Purpose
To study effects of age and horizontal duction on deformation of the optic nerve head (ONH) and peripapillary retina (PPR), as reflected by displacement of vascular landmarks, to explore the influence of adduction tethering.
Design
Cross-sectional study.
Methods
Setting : University. Study Population : Single eyes of 20 healthy young adults (average age 23.9 ± 3.9 [SD] years) were compared to 20 older subjects (average age 61.4 ± 9.3 years). Observational Procedure : The disc and PPR were imaged by scanning laser ophthalmoscopy in central gaze and at 35 degrees abduction and adduction. Main Outcome Measure : Deformations of the disc and adjacent PPR were measured by comparing positions of epipapillary and epiretinal blood vessels.
Results
Vessels within the ONH of younger subjects shifted temporally during adduction and nasally during abduction. Displacement of the nasal hemi-disc in adduction was greater at 38.5 ± 1.7 μm (standard error of mean) than the temporal half at 4.1 ± 2.1 μm ( P < .001). PPR within 1 radius of the disc margin underwent 7.6 ± 1.6 μm average temporal displacement in adduction in young subjects. In abduction, the young temporal hemi-disc shifted 4.4 ± 0.6 μm nasally without significant displacement in the nasal half. Older subjects’ ONH showed less temporal shift and less displacement in the PPR within 1 disc radius ( P < .0001) in adduction; the nasal hemi-disc shifted 24.5 ± 1.3 μm compared with 4.4 ± 2.1 μm in the temporal half. There were no significant deformations of the disc during abduction by older subjects.
Conclusion
Large horizontal duction, particularly adduction, deforms the disc and peripapillary vasculature. This deformation, which is larger in younger than older subjects, may be due to optic nerve tethering in adduction.
When the human eye rotates into large angle adduction, magnetic resonance imaging (MRI) demonstrates that the optic nerve (ON) and the optic nerve dural sheath straighten, become tethered, and exert traction on the posterior globe. , Resulting anteroposterior deformations of posterior ocular tissues during horizontal duction have been demonstrated by 2-dimensional optical coherence tomography (OCT), including anteroposterior tilting of the ON head (ONH) and peripapillary Bruch membrane. The nasal side of the ONH moves anteriorly while the temporal side moves posteriorly. , The effect is greatly enhanced with adduction exceeding a threshold of about 26 degrees. Saccades of 25-45 degrees are common during everyday eye movement and can rotate the eyes beyond this threshold. Gaze shifts with head rotation include eye movements averaging ∼30 degrees. , In abduction, the ONH also exhibits a see-saw motion directionally inverse to abduction. , Though both abduction and adduction can deform the ONH, the effect is greater for adduction, presumably owing to ON tethering. This is likely why our previous MRI study found no optic nerve tethering in exotropia, but higher occurrence in esotropia.
Biomechanical studies of stress and strain in the posterior eye using finite element analysis have focused on the structural properties of the ONH, peripapillary sclera, and lamina cribrosa to suggest possible mechanisms of glaucoma independent of intraocular pressure (IOP). Elevated IOP can of course produce mechanical stress in the ONH, , , yet IOP alone cannot explain glaucomatous optic neuropathy in many patients with normal or subnormal IOP that accounts for a majority of glaucoma cases in Asian populations. , It has been proposed that force concentration in peripapillary sclera owing to eye movements may be an IOP-independent mechanism for ONH deformation leading to glaucomatous optic neuropathy, since by this mechanism, strain is concentrated on the temporal side of the ONH where peripapillary atrophy most commonly occurs, as does the earliest damage in glaucoma.
Although peripapillary mechanical deformation is greatly exaggerated in adduction where the ON becomes tethered, eye rotation also deforms this region during smaller ductions. In papilledema, Sibony showed distortion of the peripapillary basement membrane during horizontal duction even without ON tethering. Wang and associates demonstrated by OCT in normal subjects that there are ONH strains during even moderate abduction and adduction.
