Key Points
- ▪
When anesthetic risk is correlated with age, children younger than 1 month of age are at the highest risk for adverse events during any procedure. The greatest improvements in patient safety have been in reducing the frequency of respiratory events. Elective procedures should be deferred until after the age of 6 months, when appropriate.
- ▪
The anesthetic plan is tailored to the patient’s age—for physiologic differences as well as differences in psychological development.
- ▪
Preoperative preparation that includes patient education leads to greater satisfaction with the overall hospital experience for both child and parent.
- ▪
Routes of premedication and induction of anesthesia include oral, intranasal, intramuscular, intravenous, and inhalational anesthetics.
- ▪
Decisions regarding deep versus awake extubation require careful consideration of clinical factors; awake extubation is always the appropriate choice in patients who have difficult airway anatomy or full-stomach physiology.
- ▪
Postoperative nausea and vomiting are best treated with multimodal therapy; a second dose of antiemetic agent in the same class of drug already given is rarely effective.
- ▪
Effective pain assessment and management are mandated by the Joint Commission for institutions to receive accreditation.
- ▪
Multimodal therapy for pain relief, with or without opioids, is now accepted as a more balanced approach to pain management in surgical patients. Such therapy aims to decrease the dose of any single agent and thereby improve antinociception and reduce side effects.
- ▪
Anesthetizing patients in locations outside of the operating room minimizes interference with surgical needs and contributes to efficient delivery of care.
- ▪
Implications of anesthetic neurotoxicity on the developing nervous system in humans is still unclear and is being researched. The most conservative approach would be to delay all elective procedures until after two years of age. The Food and Drug Administration does not support this position but suggests full disclosure when discussing surgical options with patients and their families.
- ▪
Medical conditions with particular impact on anesthetic considerations include upper respiratory infections, reactive airway disease, obstructive sleep apnea, cystic fibrosis, diabetes mellitus, and congenital heart disease.
- ▪
The 2007 American Heart Association guidelines for the prevention of infective endocarditis in patients with congenital heart disease seek to use evidence-based practices to minimize unnecessary exposure to antibiotics.
- ▪
Management of patients for bronchoscopy, adenotonsillectomy, and laryngeal surgery requires careful planning preoperatively and clear communication between the surgeon and the anesthesiologist throughout the procedure.
Because of the inherent complexity of the structure-function relationship in otolaryngology, pediatric otolaryngologists and anesthesiologists must work together closely in the operating room (OR) to provide optimal care to the patient. This chapter focuses on anesthesia topics that are relevant for the pediatric otolaryngologist, beginning with an overview of anesthesia for children and the preoperative assessment of pediatric patients. A discussion of the perioperative management, including choice of drug and technique to match the situational demands, is described next, along with emergence from anesthesia and postoperative care. A brief overview of anesthesia for children outside the operating room and the current review of safety of anesthesia in children are also discussed. Finally, the anesthetic implications of specific diseases and the anesthetic considerations in commonly performed pediatric otolaryngology procedures are reviewed.
Anesthesia for Children
Anesthesia carries increased risk in children compared with adults, as reported more than 50 years ago. Work from the 1960s correlated this increased risk with age less than 1 year, which is not surprising given the physiologic changes that occur during the first year of life. Nowadays, children younger than 1 month of age are recognized as being at the highest risk, which suggests that the risk of adverse events with anesthesia induction in infants is inversely proportional to age. Respiratory control is immature in infants, the carbon dioxide (CO 2 ) response curve is flatter and is shifted to the right compared with the curve in older children and adults, and hypoxia may induce apnea rather than hyperventilation. Cardiovascular responses also are immature; the myocardium is poorly compliant, so cardiac output is rate dependent. Similarly, responses to anesthetic agents differ in accordance with patient age. Preterm and full-term newborns require lower concentrations of inhaled anesthetics than those needed in older children or adults. Inhalational agents may cause more depression of cardiac output in infants and may have a more profound effect on baroreflexes. Pharmacokinetic parameters of volume of distribution, hepatic clearance, and renal clearance differ profoundly from those in older children, which affects the dose of nearly every drug.
These differences in physiology and pharmacology are reflected in the types of anesthetic mishaps that tend to occur in newborns and infants. Inadequate ventilation and anesthetic overdose were the most frequent sources of anesthetic morbidity and mortality in the 1980s. Changes in anesthetic practice, such as improvement in monitoring—that is, using pulse oximeters and CO 2 monitors—and use of different drugs, such as sevoflurane instead of halothane, have decreased respiratory events and shifted the number of anesthesia-related cardiac arrests in infants toward cardiovascular causes of cardiac arrest.
Adherence to the American Society of Anesthesiologists monitoring standards is basic to all anesthesia care performed by an anesthetist and has reduced the incidence of intraoperative complications. The components of these standards are presented in Box 3-1 . Most anesthesiologists consider pulse oximetry to be an indispensable part of the intraoperative monitoring armamentarium, although it has been difficult to document improvement in outcome as a result of this technique, even in very large series. The use of pulse oximetry alone or in combination with capnography has reduced the relative frequency of adverse respiratory events as compared with adverse cardiovascular events, perhaps because pulse oximetry and capnography are more effective for preventing respiratory events than cardiovascular events.
Continuous presence of qualified anesthesia personnel in the operating room
Continuous evaluation of the following:
- •
Oxygenation
- •
Inspired oxygen concentration with an oxygen analyzer
- •
Blood oxygenation with a pulse oximeter
- •
Ventilation
- •
Qualitative assessment that include chest excursion, observation of the reservoir bag, and auscultation of breath sounds
Quantitative assessment with end-tidal CO 2 analysis
A low-pressure disconnect alarm when mechanical ventilation is used
Circulation
Continuous electrocardiogram and heart rate
Arterial blood pressure at least every 5 minutes
At least one of the following:
- •
Palpation of pulse
- •
Auscultation of heart sounds
- •
Intraarterial blood pressure monitoring
- •
Ultrasound peripheral pulse monitoring
- •
Pulse plethysmography or oximetry
- •
Elective procedures should be deferred until the infant is at least 6 months to 1 year old, when anesthetic risk is likely to be lower. This is particularly true in infants who were born prematurely because they have an increased risk of postoperative apnea until approximately 55 weeks of postconceptual age. The use of trained subspecialty pediatric anesthesiologists for the care of infants and children has been suggested to be associated with improved outcomes, although this is difficult to prove.
Current estimates of anesthetic mortality vary widely and depend on the definition of “anesthetic death.” However, mortality in healthy pediatric outpatients as the result of an anesthetic-related cause is very rare, with rates perhaps as low as 0.36 in 10,000 cases. Accordingly, parents of otherwise healthy children can be reassured that outcomes in modern-day pediatric anesthesia are excellent.
Preoperative Assessment
Today’s emphasis on cost containment has forced many changes in the practice of pediatric anesthesia. More and more patients with complex medical problems are having procedures as outpatients, which forces the preoperative contact between the anesthesiologist and the patients and family into a very few minutes. In addition, efficiency pressures have forced institutions to search for ways to maximize patient flow in surgery areas and to minimize unexpected delays or cancellations. Preoperative anesthesia clinics have developed in response to these changes.
A visit to the preanesthesia clinic before the day of surgery affords the opportunity for taking an anesthetic history, performing a physical examination, obtaining necessary laboratory tests, and informing the family about the anticipated procedures. Information conveyed includes times for restriction of oral intake to nothing by mouth (NPO status), premedication, anesthetic induction and maintenance, and postoperative pain management techniques. It also allows confirmation that all necessary paperwork, such as consent and surgical history and physical, is present in the medical record. An attending anesthesiologist is available for consultation for more complicated cases or to address specific clinical questions. Anesthetic risk can be discussed with patients and their families, with ample time allowed for them to ask questions. In addition, the clinic nurse can take this opportunity to educate the patient and family about the impending surgery and to identify fears and concerns of the parents and child.
