Summary
The superior oblique is a complex muscle that primarily exerts an intorsional force to the eye, secondarily depresses the eye in adduction, and has a small abducting force, especially in downgaze. Congenital or acquired weakness of the superior oblique leads to hyperdeviation and extorsion, but the hyperdeviation has attracted most of the attention from strabismologists, who sometimes ignore the torsion. Data from superior oblique strengthening procedures indicate that correction of torsion tends to resolve the hyperdeviation irrespective of the amount of hyperdeviation. This finding, as well as computer modeling data that predict minimal vertical action of the superior oblique in primary position, points to the superior rectus as the cause of hyperdeviation in superior oblique palsy. If intorsion is impaired due to superior oblique weakness, the only muscle available to produce intorsion is the superior rectus, which corrects the intorsion with the cost of secondary hyperdeviation. Correction of intorsion therefore corrects the vertical deviation as well, without the need to recess the vertical rectus muscles.
The full tendon advancement of the superior oblique at its insertion has proven to be an effective and versatile procedure to strengthen a weak superior oblique. Other strengthening procedures include resection, tuck, plication, and the half tendon advancement (modified Harada-Ito procedure). Procedures to treat tight, overacting superior obliques include the hang-back recession, tenotomy, tenotomy with silicone expander, tenotomy with “chicken suture,” direct recession, and tenectomy. Brown’s syndrome is discussed in Chapter 10.
9 The Superior Oblique
9.1 Introduction
The superior oblique (SO) is an anatomically and functionally complex muscle with profound importance to motility. Some strabismus surgeons shy away from SO procedures, but if these surgeries are approached with diagnostic and surgical precision, the results are dramatic and rewarding.
9.1.1 Background (Why Does Superior Oblique Weakness Cause Hypertropia?)
The classic teaching by Marshall Parks and others 1 , 2 regarding correction of unilateral SO underaction was to titrate surgical dosage to the preoperative vertical deviation. The greater the deviation, the greater the number of muscles one would recess. The initial choice was usually the ipsilateral inferior oblique (IO), the next muscle would be the contralateral inferior rectus (IR), and the third would be the ipsilateral superior rectus (SR). He did, however, teach that a head tilt in a child with SO weakness could only be corrected with a tuck of the SO tendon. 1
When this author (and Alan Chow) first developed the full tendon advancement of the SO in the early 1990s, it was planned as an addition to Dr. Parks’ normal SO palsy protocol in order to reduce ipsilateral excyclotorsion. The surprise was a large overcorrection in each patient, necessitating a return of each recessed rectus muscle to its original insertion. With time, it became clear that a simple 3- to 5-mm advancement of the SO combined with recession of the IO would correct almost every hypertropia due to unilateral SO weakness, regardless of the vertical deviation amplitude. Essentially the same procedure in each case produced a total prism diopter surgical correction that highly correlated with the preoperative alignment. 3 , 4 (Orbit calculations were performed by Joel Miller, PhD, San Francisco.)
It seemed that correcting the excyclotorsion resolved the vertical deviation, so this finding was studied. Computer modeling was performed using the Orbit program, which calculated that the vertical force of the contracting SO was negligible in the primary position, and that its main action was incyclotorsion. Could it be that if the SO is underacting from any cause, the only muscle left to intort the eye is the SR? Its contraction could then cause the hyperdeviation in SO palsy, which is often quite large. Correction of the torsion would reduce the stimulus to the SR, resolving the hypertropia.
Over time, it also became clear that many vertical deviations that fit the classic diagnosis of SO palsy by alignment testing were in reality caused by other mechanisms such as contralateral IO fibrosis and pulley abnormalities (see below). As strabismologists have learned to pinpoint the diagnosis with precision, surgeries have become more accurately tailored, and results have improved.
9.2 Anatomy
The complex anatomy of the SO and its trochlea is discussed in Chapter 4. Additional anatomical points of interest to the surgeon include the ropelike nature of the tendon between the trochlea and the nasal border of the SR, and the “spread out fan” appearance of the insertion, which always has a 90-degree bend (Fig. 9‑1). The anterior torsional fibers are oriented anteroposteriorly, with the line of the insertion parallel and adjacent to the temporal border of the IR, where it is found. There is a bend in the insertion about halfway through its width, and the posterior, vertically-acting fibers are oriented horizontally, nasotemporal in orientation, under the IR. The bend is usually found about 9 to 12 mm behind the temporal border of the SR insertion, and the anterior margin of the SO insertion is found about 5 to 7 mm behind the SR temporal insertion (Fig. 9‑2).