Previous OCT studies of horizontal duction examined anteroposterior shifts and tilts of the ONH, but did not investigate possible horizontal and vertical deformations. Examination of the ONH by OCT is often confounded by shadows cast by epipapillary blood vessels (BVs) obscuring much of the underlining tissue. We have explored an alternative approach of en face imaging that exploits these same BVs as fiducial landmarks to study local horizontal and vertical displacements in the ONH and surrounding retina produced by gaze changes. Local deformations of the ONH and retinal surface can be deduced from differential translational shifts in local features. In the current study, we tracked large BVs on the retinal and ONH surfaces as markers of local deformation of the underlying tissues. We investigated whether horizontal ductions differently displace BVs in subjects of different ages.
Methods
Subjects
Twenty healthy young adult volunteers (11 male, 9 female) of mean ± SD age 23.9 ± 3.9 (range, 19-32) years and 20 healthy older volunteers (7 male, 13 female) of mean age 61.4 ± 9.3 (range, 48-79) years were recruited by advertising and examined to verify absence of ocular disorders besides correctable refractive error. Subjects provided written, informed consent prior to participation according to a protocol approved by the University of California, Los Angeles Institutional Review Board compliant with the Declaration of Helsinki. All subjects underwent comprehensive eye examinations to confirm normal corrected visual acuity, normal binocular alignment, and normal IOP (<21 mm Hg) without evidence of glaucomatous optic neuropathy. Only the right eye of each subject was analyzed. There were no subjects with high myopia or high hyperopia, defined as having more than 5 diopter (D) spherical equivalent refractive error. Of the total 40 subjects studied, 26 had uncorrected visual acuity at least 20/20, and so represent emmetropia or low hyperopia.
Of the younger volunteers, 12 were white and 2 each were African American, East Asian, South Asian, and Middle Eastern. The older group included 12 white, 4 African American, 1 East Asian, and 3 Middle Eastern subjects.
En Face Infrared Imaging
The infrared scanning laser ophthalmoscope mode of a Heidelberg Spectralis scanner (Heidelberg Engineering, Heidelberg, Germany) was used to image the ONH and adjacent retina. A retinal nerve fiber layer circular scan was first performed to verify normal thickness. Each right eye was then imaged in central gaze, 35 degrees abduction, and 35 degrees adduction sequentially by rotating the imager camera to calibrated angles on its azimuth pivot, including central gaze and 35 degrees abduction and adduction. The scanner rotated along with the eye, thus maintaining a constant angle relative to the line of sight. The subject’s head was stabilized to the scanner headrest using cushions and straps to prevent head rotation. To obtain images centered on the ONH, subjects fixated the scanner’s internal target that, by manufacturer’s design, is offset 12 degrees nasally from straight ahead; this offset was compensated by a custom goniometric scale to set desired ductions. During eccentric gaze imaging in some subjects, the camera was also translated toward the subject to obtain the desired gaze angle to clear the nose and other facial features. This anteroposterior camera translation changed image magnification slightly, but this was corrected during analysis. En face images were exported at 5.85 μm/pixel.
Large BVs on the retinal and ON surface were used as fiducials to track local deformations of the underlying tissues to which they are attached. Figure 1 shows a major BV branch traced during central gaze ( Figure 1 A, green) and adduction ( Figure 1 C, red). Figure 1 B superimpose the green and red paths, highlighting relative displacement between gazes. Dotted green and red paths in the figure are shown for representation of major BV displacement.

B-scan Optical Coherence Tomography
Subjects were imaged using in the OCT mode with enhanced depth imaging of the ONH and peripapillary retina (PPR). The B-scans were assessed for vitreous attachment.