A preoperative medical assessment includes a review of systems and the patient’s response to previous anesthetics; current medications; allergies; recent illnesses, including upper respiratory infections; and family history of problems with anesthesia. The physical examination concentrates on airway anatomy and includes the mouth, jaw, teeth, and neck along with chest and heart auscultation to confirm the absence of significant cardiorespiratory disease. Laboratory tests are obtained as indicated by the child’s condition and type of surgery planned, rather than as a routine component of the assessment. Identification of clinical problems at a preanesthesia visit also allows appropriate evaluation or referral before surgery, thereby avoiding delay or cancellation on the day of surgery.
Preparation for Anesthesia
Behavioral Preparation
The days and hours leading up to surgery can be an anxious time for both children and parents. Fears about hospitalization are common among children, including fear of separation, pain, loss of control, and even death. Many of these fears depend on the child’s age ( Table 3-1 ). Awareness of these developmental stages allows the anesthesiologist to better anticipate the needs of most children. In addition, understanding the impact of parental anxiety on a child’s anxiety can help in the selection of interventions that will be helpful to both the child and the parents.
Age | Specific Concerns |
---|---|
Infant (6-18 months) | Separation anxiety |
Toddler (1-2 years) | Fear of strangers, loss of mastery of the environment |
Preschool (2-5 years) | Separation anxiety; difficulty distinguishing reality from fantasy; fear of pain, mutilation |
School age (6-12 years) | Fear of the unknown, pain, mutilation, loss of control or autonomy |
Adolescent (13-18 years) | Precarious sense of self; fear of pain, mutilation, loss of control or autonomy |
Details of the anesthetic and surgical experience should be presented in age-specific language for children of appropriate age. Children who are given specific information before surgery have been shown to be less anxious than children who are given only general information. Movies or videotapes can help children and parents cope with the mysteries of hospital routines. Comprehensive preoperative preparation programs have been shown to be helpful for reducing preoperative anxiety and enhancing coping behavior in children. Learning coping strategies and developing a better understanding of the environment in which they will receive their care may be especially important for children who will require repeated procedures. Parents also should be involved in these programs because decreasing their anxiety is likely to provide the added benefit of further decreasing the child’s anxiety. These efforts are important even if pharmacologic agents will be used to decrease anxiety because the combination of psychologic and pharmacologic interventions can produce synergistic effects.
Premedication
The choice of premedication is based on the age and specific needs of the patient. The usual goals of premedication are to reduce anxiety and to ease separation from parents. Because most children fear needles, the oral administration of sedatives has become the standard of care at many institutions. Children who are younger than 8 months of age generally do not need premedication and separate easily from their parents. Children between 8 months and 8 years of age often experience enough preoperative anxiety that preoperative medication may be helpful. Midazolam (0.5 to 1.0 mg/kg up to a maximum of 20 mg), mixed in flavored syrup and administered orally 10 to 20 minutes beforehand has become the most popular agent for preoperative sedation for children to smooth separation from the parents and ease induction of anesthesia. Midazolam usually is well tolerated, although a rare decrease in oxygen saturation or blood pressure may be observed. In addition, intravenous (IV) flumazenil (10 µg/kg, administered over 1 minute, repeated as needed for a total of 5 doses; max. 0.2 mg/dose, 1 mg total dose) can be administered to reverse adverse effects such as oversedation, paradoxic agitation, or emergence delirium.
Older children may be willing to have an IV line started preoperatively, especially if a topical anesthetic cream such as EMLA ( e utectic m ixture of l ocal a nesthetics) or ELA-Max is used. EMLA cream is a mixture of lidocaine and prilocaine that penetrates intact skin, and when applied for 60 minutes or more, it significantly decreases the pain associated with an IV insertion. EMLA should be used with caution in children younger than 3 months of age or in children who are receiving other medications that may induce methemoglobin because of the risk of methemoglobinemia. Plasma concentrations of lidocaine and prilocaine achieved with topical application are well below the toxic level, but they may be higher in children with traumatized or inflamed skin. Another effect of EMLA that may be problematic is vasoconstrictive blanching, which makes the identification of potential IV insertion sites difficult for some practitioners. ELA-Max is an alternative to EMLA, and it contains only lidocaine in a liposomal matrix that allows for effective absorption across intact skin. ELA-Max has been shown to provide equal topical anesthesia to EMLA, and it has a quicker onset of 30 minutes. ELA-Max also produces less blanching than that seen with EMLA. If an IV line is placed, midazolam or another sedative can be titrated intravenously until the desired effect is achieved. Although the anesthetic creams are most frequently used, alternative methods for providing topical anesthesia may be standard in some institutions.
Younger children may not accept oral premedication well, which makes this method of administration as anxiety provoking as separation from the parents without any premedication. Intranasal or intramuscular administration of premedications may also be useful alternatives. Intranasal midazolam (0.2 to 0.3 mg/kg) can be easily administered using either an atomizer or simply a needleless tuberculin syringe in a patient small enough to be held or restrained. Intranasal midazolam begins acting immediately after dosing and reaches peak concentrations by 14 minutes, with the advantage of increased bioavailability with minimal first-pass metabolism in the liver. Intramuscular (IM) administration of premedication becomes necessary in larger children with behavioral issues that limit patient cooperation—autism, for instance. Because IM injection is technically easier than IV line placement, this technique can be achieved quickly, and the child then be allowed some time with the parent to minimize the trauma from the encounter. IM ketamine at a dose of 2 to 5 mg/kg or a combination of IM ketamine and midazolam causes sedation and dissociation from the environment, which makes the patient more approachable for procedures, anesthesia induction, and other interventions. Larger doses of ketamine administered intramuscularly (up to 10 mg/kg) can induce general anesthesia (see the following discussion).
Anticholinergic agents such as atropine (0.02 mg/kg) or glycopyrrolate (0.01 mg/kg) given intravenously at the time of induction may be useful as premedications. Anticholinergics act to ameliorate the increase in vagal tone that may occur in cases that involve airway manipulation, when halothane is used as an inhalational agent, or in patients with copious airway secretions. Although halothane is no longer available for clinical use in the United States, it is still used in developing countries. Halothane may cause bradycardia and a significant reduction in cardiac output, particularly in children younger than 6 months of age; in younger patients, the routine use of anticholinergic agents with halothane can prevent these changes. Anticholinergics are also a useful adjunctive premedication to ketamine, which increases salivary secretions.
Nothing-by-Mouth Orders
To minimize the risk of the aspiration of gastric contents during the induction of anesthesia, an adequate period of fasting must be maintained. Traditionally, both food and fluids have been withheld after the midnight before surgery. However, a prolonged period of fasting is uncomfortable for children, unpleasant for the family, and risks hypovolemia. It has recently been recognized that the administration of clear liquids to children up to 2 to 3 hours before induction does not increase gastric residual volume or acidity compared with a more prolonged fast. In addition, morbidity and mortality rates for aspiration of gastric contents in children are exceedingly low. Thus, fasting guidelines have been liberalized in recent years ( Table 3-2 ). Some debate surrounds whether breast milk should be considered a solid or clear liquid, with many institutions allowing breastfeeding within the same guidelines as clear liquids. Nevertheless, in giving these instructions to parents, it is important to emphasize the safety issues involved to maximize compliance and avoid unnecessary delays in the start of the procedure.