9.3 The Weak Superior Oblique
9.3.1 Diagnosis
There is a tendency of some ophthalmologists to diagnose every vertical deviation as an SO palsy, but definitive proof would require electromyographic (EMG) recording from the muscle belly or magnetic resonance imaging (MRI) evidence of muscle atrophy. Many SO “palsies” are actually SO underactions due to lax or malpositioned tendons, 5 , 6 with a normally functioning muscle belly. Correction of the insertional/mechanical abnormality can restore fully normal SO function if the muscle itself is normal. It is less clear why SO strengthening can succeed when the muscle is weak due to paralysis, but SO advancements and tucks do work frequently in these cases. Perhaps there remains some residual SO muscle function, or the increased mechanical tension of the tendon between the trochlea and tendon insertion point intorts the eye.
Diagnosis always begins with a detailed history. In unilateral cases, was there a head tilt since infancy, or was it acquired? Did it worsen over time, or was there an acute onset, suggesting a traumatic, vascular, or inflammatory event? Sorting through old photographs is often helpful when determining head tilt history. In bilateral cases of SO weakness, there may be vertical head positioning such as a chin down position to control a downgaze V pattern.
The Parks three-step test to diagnose unilateral SO palsy 7 is applicable only to palsied or weak muscles, and not to other etiologies. It does not rule out other causes of vertical deviation, which can exhibit the same strabismus pattern, such as fibrosis of the contralateral IR (Chapter 12, Chapter 13), flap tear of the ipsilateral IR or contralateral SR (Chapter 20), or erosion of the IR by a retinal buckle (Chapter 20). Heterotopic muscle pulleys have also been shown to simulate oblique muscle dysfunction, 8 adding further complexity to the diagnosis. MRI-proven SO palsy has also been shown to be associated with medial, superior, and inferior rectus pulley displacements, adding to the strabismic deviation. 9 Accurate diagnosis therefore requires the gathering of more information beyond alignment and version testing. If alignment testing points to SO weakness, and this is corroborated by deficient depression in adduction during version testing, it also needs to be further confirmed by the observation of substantial excyclotorsion of the involved eye (or both eyes in bilateral cases) on fundus examination. If there is modest extorsion in the contralateral eye, there is more likely IR fibrosis of that eye, and SO surgery should not be considered. If there is no torsion or intorsion, something else is causing the vertical deviation, and again, SO strengthening is not indicated. Subjective measure of torsion may be helpful but is not always accurate in long-standing cases due to secondary sensory adaptations such as torsional anomalous retinal correspondence. Provided that fundus torsion still suggests SO weakness, the diagnosis is still not definitive. Pulley abnormalities must still be ruled out, as pulley heterotopy can completely mimic SO weakness, 8 , 9 including fundus torsion and abnormal torsional forced duction, and is dealt with differently (Chapter 19). In adults, pulley displacement may be ruled in or out with MRI coronal sections through the orbits, but in young children, it may await intraoperative inspection.
9.3.2 Surgical Planning
For unilateral SO “palsy”, some authors prefer recession of one or more vertical rectus muscles, with or without ipsilateral IO recession. 10 This approach avoids the risk of postoperative torsional diplopia, especially if true SO weakness is not the actual etiology. Vertical rectus recession does carry the risk of late overcorrection due to stretched scar, does not correct torsion in the extorted eye, and in the case of ipsilateral SR recession, actually worsens extorsion. One has also altered the anatomy of a normal muscle. The contralateral IR has been shown to be larger and more contractile in MRI-proven cases of SO palsy, giving some rationale for weakening that muscle. 11 The ipsilateral IO was shown to not be enlarged in SO palsy. 12 Many advocate recession or anterior transposition (AT) of the ipsilateral IO alone as the procedure of choice, 13 , 14 but this has a minor effect on extorsion. 15 If stretched scar or scar migration develops after IO AT, it will cause gradual loss of effect and a return of the original deviation, but not a late overcorrection. In several reported series, the combination of IO recession with SO tuck proved the most effective combination to treat larger primary position deviations or childhood torticollis. 16 , 17
The importance of correction of excyclotorsion in the management of SO palsy has been controversial. Traditionally, it was taught that if hyperdeviation is corrected, residual cyclodeviation is unimportant due to sensory adaptations. 8 , 18 This author’s results from over 20 years of performing SO full tendon advancements indicate that the converse also holds true: if torsion is corrected, the hyperdeviation resolves, independent of amount of vertical surgery. Vertical effects, including overcorrection with hypotropia, were reported with the Harada-Ito procedure, which was designed for torsional effect alone. 19
One hypothesis that could explain the two seemingly contradictory findings would be to consider the weak torsional actions of both vertical rectus muscles. Assuming that the eye attempts to maintain normal torsion, the SR increases its tone and the IR decreases its tone to compensate for abnormal excyclotorsion. Because the actions of these muscles are mostly vertical, however, proper torsional orientation is achieved at the expense of hyperdeviation of the eye, which is then controlled by vertical motor fusion using the contralateral eye. Correction of torsion eliminates this stimulus to the SR and eliminates hyperdeviation. Vertical rectus surgery reduces torsion in addition to reducing the effort of vertical fusion.
