fluid moved into the posterior chamber. The latter step requires an active metabolic process. Ultrafiltration and active secretion occur in tandem.
ACTIVE TRANSPORT
Active transport (secretion) is an energy-dependent process that selectively moves a substance against its electrochemical gradient across a cell membrane. It is postulated that the majority of aqueous humor formation depends on an ion or ions being actively secreted into the intercellular clefts of the non-pigmented ciliary epithelium beyond the tight junctions ( Fig. 2-7 ). This process is accomplished by about a million non-pigmented epithelial cells, each of which secretes aqueous humor equal to about one-third of its own intracellular volume per minute. In the small spaces between the epithelial cells, the secreted ion or ions create sufficient osmotic forces to attract water. By the time the newly secreted fluid reaches the posterior chamber, the osmotic driving force has been nearly dissipated.

First, the dialysate from the plasma has to be transported into the pigmented epithelial cells. The best current evidence suggests that the paired Na + /H + and Cl − /HCO − antiports actively transport Na + and Cl − from the stroma into the cell. Intercellular gap junctions between the two cell layers appear to be critical. In addition, the natriuretic peptide precursor B (NPPB)-sensitive Cl channels at the basolateral surface in non-pigmented epithelial cells also play a crucial role in regulating the Cl movement across the functional syncytium.
It is not clear which ion or ions are actively transported across the non-pigmented ciliary epithelium, though most theories include sodium, chloride, and/or bicarbonate ( Table 2-2 ). Electrophysiologic studies of the isolated ciliary epithelium indicate that the transepithelial potential difference and the short circuit current, indicators of ion transport across membranes, are dependent on Na + and HCO 3 −. A number of investigators postulate that the active transport of the sodium ion is the key process in aqueous humor formation. This theory is supported by the observation that membrane-bound ouabain-sensitive Na + -K + ATPase (the enzyme that facilitates transport of potassium into, and sodium out of, cells) is found in the non-pigmented ciliary epithelium of many different species, which explains the 70–80% reduction in aqueous humor seen with oubain.
Substance (nM/kg H 2 O) | Rabbits * | Humans ** | |||
---|---|---|---|---|---|
Anterior chamber aqueous humor | Posterior chamber aqueous humor | Plasma | Anterior chamber aqueous humor | Plasma | |
Na + | 145 | 144 | 146 | 163 | 176 |
Cl – | 105 | 105 | 112 | 126 | 117 |
HCO 3 – | 28 | 34 | 24 | 22 | 26 |
pH | 7.6 | 7.57 | 7.40 | 7.21 | 7.40 |
Ascorbate | 0.96 | 1.30 | 0.02 | 0.92 | 0.06 |
* Modified from Kinsey VE, Reddy DVN: Chemistry and dynamics of aqueous humor. In: Prince JH, editor: The rabbit in eye research, Springfield, Ill, Charles C Thomas, 1966.
** Modified from Becker B: Chemical composition of human aqueous humor: effects of acetazolamide, Arch Ophthalmol 57:793, American Medical Association, 1957.
Enough Na + and K + ATPase activity is present in the ciliary non-pigmented epithelium to drive aqueous humor formation mainly by the sodium gradient. However, the primacy of Na + as the driver for aqueous humor formation has been questioned in several species including rabbit, cat, and ox. Candia et al suggested that while active transport of Na + is important, it cannot account for the entire rate of aqueous formation in vivo. At least in the pig and some other mammals, Cl − is actively transported across the non-pigmented ciliary epithelium and may be the driving force of aqueous formation. Do & Civan have found that swelling-activated calcium channels in the non-pigmented ciliary epithelium modulate the formation of aqueous in both the cow and the mouse. The notion that chloride transport may be important at least in some species is supported by the observation that, unlike the rabbit, the concentration of chloride ion in bovine, ovine and porcine aqueous humor is higher than in plasma.