Ingested Material | Minimum Fasting Period (hr) |
---|---|
Clear liquids | 2 |
Breast milk | 4 |
Infant formula and nonhuman milk | 6 |
Light meal | 6 |
Fatty food and meat | 8 |
Parental Presence
With increasing frequency, parents are expressing a desire to be present at the time of their child’s anesthetic induction. Although parents’ motives vary, most hope that the child’s anxiety will be lessened by the emotional comfort of their presence. Some hospitals have induction areas outside of the sterile envelope of the OR. If not, parents can be dressed in cover gowns and escorted into and out of the OR for the induction.
Studies to examine the impact of parental presence have had variable results: some show no significant impact, and some show a decrease in patient anxiety, and still others show an increase in patient anxiety. Parents with a high anxiety level seem to have a negative impact on their child during induction, whereas calm parents seem to have a positive impact. One survey reported that a majority of anesthesiologists are in favor of the practice of having parents present during induction, and many hospitals tend to support this request as a mechanism to improve patient and parent satisfaction. Thus it is likely that the trend will continue. Parents should be thoroughly educated about what to expect and how they can best help their child; they must agree to leave the induction area immediately when instructed to do so, and one member of the care team must be identified to help them during the induction. Highly anxious parents should be encouraged not to participate.
Anesthetic Induction
Anesthesia can be induced by mask inhalation of a potent anesthetic agent in oxygen with or without nitrous oxide (inhalation induction), by injection of a sedative-hypnotic agent through an IV catheter (IV induction), or by intramuscular injection. As much as possible, children are given a choice of technique; younger children tend to fear needles and prefer an inhalation induction, whereas older children and teenagers usually accept IV induction. Inhalation induction will take longer in teen-aged and older patients than in younger patients because of age-related physiologic differences. The prolonged time to effect must be taken into consideration if an inhalation induction is selected in older children, but it does not preclude such an induction. Certain clinical factors (e.g., obesity, severe gastroesophageal reflux, full stomach) also may incline the choice of induction technique toward IV.
Inhalation Induction
Sevoflurane is the inhalational induction agent of choice because of its minimal pungency, fewer cardiovascular side effects, and rapid uptake. A small amount of pleasantly scented liquid (e.g., bubble gum flavoring) applied to the inside of the mask disguises the odor of the anesthetic agent and improves the patient’s acceptance of the technique. Many anesthesiologists will begin the induction with the patient breathing 50% to 70% nitrous oxide, which is odorless, before introducing sevoflurane, thus making the addition of the sevoflurane less distressing. Noise in the room should be kept to a minimum during anesthesia induction, and the anesthesiologist often tells the child an entertaining story to direct the child’s thoughts away from the reality of the situation. As the concentration of the anesthetic agent is increased, most children lose consciousness in less than 1 minute. The parents, if present, can be ushered out at this point, and an IV catheter can then be placed.
Intravenous Induction
Intravenous induction requires the presence of an IV catheter and thus is reserved for older children or teenagers, children with a preexisting IV catheter, children who need a rapid-sequence induction because of a full stomach, or children who object to an inhalation induction. Owing to slower uptake of inhaled anesthetic agents, inhalational induction takes much longer in older, heavier children, and it poses a consequently higher risk for laryngospasm and bronchospasm. An IV induction is therefore also the preferable method in this patient group.
The most common agent for IV induction is propofol, an alkylphenol formulated as a 1% solution in a white soybean oil, egg lecithin, and glycerol emulsion. Propofol is highly lipophilic and rapidly redistributes throughout body compartments, with a distribution half-life of approximately 2 minutes and an elimination half-life of approximately 30 minutes. The dose for the induction of anesthesia varies with age ; infants 1 to 6 months of age require approximately 3 mg/kg, whereas older children require approximately 2.5 mg/kg. Propofol is the IV induction agent of choice for outpatient procedures because recovery is rapid, and the patient is clear-headed with minimal associated nausea and vomiting. The major disadvantage is pain on injection, particularly into small veins. Lidocaine (0.5 to 1.0 mg/kg) administered intravenously before or during propofol injection may decrease the incidence of pain. Propofol may cause hypotension, apnea, and desaturation; it can also be used for anesthesia maintenance as an IV infusion of 100 to 200 µg/kg/min. This technique can be very useful for patients who have a history of severe postoperative nausea or who are undergoing procedures in which postoperative nausea is especially common (e.g., tympanoplasty). Many pediatric anesthesiologists use a propofol infusion to provide anesthesia during bronchoscopy because it provides a reliable, stable method of administering anesthesia with spontaneous ventilation when the airway is shared with the surgeon, and it decreases the exposure of the surgeon to unscavenged anesthetic gases. An alternative IV agent for induction, thiopental, is an ultrashort-acting barbiturate that is no longer available for use in the United States
Etomidate, a short-acting nonbarbiturate, is also used as an IV induction drug. It is an imidazole-derived, steroid-based, potent sedative-hypnotic agent without analgesic properties. Etomidate has a rapid onset of action (5 to 15 seconds) with a peak effect at 60 seconds, and a short duration of action (3 to 5 minutes) that is terminated by redistribution. Its primary advantage is that it has minimal cardiac depressant effects or hemodynamic impact. Etomidate also reduces the intracranial pressure, the cerebral blood flow, and cerebral metabolic rate but has little effect on ventilation and smooth muscle tone. Bolus injection of etomidate does not release histamine. These features make it an extremely useful agent in critically ill children who have an unstable cardiovascular status and those with a head injury, hypovolemia, and trauma.
The recommended induction dose of etomidate in healthy children is 0.2 to 0.3 mg/kg, depending on cardiovascular status. Metabolism is via the liver. Etomidate has a number of side effects that preclude regular use. Like propofol, it burns with injection, an effect that is ameliorated with the newer formulation that dissolves the drug in a fat emulsion. Myoclonic movements are also common with its use but may be attenuated by prior opioid administration. Adrenal suppression occurs with multiple doses or infusion, and steroid supplementation may be required.
Rapid-Sequence Induction
Patients with a full stomach are at increased risk for the aspiration of stomach contents during the induction of anesthesia. Patients who require full-stomach precautions include those who have not fasted for an adequate period, those with gastrointestinal obstruction, trauma patients whose gastric motility is depressed, or those who are at a mechanical predisposition for aspiration (e.g., patients with ascites). It is mandatory that these patients have an IV induction. Patients are preoxygenated with 100% oxygen by mask and are then given an induction dose of a hypnotic and a rapid-acting muscle relaxant—either succinylcholine (2 mg/kg) or rocuronium (1.2 mg/kg). Application of manual pressure over the cricoid, known as the Sellick maneuver, can be performed in an attempt to seal the esophagus and to prevent passive regurgitation. Pressure is not released until the endotracheal tube is in place, proper position is confirmed, and the cuff, if necessary, is inflated. At the end of the procedure, these patients should be allowed to emerge completely before extubation to ensure adequate airway protection.
Intramuscular Induction
Ketamine is a derivative of phencyclidine with potent analgesic and amnestic properties. Anesthetic induction can be achieved with the recommended dose of 1 to 3 mg/kg intravenously or 5 to 10 mg/kg intramuscularly. As noted previously, ketamine is very useful as an intramuscular induction agent in children who are combative or unable to cooperate with a standard inhalation or IV induction, often because of intellectual disabilities and behavioral disorders. Ketamine is an excellent choice for anesthetic induction in hypovolemic patients because of its sympathomimetic properties; it rarely causes hypotension in these patients.
Ketamine tends to produce copious secretions and should be accompanied by the administration of atropine or glycopyrrolate. Nystagmus and diplopia are common side effects and will resolve as the drug is cleared from the patient’s circulation. Intraoperative and postoperative dreams and hallucinations have been reported, more often in older than in younger children and less often when other sedative-hypnotic agents are used in conjunction with ketamine. If this agent is given preoperatively with the parents still present, they should be informed to expect these side effects.