Computer simulation of this hypothesis using Orbit 1.8, 20 an extraocular muscle modeling program, indicates that 36 prism diopters of hyperdeviation would be created by nullification of 6 degrees of excyclotorsion using the vertical rectus muscles. An alternative and possibly complementary hypothesis is that excyclotorsion reduces the effectiveness of IR pull by creating muscle slack, and increases SR muscle tension, increasing the effectiveness of its pull. Computer simulation of this effect affirms the hypothesis, but weakly. Ten degrees of excyclorotation would create a 0.5-g enhancement of the SR and weakening of the IR. Surgical correction of excyclotorsion would also correct this factor. (Orbit calculations were performed by Joel Miller, PhD, San Francisco.)
The true action and purpose of the SO are also not fully understood. In the primary position, its function is generally thought to be pure incyclotorsion, and this has been confirmed experimentally. 21 Computer simulation 20 estimates that the SO produces 1 degree of depression in the primary position. One would, therefore, expect no more than 1.8 prism diopters of primary position hyperdeviation in complete SO palsy due to loss of the SO’s depressive effect. Why SO palsy causes primary position hyperdeviation is therefore only clear if one considers the secondary vertical effect of torsion described above. In a study involving experimentally viewed SO action in macaques, incyclotorsion was the main effect in all angles of gaze. Secondary effects normally attributed to the SO, including depression in adduction and abduction in downgaze, were attributed to secondary effects of other muscles. 22 Jampel 22 , 23 concluded that the normal function of the oblique muscles is to maintain alignment of the primary retinal meridians and to inhibit torsion. However, the SO was shown to increase its cross-sectional area during downgaze on MR images. 24
Upgaze restriction may result from IO AT, as well as large SO tucks or advancements (usually described as iatrogenic Brown’s syndrome). 16 It seems logical that more conservative surgery on both oblique muscles—one correcting the anatomical defect and the other reducing tightness of its contractured direct antagonist—would provide the most physiologic approach, with the lowest risk of restriction due to mechanical overeffect. This has been the author’s long-term experience with full tendon advancement of the SO, which is often combined with a conservative posterior (5 mm or greater from the IR insertion) recession of the IO.
9.3.3 Superior Oblique Strengthening Procedures
9.3.3.1 Techniques
See Chapter 26 for specific techniques.
9.3.3.2 Tuck
Although there is little agreement in the literature as to the preferred surgical approach to correction of SO palsy, many authors recommend SO strengthening, usually by tuck, in selected cases. 16 , 17 Being purely a shortening procedure, the tuck limits surgical options, which is a potential disadvantage when the SO weakness is due to an anomalous insertion site. 6 Also, the bulk of the tucked tissue can cause mechanical problems, and surrounding scar tissue formation may be unpredictable. Brown’s syndrome is a common complication of SO tuck. 16
The fundamental principle underlying most surgical procedures is the redirection of tissues with the creation of a scar joining two cut ends of tissue (Chapter 5). The tuck relies on permanent redirection of tissues primarily by suture alone, without predictable redirection of forces by scar tissue, as there are no cut ends of tendon to initiate healing. The long-term effect of the tuck therefore is dependent on the strength and stability of the suture alone.
9.3.3.3 Full Tendon Advancement of the Superior Oblique
A procedure that creates a simple directed scar would theoretically improve stability. Additionally, a procedure that limits dissection to a few millimeters of tendon insertion could reduce postoperative adhesion formation.
Disinsertion and advancement of the SO tendon provides, at least theoretically, unlimited surgical options for repositioning the tendon and changing the actions of the muscle (Fig. 9‑3, Fig. 9‑4, Fig. 9‑5). Also, it is more likely that a localized, directed scar would allow more natural tendon function. These considerations led us to design a procedure to strengthen the SO with dissection limited to the insertion site alone.



Overcorrections were common in early cases, in which the SO was advanced to the superior border of the lateral rectus (LR) muscle (similar to the Harada-Ito procedure). By reducing the advancements to 4 to 5 mm in most cases, postoperative incyclotorsion and Brown’s syndrome were avoided. The resolution of primary position hyperdeviation with SO advancement (sometimes with conservative IO recession) was a surprise. In other words, essentially the same procedure corrected from 0 to 45 prism diopters of primary position hypertropia.