For many years investigators believed that the bicarbonate ion could not be actively secreted into the aqueous humor of the primate eye because the posterior chamber bicarbonate concentration is lower than the plasma concentration. However, more recent investigations indicate that bicarbonate is actually present in excess in newly formed posterior chamber aqueous humor. Bicarbonate appears to be in deficit in the aqueous humor because the fluid that is usually sampled has undergone metabolism and diffusional exchange with surrounding tissue. Carbonic anhydrase (CA) type II (isoenzyme C) is present in the cell membrane and cytoplasm of the non-pigmented and pigmented epithelium of the ciliary body. This enzyme catalyses the following reaction:
CO 2 + OH – ⇄ CA HCO 3 –
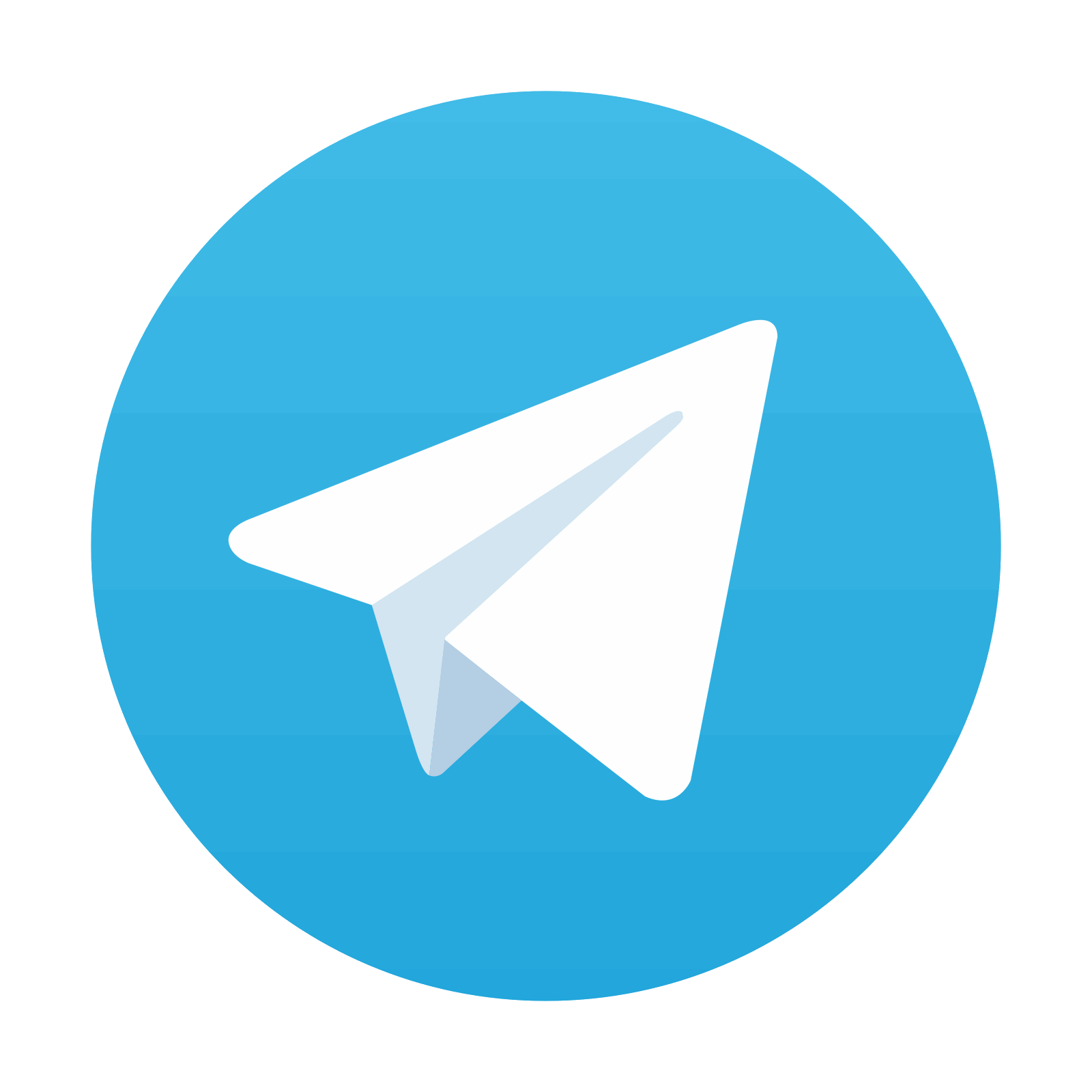
Stay updated, free articles. Join our Telegram channel

Full access? Get Clinical Tree
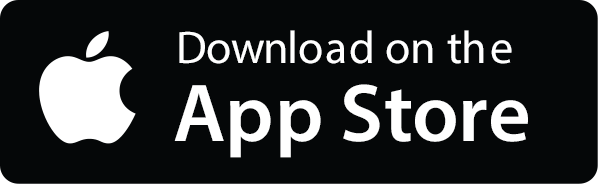
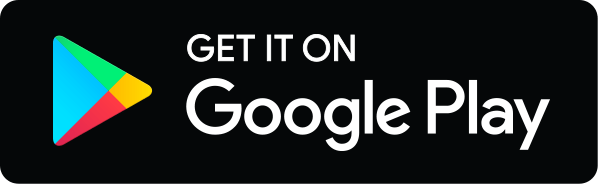