Anesthesia Maintenance
A variety of agents may be used to maintain anesthesia in the pediatric population. The otolaryngologist should be familiar with the effects of each of these.
Inhalational Agents
Nitrous Oxide
Nitrous oxide is a widely used inhalational induction agent in children. Its popularity is a result of its low solubility, which results in rapid uptake and distribution. Nitrous oxide also is odorless and does not cause cardiovascular depression. Although nitrous oxide allows the more rapid uptake of lower concentrations of other anesthetic agents, nitrous oxide itself is not a very potent anesthetic agent. Consequently, it must be delivered in high concentrations to have an analgesic and hypnotic effect, thereby limiting the concentration of oxygen that can be delivered. It is not useful for patients who require high concentrations of oxygen and should be avoided in patients with pulmonary hypertension because it may aggravate it. Because nitrous oxide is less potent and is implicated in an increased incidence of postoperative nausea and vomiting (PONV), its use for anesthetic maintenance has decreased. A 34-fold difference exists in the blood-gas coefficients of nitrogen (0.013) and nitrous oxide (0.46); thus, nitrous oxide will enter air-filled cavities faster than nitrogen can leave. In a fixed cavity such as the middle ear, the result is an increase in pressure. During tympanoplasty, the middle ear pressure generated by nitrous oxide can lift off the tympanic membrane graft, and it is therefore usually avoided entirely during those procedures. Nitrous oxide use also may present a hazard for patients with previous reconstructive middle ear surgery. Finally, nitrous oxide is flammable and should be avoided when fire is a risk, such as during tonsillectomy or with use of a laser in the airway.
Sevoflurane
Sevoflurane has completely taken the place of halothane in pediatric institutions in the United States. It is a valuable addition to the anesthesiologist’s armamentarium for several reasons. First, it is less pungent than halothane, and it is even better tolerated during inhalation induction. Second, it has a blood-gas partition coefficient similar to that of nitrous oxide, so induction and emergence times are shorter than with halothane. Third, it has fewer cardiovascular effects than halothane.
Sevoflurane has several disadvantages. Its offset is so rapid that without adjunctive administration of narcotics, the perception of and response to pain may be accentuated. There are reports in the literature of a significant incidence of emergence excitement associated with cases in which sevoflurane was used. For these reasons, sevoflurane has assumed a role as an induction agent and a maintenance agent for very short procedures, but it is often replaced by isoflurane or desflurane after induction, depending on case type and anesthesiologist preference. Like all halogenated inhalational agents, it is a potential trigger agent for malignant hyperthermia and should be avoided in susceptible patients.
Isoflurane
Isoflurane has been used for many years as a standard maintenance inhalational agent for children and adults. In practice, it offers no clear advantage for the pediatric otolaryngology patient. It is more pungent and not nearly as well accepted by children during inhalation induction as sevoflurane. Isoflurane does increase, rather than decrease, heart rate. It also causes decreases in blood pressure via peripheral vasodilation.
Desflurane
Desflurane is the newest inhalational agent to be introduced into clinical practice. Its principal advantage is its quicker time to recovery compared with sevoflurane. It appears to be well tolerated as a maintenance anesthetic because it provides stable hemodynamics and respiratory parameters during its use. Similar to sevoflurane, desflurane has been associated with increased agitation and emergence delirium. However, this effect does not seem to occur frequently enough to justify avoiding its use in children.
Desflurane does have an irritant effect on the airway that makes it contraindicated for inhalational induction or for use during airway procedures such as bronchoscopy. However, many centers have adopted a practice of inducing anesthesia with sevoflurane and then switching to desflurane to take advantage of its quick recovery time while avoiding its airway effects during induction. It also has been suggested that this agent may be particularly useful in neonates and formerly premature babies, in whom residual anesthesia effects may increase the risk of apnea postoperatively.
Intravenous Agents
Intravenous agents include the various opioids and other agents. The otolaryngologists should be familiar with their effects in the pediatric population.
Opioids
Fentanyl.
Fentanyl is a potent synthetic opioid and is the most commonly used supplement to anesthesia in children. It has a relatively short duration of action (1 to 2 hr), and is about 100 times as potent as morphine. Fentanyl is ideal for patients who need short-term anesthesia and analgesia. It is useful for patients who require rapid recovery to baseline ventilatory function and respiratory drive, such as patients having same-day surgery, neurosurgical procedures, and airway instrumentation. IV infusion doses that range from 0.5 to 4 mµg/kg/hr infusions provide adequate analgesia and sedation and can be used as adjuncts for maintenance of anesthesia. Fentanyl doses in excess of 100 µg/kg have been given to cardiac surgery patients with minimal cardiovascular depression. An initial peripheral vasodilation occurs, but tachyphylaxis to this side effect occurs with additional dosing. Preterm and full-term newborns have variable and prolonged clearance, probably related to reduced hepatic blood flow. In addition, they are extremely sensitive to the effects of fentanyl on chest wall rigidity. In neonatal patients who are not intubated, fentanyl should be given only in small increments.
The effect of low-dose fentanyl is terminated largely by redistribution and thereby results in a rapid reduction in clinical effect. However, higher or repeated doses will result in drug accumulation, because fentanyl is highly lipophilic. Clearance then becomes dependent on metabolism, and the clinical effect, including respiratory depression, may last for hours. Chest wall rigidity has been reported after rapid fentanyl administration, although the etiology of this problem is unclear. Bradycardia may occur as a result of increased vagal tone, especially when given with other agents that may have a similar effect.
Morphine Sulfate.
Overall, morphine is the most frequently used opioid in children. It can be used in the OR to supplement inhalational agents and to provide postoperative analgesia. The usual IV dose is 0.05 to 0.1 mg/kg as part of a balanced anesthetic technique, although higher doses may be used for a narcotic-based technique. The dose may need to be reduced in critically ill children or young infants. The half-life after IV administration is approximately 3 hours in older children but is significantly longer in infants as a result of diminished clearance. The major side effect is respiratory depression, which results in diminished minute ventilation with a greater effect on respiratory rate than tidal volume. Many believe that newborns are at higher risk than older children for respiratory depression. Infusions of morphine at rates of 10 to 30 µg/kg/hr have been described in small infants without significant respiratory depression. Appropriate monitoring for apnea should occur in premature infants and those neonates with a history of apnea and bradycardia. Histamine release is also common with the administration of morphine, and it most commonly results in a localized or generalized rash. Bronchospasm and hypotension have been reported, but they are much less common.
Hydromorphone.
Hydromorphone has become a commonly used alternative to morphine. It is approximately five to seven times more potent than morphine, and the usual IV dose ranges from 0.015 to 0.02 mg/kg. Its half-life is similar to that of morphine, and it has a similar duration of action. Hydromorphone may be useful in patients with renal failure because its metabolic by-products are less active than those of morphine. In addition, hydromorphone may be a good alternative for those patients who suffer side effects (e.g., itching, nausea, hallucinations) with morphine. As with other opioids, respiratory depression is the most concerning side effect, but it is rare with appropriate dosing.
Meperidine.
The use of meperidine has dramatically decreased because of its unique side effect profile and the availability of equianalgesic alternatives. The principal metabolite of meperidine is normeperidine, a compound that can cause neurologic excitation manifested as tremors, irritability, or seizures. This side effect is especially pronounced with prolonged use or in patients with hepatic or renal dysfunction. Meperidine’s superiority over other opioid agonists in the treatment of postoperative shivering probably is related to its κ-receptor activity, and it is the one reason that it has not become entirely obsolete.
Alfentanil.