Image Analysis
Images were exported as TIFF files and processed using Adobe Photoshop (Adobe Systems, San Jose, California, USA). In subjects who required translation of the OCT camera to focus the retina, magnifications of images obtained in abduction and adduction were isotropically scaled to match the size of the central gaze image. In all cases, the resulting 3 images for each eye were superimposed in a digital stack for analysis. Images obtained in abduction and adduction were rotated and translated to match the central gaze image using BVs remote from the ONH as anchor fiducials; this approach compensates for physiologic ocular torsion in eccentric gazes resulting from Listing’s law. A circular grid ( Figure 2 ) with 5 concentric rings divided radially into 8 sectors was overlaid on the image stack. The center ring had diameter equal to the ONH, and each successive ring had an integer multiple of the center diameter, dividing the retina into concentric regions. Region 0 represented the ONH. Region 1 enclosed the area 1 disc radius beyond the ONH border. Region 2 extended an additional disc radius from region 1, region 3 the next disc radius, and region 4 extended a further disc radius beyond region 3 to a maximum of 4 disc radii from the ONH. Each of the 5 regions was divided into 8 equal sectors numbered clockwise from the top, as shown in Figure 2 .

Whenever 1 or more BV intersections or branch points were present in each of the 40 zones, a marker was placed on each feature, thus demarcating fiducials that permitted robust tracking of underlying retinal displacements associated with changes in gaze direction. By alternating the opacity of images obtained in abduction and adduction superimposed on the central gaze image, displacements caused by the gaze change were tracked for each marker.
Each of the 3 image layers had associated marker fiducial layers that represented BV intersections or branch points in each sector containing such features ( Figure 3 ). ImageJ64 (W. Rasband, National Institutes of Health, Bethesda, Maryland, USA; http://rsb.info.nih.gov.easyaccess2.lib.cuhk.edu.hk/ij/ , 1997-2018, in public domain) was used to compensate magnification variations and to determine the location of each fiducial. Custom programs then calculated horizontal and vertical displacements of fiducials for each gaze position. Programs written in the MATLAB (MathWorks, Inc, Natick, Massachusetts, USA) software suite was used to plot heat maps of the displacements.

Statistical Analysis
Using GraphPad Prism (GraphPad Software, San Diego, California, USA), horizontal and vertical displacements of fiducials from central to 35 degrees abduction and adduction were compared using paired t tests in each circular region and section to determine significant differences from zero and between age groups at the 95% confidence level. All measurements were repeated by a second analyst. Results by the 2 analysts did not differ significantly.
Results
Horizontal Displacements
Adduction to 35 degrees temporally shifted vessels intrinsic to the ONH in both young and elderly subjects, indicting displacement of the ONH itself. In young subjects ( Figure 4 ), all sections (1-8) overlying the ONH (region 0) exhibited significant temporal displacement ( P < .0001) with the largest displacement in section 2 (41.7 ± 5.8 μm SEM), which is the superonasal disc. The entire nasal half (sections 1-4) of the ONH was displaced more on average than the temporal half (sections 5-8) at 4.1 ± 2.1 μm ( P < .001). The PPR (region 1) extending 1 disc radius from the ONH was significantly displaced temporally in all sections but 6 and 7, averaging 7.6 ± 1.6 μm ( Figures 4 and 5 ). There was less displacement farther than 1 disc radius from the ON center; in region 2, only section 8 was significantly displaced in adduction. There were no significant horizontal displacements in the more distal regions 3 and 4. Abduction shifted vessels in and near the ONH nasally, but less than in adduction; there were no displacements beyond the ON border (regions 1-4) in abduction.