The results were analyzed in 59 patients. Interestingly, it became evident that the amount of correction of vertical deviation was relatively independent of the amount of surgery, and that if torsion was corrected, the vertical deviation resolved. Essentially, the same procedure (with small variations in amount of SO advancement and anteropositioning of the IO) corrected from 6 to 45 prism diopters of vertical strabismus. The correlation comparing total correction to preoperative deviation in this group was 0.96, which is highly significant (P < 0.0001; Pearson Product Moment Correlation). 3 , 4
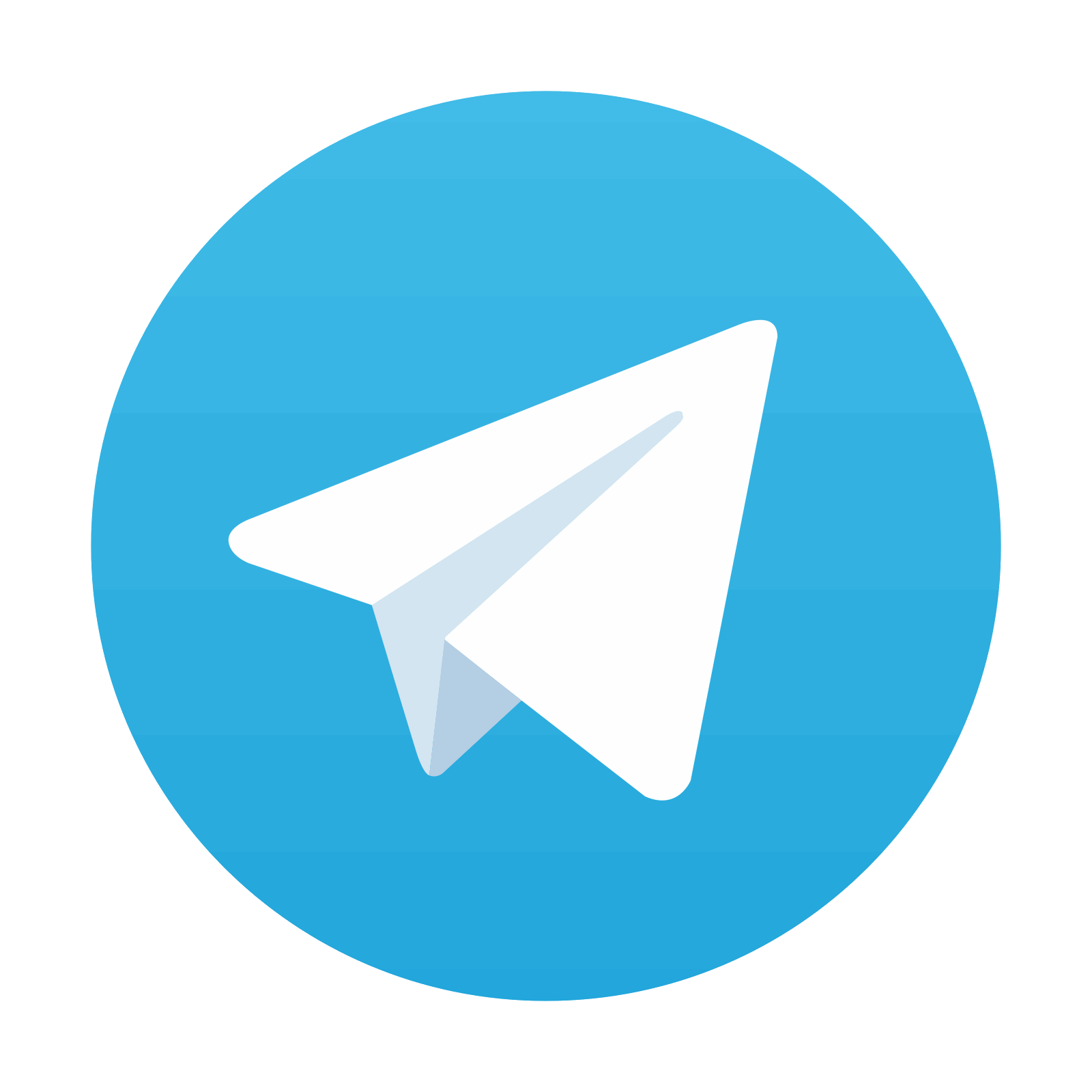
Stay updated, free articles. Join our Telegram channel

Full access? Get Clinical Tree
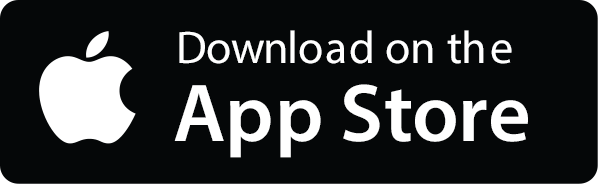
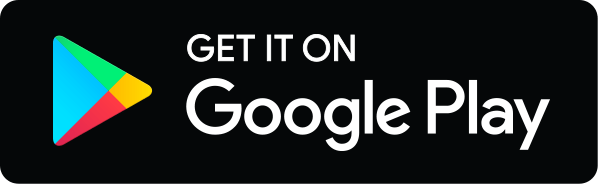