As a short-acting analog of fentanyl, alfentanil is commonly used for short outpatient procedures. It is approximately one fourth as potent as fentanyl and has one third the duration of action. It has a very fast onset of action (1 min) and a short elimination half-time (1.5 hr). It is less lipophilic than fentanyl, and the dose range is 10 to 20 µg/kg given intravenously. Renal failure does not alter the clearance of alfentanil. Beside the opioid-related side effects, thorax rigidity and bradycardia may be seen.
Remifentanil.
Remifentanil has the same analgesic potency as that of fentanyl. The key feature of remifentanil is the ester side chain hydrolysis by blood and tissue esterases, which results in rapid metabolism (elimination half-time is 10 to 20 min). This results in zero order kinetics, meaning that its offset of action is 10 to 20 minutes after discontinuation of the infusion regardless of the duration of the infusion; in other words, it does not accumulate. Remifentanil does have a rapid onset, and because of its short duration of action, it usually needs to be administered as a continuous IV infusion at the rate of 0.05 to 1 µg/kg/min for neonates and up to 2 µg/kg/min for older children. Pharmacokinetic parameters of remifentanil are unchanged by hepatic or renal disease. Disadvantages are the high cost and the lack of postoperative analgesia, which can be overcome with application of longer-acting opioids at the end of surgery ( Table 3-3 ).
Drug | Order of Potency | Onset of Action (min) | Duration of Action | Routes of Administration | Drug Doses | |
---|---|---|---|---|---|---|
Adjunct to GA (IV) | Postop. Pain (IV) | |||||
Fentanyl | 150× | 6.8 | Shorter than morphine if single dose, (but due to redistribution is most prolonged if continuous IV) | IV, IM, intranasal, OTFC, transdermal patch | 2 to 10 µg/kg | 1 µg/kg |
Sufentanil | 1000 to 1500× | 6.2 | Similar to fentanyl | IV, intranasal | 0.5 to 1.5 µg/kg/min | — |
Remifentanil | 300× | 1-2 | Very short (6 to 8 min offset) | IV | 0.025 to 2 µg/kg/min | — |
Alfentanil | 20 to 40× | 1.5 | One third of fentanyl | IV | 0.5 to 3 µg/kg/min | — |
Morphine | 1 | 15-30 | 3 to 4 h | IV, IM, SC, PO | 0.05 to 0.1 mg/kg | 0.05 to 0.1 mg/kg |
Hydromorphone | 5 to 10× | 10-20 | 2 to 4 h | IV, PO | 0.015 to .020 mg/kg | 0.005 to 0.015 mg/kg |
Methadone | 1 | 15-30 | 12 to 50 h | IV, PO | 0.1 mg/kg | 0.05 to 0.1 mg/kg |
Ketamine
Ketamine is a versatile nonbarbiturate drug that dissociates the thalamus from the limbic cortex, thus producing a cataleptic state of dissociative anesthesia. The patient is noncommunicative, and the eyes remain open with a slow nystagmic gaze. Varying degrees of hypertonic and purposeful movements occur independent of stimuli.
Ketamine rapidly induces general anesthesia within 0.5 to 2.0 minutes when given intravenously in doses of 0.5 to 2 mg/kg. Further anesthesia can be maintained with infusions of 0.01 to 0.1 mg/kg/min. It is also well absorbed following oral (3 to 6 mg/kg), rectal (4 to 6 mg/kg), nasal (4 to 6 mg/kg), and intramuscular administration. The onset of action may be delayed with these routes from 10 to 35 minutes. Administration of subanesthetic doses produces intense analgesia, amnesia, and altered consciousness. These properties make ketamine an ideal agent for painful procedures, particularly in children with congenital heart disease, asthma, trauma, hemodynamic instability, burns, and poor IV access.
Ketamine increases arterial blood pressure, heart rate, and cardiac output. It also increases cerebral oxygen consumption, cerebral blood flow, and intracranial pressure. It does not produce significant respiratory depression in low doses, and the upper airway and laryngeal airway reflexes remain intact, and the ventilatory response to hypoxia and hypercarbia are maintained. Ketamine further increases circulating catecholamines and causes the relaxation of tracheal, bronchial, and alveolar smooth muscle, making it the IV induction agent of choice in patients with reactive airways. Additionally, ketamine also raises the intraocular pressure and is associated with increased incidence of emergence delirium and nausea and vomiting. It is metabolized extensively by hepatic microsomal enzymes and its active metabolite, norketamine, may contribute to the prolonged effects of ketamine.
Dexmedetomidine
Dexmedetomidine is the most recently approved agent by the Food and Drug Administration (FDA) for anesthesia and sedation. It is a selective α-2 agonist with sedative, anxiolytic, and analgesic properties. It has a short distribution half-life of 6 minutes and an elimination half-life of 2 hours. It is metabolized in the liver, eliminated by the kidneys, and is 93% protein bound.
Dexmedetomidine is a centrally acting agent that produces sedation and anxiolysis via activation of inhibitory γ-aminobutyric acid neurons. Specifically, it decreases central sympathetic activity, and increases central parasympathetic outflow from the locus ceruleus. Being highly lipid-soluble, it quickly crosses the blood-brain barrier. In a dose-dependent manner, it decreases heart rate and mean arterial pressure. The effects of sympatholysis cause peripheral vasodilation and hypotension at lower doses and vasoconstriction and hypertension at higher doses.
Dexmedetomidine provides respiratory stability and minimal ventilatory depression, which distinguishes it from opioids, benzodiazepines, and other hypnotic agents. In spontaneously ventilating patients, the respiratory rate, carbon dioxide tension, and oxygen saturation are generally maintained despite some blunting of the carbon dioxide response curve. Analgesia is another useful property of the drug, which exerts its effect on the dorsal horn of the spinal cord and the supraspinal and peripheral nerves, thereby inhibiting the release of substance P. When used with other opioids, it potentiates their analgesic effects. Finally, in decreasing sympathetic tone, dexmedetomidine attenuates the stress response. IV dosing is by loading 1 µg/kg infused over 10 minutes to minimize hypertensive effects, followed by infusing 0.5 to 1 µg/kg/hr. Additional possible routes of administration include intramuscular, intranasal, buccal, and oral.
For a relatively new drug, dexmedetomidine has found a plethora of uses. Given its synergy with hypnotics and opioids, it reduces the overall doses of sedation drugs and decreases long-term exposure in mechanically ventilated patients. In neonates and children, dexmedetomidine is frequently used for invasive and noninvasive procedural sedation and as an anesthetic adjunct during surgery. Its analgesic properties make it useful during airway stimulating procedures such as debridement of burns, bronchoscopy, laryngoscopy, and tonsillectomy among others. Dexmedetomidine provides an interesting quality of sedation that permits arousal with gentle stimulation. This subtle wake-up allows for an agitation-free emergence from anesthesia. In the treatment of anesthetic emergence delirium, a dose of 0.3 µg/kg has been shown to be very effective. In patients with opioid tolerance, it facilitates in the weaning of opioids relatively quickly with minimal hemodynamic consequences. Other indications such as treatment of shivering, chronic regional pain syndrome, and withdrawal continue to emerge. The side effect of bradycardia makes it contraindicated for patients on digoxin and unsuitable as a sedative for electrophysiologic studies. It should be used with caution in children with preexisting bradycardia, atrioventricular conduction defects, hypertension, and decreased cardiac output.
Muscle Relaxants
Succinylcholine.
Succinylcholine is the only depolarizing muscle relaxant. With the advent of intermediate-acting nondepolarizing relaxants, the use of succinylcholine for routine surgical procedures is declining, primarily as a result of side effects that relate to its depolarizing mode of action (see below). However, it remains the most rapid acting of all muscle relaxants, and it is still indicated for use in rapid-sequence inductions and in the treatment of laryngospasm. IV administration of 1.5 to 2.0 mg/kg achieves 95% twitch depression in 40 seconds and results in excellent intubating conditions. Succinylcholine also can be given intramuscularly (4 to 5 mg/kg) if the IV route is unavailable, although the clinical effect is delayed in onset, depending on perfusion to the area of deposition.