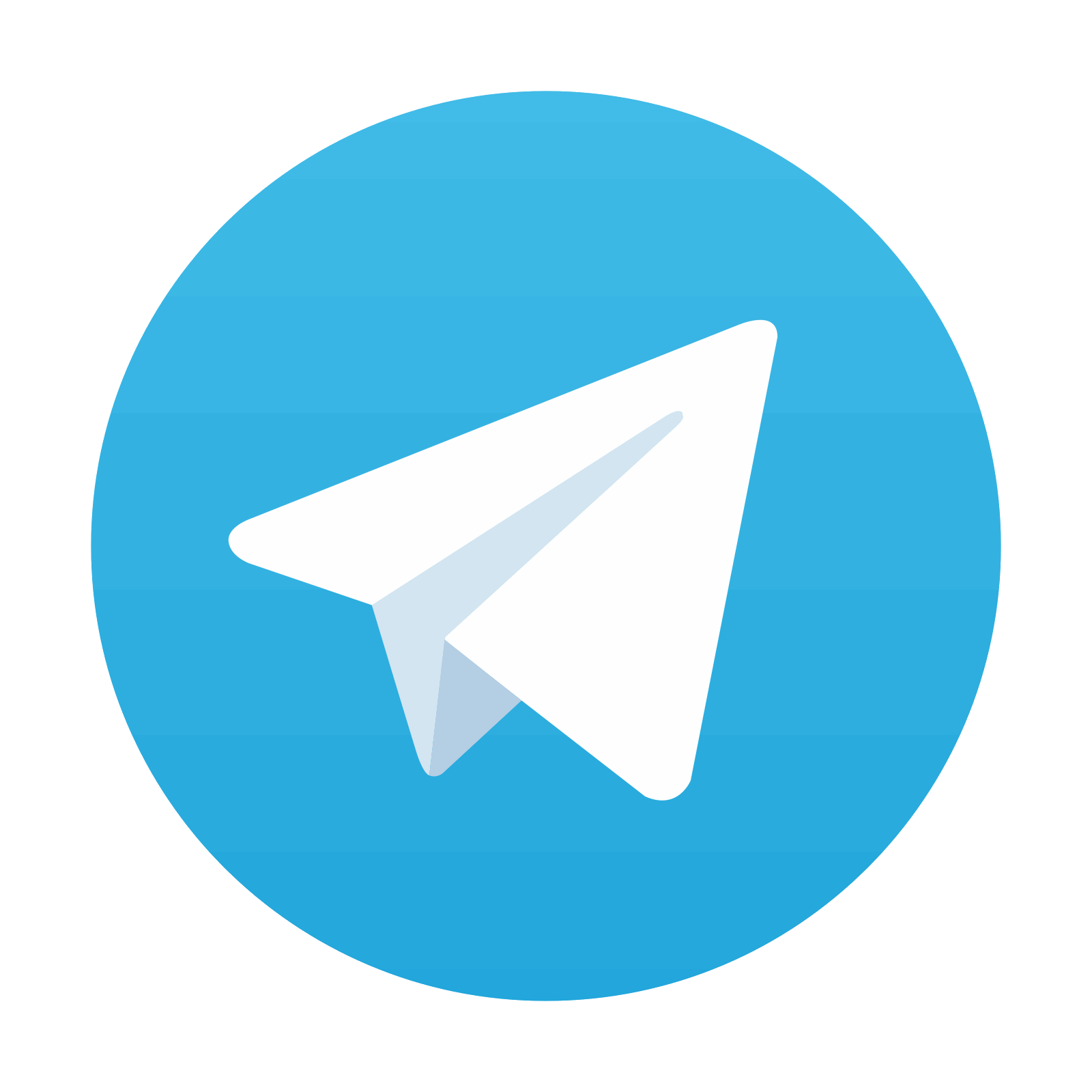
Stay updated, free articles. Join our Telegram channel

Full access? Get Clinical Tree
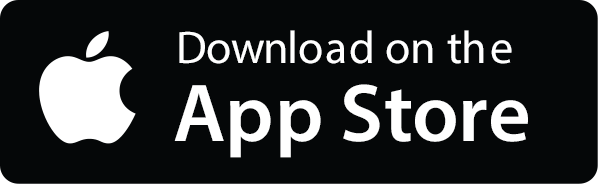
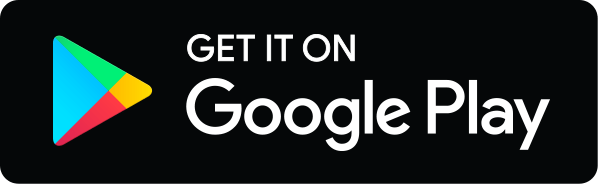