The side effects of succinylcholine are numerous. Some degree of increase in masseter muscle tone is common and, in some cases, is extreme enough to mimic true trismus. This is an important distinction to make because as many as 50% of patients with trismus after succinylcholine are biopsy positive for susceptibility to malignant hyperthermia. Succinylcholine can also cause bradycardia as a result of an increase in vagal tone, an effect that is especially prominent in younger children and infants. The decline in heart rate usually is transient; if persistent, it is responsive to IV atropine, and occasionally, asystole is seen. Hyperkalemia can be seen as a result of depolarization of the myoneural junction, and increases of serum potassium of 0.5 mEq/L occur even in normal children. Life-threatening hyperkalemia after succinylcholine administration can occur in children with burns, tetanus, paraplegia, encephalitis, crush injuries, and neuromuscular disease. Myoglobinemia occurs in approximately 40% of children anesthetized with halothane who are given succinylcholine. This effect can be partially attenuated with a previous dose of a nondepolarizing agent to prevent fasciculations. A series of cardiac arrests were reported in boys who were given succinylcholine, with subsequent development of massive muscle breakdown and potassium release. The presumed etiology was previously undiagnosed muscular dystrophies. In 1994, in response to these reports, the manufacturer placed a warning on the package insert cautioning the practitioner about the routine use of succinylcholine. As a result, many pediatric anesthesiologists have abandoned the routine use of succinylcholine except for rapid-sequence inductions and to treat laryngospasm.
Of note, 90% of an IV dose of succinylcholine is rapidly hydrolyzed in the plasma by pseudocholinesterase. Patients with deficient or reduced levels of pseudocholinesterase exhibit a prolonged effect from succinylcholine.
Nondepolarizing Muscle Relaxants.
A variety of nondepolarizing muscle relaxants are available. These agents vary in dose, speed of onset, duration of action, and side effects. As shown in Table 3-4 , the pharmacologic properties of these drugs may be different in infants from those in older children. The choice generally is based on the duration of the surgical procedure. As noted, rocuronium has been shown to produce good intubating conditions but with a slightly longer onset of action (less than 1 min) than with succinylcholine (less than 30 sec).
Intubating Dose (mg/kg) | Onset Times * (min) | Recovery Times in Infants (min) | ||
---|---|---|---|---|
Adults | Children | |||
Atracurium | 0.5 | 0.5 | 2 | 40-60 |
Cisatracurium | 0.1 | 0.1-0.2 | 2.5 | 53 |
Vecuronium | 0.07-0.1 | 0.1 | 2.4 | 35 |
Rocuronium | 0.5-1.0 | 0.6-1.2 | 1.3 | 42 |
Pancuronium | 0.1 | 0.1 | 2.5 | 50 |
Reversal Agents of Neuromuscular Relaxants
Sugammadex
Sugammadex is a biologically inactive neuromuscular reversal drug with a radically new mechanism of action. It is a modified γ-cyclodextrin and exerts its effect by forming very tight water-soluble complexes at a 1 : 1 ratio with steroidal neuromuscular blocking drugs like rocuronium, pancuronium, and vecuronium. It then directly removes these complexes from the neuromuscular junction.
Prior to sugammadex, only two options—succinylcholine and rocuronium—were available to facilitate tracheal intubation during a rapid-sequence induction. Relaxation with succinylcholine carries concomitant risks, including that of hyperkalemic arrest. However, a high dose of rocuronium is needed for rapid-sequence inductions, and it cannot be immediately reversed. In the case of a difficult airway, in which the patient cannot be ventilated after administration of rocuronium, there would be no way to back out of the anesthetic and return the patient to spontaneous ventilation in a life-sustaining amount of time. At least 30 minutes is required before neostigmine can be administered, and anticholinergics must also be administered because neostigmine is an anticholinesterase inhibitor and indirectly increases the activity of the cholinergic system. Thus, the patient must be subjected to the hemodynamic side effects of both neostigmine and anticholinergics.
During rocuronium-induced neuromuscular blockade, the IV administration of sugammadex creates a concentration gradient that favors the movement of rocuronium molecules from the neuromuscular junction back into the plasma. This results in a fast recovery of neuromuscular function and rapid termination of the block. Sugammadex administered 3 to 5 minutes after rapid-sequence induction with rocuronium produces markedly faster recovery than placebo or spontaneous recovery from succinylcholine-induced block.
The recommended dose of sugammadex depends on the level of neuromuscular blockade to be reversed. Different doses (2, 4, and 16 mg/kg) are available to reverse different levels of neuromuscular blocks. A dose of 2 mg/kg is indicated for the reversal of shallow neuromuscular blockade, whereas 4 mg/kg is indicated for the reversal of profound neuromuscular blockade by rocuronium. Reversal and return of train of four of 0.9 is likely within 1.5 minutes of the shallow block and within 3.3 minutes of the profound block. Immediate reversal of neuromuscular blockade by high-dose rocuronium requires sugammadex at a dose of 16 mg/kg, and return of train of four of 0.9 is likely within 5.7 to 6.7 minutes. The speed of recovery is dose dependent, and the reversal is sustained without any signs of return of paralysis. Sugammadex 4 mg/kg IV reverses rocuronium neuromuscular blockade more rapidly than neostigmine or edrophonium.
Sugammadex is safe and well tolerated, and it does not bind to plasma proteins. It is excreted through the kidneys and enhances the renal excretion of rocuronium. Its efficacy as an antagonist does not appear to rely on renal excretion of the cyclodextrin-relaxant complex.
The most frequently reported and anesthetically concerning side effects have been hypersensitivity allergic reaction, anaphylaxis, hypotension, and prolongation of the corrected QT interval. In view of the potential of sugammadex to reverse even a profound neuromuscular block, as well as its favorable safety profile, this agent may fulfill the criteria of an ideal reversal agent for rocuronium.
Total Intravenous Anesthesia
Total intravenous anesthesia (TIVA) is a continuous titration of IV drugs for maintenance of anesthesia. Drugs are used in some combination of propofol, remifentanyl, alfentanil, fentanyl, ketamine, and midazolam. This technique provides safe anesthesia in the malignant hyperthermia–susceptible patient. It is often used in patients with severe PONV and is also the technique of choice in some airway cases.
Emergence from Anesthesia
The decision of whether to extubate a patient while he or she is awake or under surgical planes of anesthesia (deep extubation) depends on a number of factors. Deep extubations are smoother and facilitate rapid turnover. Considerations in making this decision include the patient’s age, airway anatomy, underlying disease, the presence of blood or secretions in the airway at the time of extubation, the presence of a full stomach preoperatively, the ability of the postanesthesia care unit staff to manage an obstructed airway, and the immediate availability of an individual skilled in reintubation should it be required. Deep extubation is preferred in patients with asthma or in those for whom coughing would be contraindicated. Factors that favor awake extubation include a full stomach at the start of the procedure, age younger than 6 months, difficult airway anatomy for either a mask or intubation, copious blood or secretions in the airway, and a predisposition to apnea. Systematic comparisons of awake versus deep extubation have revealed that patients extubated while awake have lower oxygen saturations, probably because of coughing during emergence, but there is no difference in overall morbidity or mortality. Either technique can be performed safely; the anesthesiologist should have both approaches in his or her armamentarium and, using clinical judgment, should choose the one that best fits the situation.
Emergence delirium is an abnormal condition of a dissociated state of consciousness and increased psychomotor activity that occurs after administration of inhalational anesthetics in children. Presentation ranges from involuntary jerking movements with muscular hypertonia of the trunk and extremities to thrashing, disorientation, crying, screaming, inconsolability, and inability to recognize the surroundings. The incidence is approximately 13%, although there is quite a range in different studies. It is more frequent in younger children.
The exact etiology of the restlessness and agitation is still uncertain; thus no preventative treatment has been found. Although emergence delirium is an unpleasant condition, it is generally self-limiting within 5 to 15 minutes. Treatment with sedatives or analgesics may potentially prolong recovery and time until discharge of the patient. Variable success has been reported with analgesics, opioids, benzodiazepines, clonidine, ketorolac, midazolam, propofol, thiopental, and dexmedetomidine. Although no clinical evidence suggests that emergence delirium has long-term behavioral effects, it is a cause of dissatisfaction for most parents and caregivers.
Postoperative Management
Nausea and Vomiting
Nausea and vomiting can result in an unpleasant recovery from surgery and anesthesia, a delayed discharge from the postanesthesia recovery unit, and an increase in overall cost. In the pediatric population, the incidence is variable and depends on the type of surgery, the anesthetic used, gender, age, history of motion sickness, and adequacy of hydration. Although the overall incidence is lower in children younger than 2 years of age, children older than 3 years have an average vomiting incidence of more than 40%. Procedures such as tonsillectomy and middle ear surgery may be associated with an incidence of vomiting as high as 70%. The use of narcotics, halogenated inhalational agents, and perhaps nitrous oxide increases the risk of nausea and vomiting. By contrast, propofol reduces the incidence of vomiting compared with halothane in children undergoing adenotonsillectomy.
Consequently, antiemetics often are given prophylactically for children at risk for PONV, and a multimodal approach has been shown to be the most effective one. The use of IV ondansetron (50 to 100 µg/kg up to 4 mg), IV dexamethasone (150 to 500 µg/kg up to 8 mg), and IV droperidol (50 to 75 µg/kg up to 1.25 mg) is evidence-based as a prophylaxis for patients at risk for PONV and for treatment of patients with PONV.
Serotonin (5-hydroxytryptamine 3 ) receptor antagonists such as ondansetron have shown efficacy and safety in prophylaxis and treatment of PONV, with a greater antiemetic than antinausea effect. When prophylaxis with a serotonin receptor antagonists fails, rescue therapy with serotonin receptor antagonists is not recommended within the first 6 hours of surgery because it confers no additional benefit.
Another drug that has been shown to help control PONV during the 24 hours after surgery is dexamethasone. Children who receive a single intraoperative dose of dexamethasone were two times less likely to vomit during the first 24 hours than children who received placebo. In other words, routine use in four children would be expected to result in posttonsillectomy emesis experienced by one fewer patient. Additionally, children who received dexamethasone were more likely to advance to a soft to solid diet on posttonsillectomy day 1 than were those children who received placebo.
Droperidol is an antidopaminergic antiemetic. Although effective in the treatment of PONV, its sedating and extrapyramidal side effects should be considered. In 2001, the FDA issued a black box warning against the use of this drug because of concerns of serious cardiac arrhythmias secondary to QT prolongation. An overwhelming majority of anesthesiologists surveyed (92%) did not consider the ban warranted because torsades de pointes was seen mostly when the drug was administered in very high doses or when there were confounding factors. Still, prudence would limit its use to cases in which patients do not have underlying cardiac disease, and when other classes of antiemetics have been exhausted; the maximum total pediatric dose is less than 100 µg/kg or 2.5 mg total.
Finally, an inexpensive but effective method of reducing the incidence of nausea and vomiting is to withhold oral fluids during the postoperative period until the child asks for them because forced oral fluids increase the incidence of vomiting and delay discharge. This can be safely done by generously hydrating the patient with IV fluids intraoperatively. Nontraditional treatment methods such as acupressure are being studied and thus far confirm their effectiveness in the prevention of PONV; they are useful adjuncts, especially in view of the total absence of side effects.
Postintubation Croup
In children up to approximately 8 years of age, the subglottis is the narrowest portion of the airway, and it may be the source of postoperative airway obstruction secondary to mucosal swelling. A reduction in the reported incidence of postintubation croup from 1% to 0.1% may be obtained with use of proper-sized, non–tissue-reactive tubes that allow an air leak around the tube at 25 cm H 2 O or less.
Racemic epinephrine (0.5 mL of 2.25% in 2.5 to 3.0 mL of normal saline for children weighing 15 kg or more) nebulized in oxygen is the management of choice for postintubation croup; the effect is thought to result from the vasoconstriction of the subglottic mucosa. (The dose varies and ranges from 0.2 mL for infants weighing less than 5 kg, 0.3 mL for 5- to 10-kg infants, and 0.4 mL for 10- to 15-kg infants.) Because symptoms can recur within 2 to 4 hours, the child should be observed for 4 hours after treatment before being sent home. If frequent and repeated treatments are necessary, the patient should be admitted. Dexamethasone (0.3 to 0.5 mg/kg) also may be used, although its effect is slow in onset, if it occurs at all.
Pain Management
The theory that children, particularly neonates, perceive pain less acutely and therefore do not need pain medication is no longer accepted. As a result, since the early 1990s, there has been a significant change in how pediatric pain is approached. Perhaps the most powerful sign of this change was that the Joint Commission (formerly the Joint Commission on Accreditation of Healthcare Organizations) added pain management to its accreditation review in 2000. Areas that now must be addressed by accredited institutions include pain assessment, pain treatment, and education about pain management.
Pain assessment can be challenging in children. Assessing the degree of pain in preverbal children is problematic, although several observational scales have been developed to assist with this population. Children 4 to 8 years of age have been shown to be capable of giving a self-report with simplified tools, and older children can use the same scales as those for adults. Patients in the intensive care unit setting (immediately postoperatively) and cognitively impaired children remain populations to be studied in the area of pain assessment.
IV opioids—whether given by bolus, infusion, or patient-controlled analgesia—are the mainstay of therapy for severe pain. Children 8 years of age and older and some mature 6- to 7-year-old children do well with patient-controlled analgesia, tend to use less total drug, and have a better feeling of control than with traditional methods of administration. Younger children who require IV opioids may benefit from administration accomplished using parent- or nurse-controlled analgesia. Appropriate parent education is a necessary prerequisite to parent-controlled analgesia.
Milder degrees of pain can be handled with nonopioid analgesics. Acetaminophen (10 to 15 mg/kg q4-6h to a maximum 4 g q24h), alone or with oxycodone (0.1 to 0.15 mg/kg), is useful for children who can take oral medications. Rectal acetaminophen can also be given, although doses of 20 to 40 mg/kg are necessary to achieve serum levels equivalent to those obtained with 10 to 15 mg/kg given orally. IV acetaminophen (15 mg/kg q6h to a maximum daily dose of 75 mg/kg) has been newly approved for children older than 2 years and has been shown to be effective and safe. Ketorolac (0.5 mg/kg IM or IV q6h up to 48 hr) is a nonsteroidal antiinflammatory drug that has potent analgesic properties; these may be equivalent to those of morphine but without the respiratory depression or nausea associated with opioids. However, ketorolac requires 30 to 45 minutes for onset, has a 12% incidence of nausea, and may be associated with a higher incidence of bleeding because of effects on platelet function. Nonopioid analgesics also are a good adjunct to opioid and other pain medications, although multimodal therapy has been shown to be most effective in the treatment of pain, especially in difficult-to-treat cases.
Codeine, often used as a first-line oral opioid, seems to be a weak analgesic. Essentially a prodrug of morphine, it needs to be converted to its active form in the liver. Approximately 10% of the population lack the enzyme CYP2D6 and will not achieve pain relief from codeine. Accordingly, a more rational approach is to use a stronger oral analgesic as a first-line agent, especially after significantly painful procedures. Some children appear to be ultrarapid metabolizers of codeine, which is an inherited (genetic) ability that causes the liver to convert codeine into life-threatening or fatal amounts of morphine in the body. Consequently, a new Boxed Warning , FDA’s strongest warning, has been added to the drug label of codeine-containing products about the risk of codeine in postoperative pain management in children following tonsillectomy and/or adenoidectomy. As with all opioids, nausea and vomiting are potential side effects and may be more prevalent with use of codeine.
Sedation and Anesthesia Outside of the Operating Room
Over the past 10 years, an increasing number of procedures have been performed outside of the OR. Physician time constraints, reimbursement changes, new technology for procedures that cannot be done in the OR, and the development of new anesthetic agents have all contributed to this development. However, the risk of adverse events is potentially greater than that for OR procedures as a result of monitoring variation, poor presedation evaluation, inadequate training, and lack of adequate recovery procedures. More recently, a greater degree of scrutiny has been given to the practice of sedation for children outside of the OR. In an effort to improve safety, the Joint Commission has been instrumental in forcing institutions to develop policies to improve the care of children and adults who are receiving sedation as part of overall accreditation efforts. In addition, specialty organizations have developed policy statements about this topic. As a result, in many institutions, pediatric anesthesiologists are more directly involved with sedation outside of the OR than in the past.
A large number of procedures that are performed in hospitals may require use of sedation or analgesia, especially in children. Painful procedures such as lumbar puncture, bone marrow biopsy, and chest tube placement are obvious examples. Other procedures, such as magnetic resonance imaging or gastrointestinal endoscopy, also may require sedation or anesthesia because of the distressing nature of one or more aspects of the examination or the need for immobility. In the context of otolaryngology, the most common candidates for out-of-the-OR or clinic procedures may be the removal of pressure equalization tubes, nasal ciliary biopsy, fine-needle aspiration, or the removal of sutures. One report suggests that these procedures can be performed in the clinic safely and with less cost using IV sedation compared with general anesthesia in the OR.
In general, at children’s hospitals, a pediatric sedation service oversees sedation or anesthesia for a majority of out-of-the-OR procedures. All children scheduled for procedures receive a phone call before their appointment to identify any significant medical problems and to provide nil per os (NPO) instructions. On the day of the procedure, the patient arrives approximately 30 minutes before the planned time of the procedure for application of topical anesthesia cream. A sedation nurse then starts an IV line, after which the pediatric anesthesiologist provides IV sedation or anesthesia. After the procedure is completed, the sedation nurse oversees the patient’s recovery, until the patient is ready for discharge. For less invasive procedures in cooperative patients, the sedation nurse may provide conscious sedation with oral, IV, or intranasal medications rather than the method described previously.
Another option is for the otolaryngologist to provide the sedation, often with oral or IV midazolam, possibly combined with fentanyl. Even with this type of conscious sedation, practitioners should be aware of their institution’s policy about sedation. A preprocedure evaluation should be documented, monitoring should be performed during the procedure and documented, and appropriate recovery procedures should be followed. Documentation of NPO status also may be relevant, depending on the depth of sedation planned.
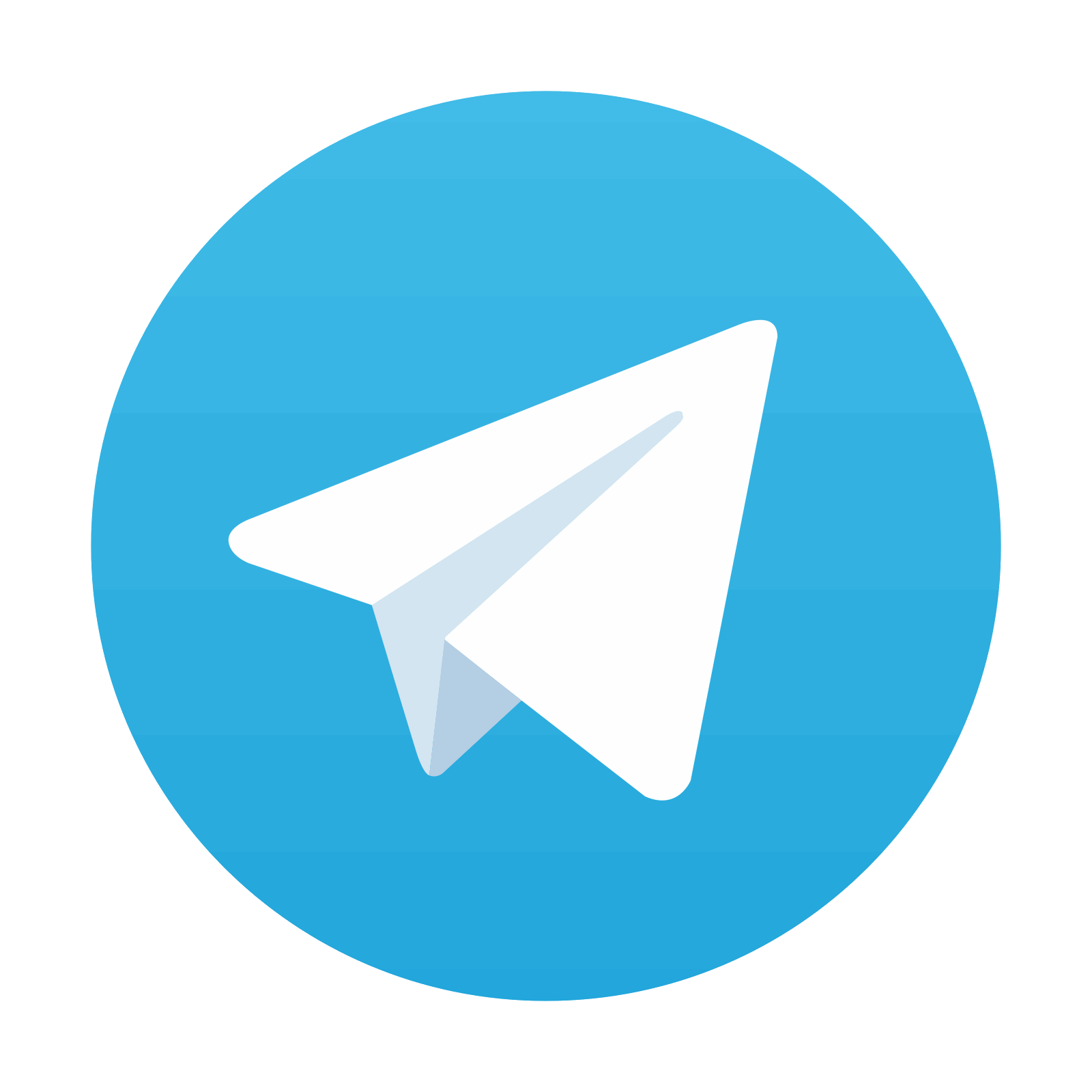
Stay updated, free articles. Join our Telegram channel

Full access? Get Clinical Tree
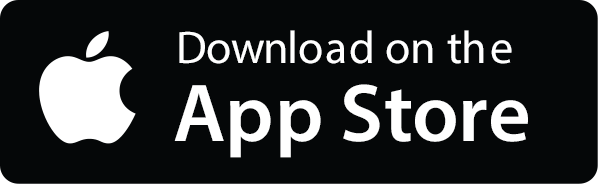
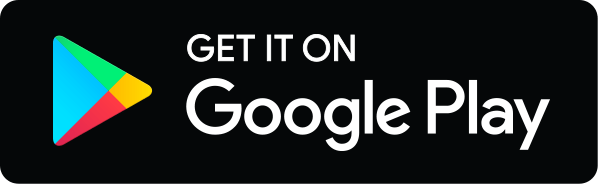