Summary
We have come far in the surgical correction of strabismus, but there is much more on the horizon. One advance already expected to be available in the near future is automated measurement of alignment and fusion. Automated prism adaptation testing will not be far behind. Greater availability of high-resolution magnetic resonance imaging (MRI) combined with the automated alignment data would allow computerized calculation of a customized surgical plan for each patient. Inclusion of collagen and wound healing data for that patient would add further predictability and stability to the surgical result. New materials and devices to repair and replace traumatized tissues and restore motility in scarred, restricted strabismus may also be developed in the future. Targeted manipulation of the wound healing process could potentially reduce the complications of excessive fibrosis and hyperkeratosis as well as stretched scar, scar migration, and other results of weak healing. Anatomical studies of the orbital connective tissue pulleys and intermuscular bands have recently begun to lead to the development of new strabismus surgical procedures, but more is bound to come as strabismologists try different approaches.
36 Imagining the Future of Strabismus Surgery
36.1 Introduction
The field of strabismus is entering an exciting new phase. The paradigm shift in understanding eye muscle anatomy and function with the critical importance of the supporting orbital walls and connective tissue pulleys is just starting to give rise to new approaches to strabismus repair. These new concepts have been sprinkled throughout the book. What possibilities can we envision for the future?
36.2 Automated Diagnosis and Surgical Planning
I asked Robert Clark (Chapter 4) to share his vision for the future of strabismus surgery. His concepts were unique and different from my own, so they are presented in the box below in his own words.
My goal is not to create a computerized system—I think that will happen without my help. My goal is to determine what are the important measurements that an automated system should capture to improve the precision of both diagnosis and treatment. I can visualize a day when a patient will enter your office and be given a set of smart goggles to wear. By the time you walk in, the smart goggles will have uploaded all of the motility data into your tablet and the AI [artificial intelligence] has already provided the diagnosis. Imaging will have been performed to determine the rotational axis and EOM [extraocular muscle] positions, using office-based technology like OCT [optical coherence tomography] and ultrasound. During your brief time with the patient, you will quickly verify the findings—no cover testing, just the primary gaze misalignment and any limitations in ductions. You plug in the misalignment and, based on the exact leverage for the different EOMs in that patient, you customize which muscles to work on and how much surgery to perform. You hit “send” and the patient receives a copy of the clinical findings and your recommendations, and the family makes a decision about surgery. Your total time with the patient—less than 5 minutes.
The other future possibilities are ones that we have discussed already—the movement towards direct pulley surgery instead of EOM insertional surgery and partial or compartmental muscle surgery based on the exact compartment that gives the best effect.
36.2.1 EyeTracking–Based Strabismus Diagnosis
The strabismus-diagnosing smart goggles may already be here. An innovative Israeli company, Novasight, demonstrated just such a system, called Eyeswift, at the 2018 combined meeting of the International Strabismological Association and the American Association for Pediatric Ophthalmology and Strabismus. 1 Their own summary of their system is presented in the following box.
Measuring eye deviation can sometimes be challenging in babies, toddlers and children. The current gold standard cover test requires the examiner to observe the ocular movements while covering and uncovering the eyes. Yet, this test suffers from large variably [sic] both due to intra- and inter-examiner variability and intra-patient variability. 1 , 2 , 3 , 4 In addition, the angle of strabismus may vary over time. 5 Accurate, subjective and automated measurements that are easily repeated several times may improve the measurement accuracy and thus significantly decrease the variability of test results.
A newly developed eye-tracking-based system is aimed for objectively measuring both manifest and latent eye deviation. An eye tracker estimates the point of gaze in real time from a video image of the pupil with high accuracy, using advanced image processing and mathematical algorithms. Near-infrared illumination is used to create reflection patterns on the cornea (glint) and pupil of the eye, while these reflections are detected by an infrared camera. As a result, an eye tracker can detect eye movements similarly to an eye care specialist, but with a much greater precision.
The new system comprises dedicated synchronized liquid crystal glasses (LCD), used for ocular occlusion to enable alternating opening and occluding of each eye (i.e., monocular or binocular viewing), similarly to the traditional cover test. In contrast with the traditional cover test, the position of both the occluded and the non-occluded eyes is continuously registered and then combined to calculate the exact deviation between the eyes. The subject puts on the testing LCD glasses and is asked to watch a short video. No verbal skills are required to perform the test and no initial calibration dependent on a high level of patient collaboration, rendering it suitable for infants as young as 1 year. Using a fast, objective and accurate algorithm, the system detects and measures the magnitude and direction of both manifest and latent components of eye deviation and other properties of binocular function, such as fusional reserves (convergence and divergence), thereby enabling orthoptic assessment in locations deprived of other services and substantially increasing the efficiency in clinics. The newly developed instrument was successfully clinically tested on adults and children. 6
36.2.2 Automated Prism Adaptation
An automated smart goggle system, as described above, could potentially be adapted to perform in-office prism adaptation (Chapter 2), which is a very useful but time-consuming test. With current insurance reimbursement for office visits, there is little incentive for strabismologists to devote the extra time required for prism adaptation. Automating the process would be a game-changing advance.
36.3 Improved Imaging, Upright MRI, Upright Surgery
The high-quality magnetic resonance imaging (MRI) described in Chapter 4 is not yet widely available. Hopefully this technology will be adopted by more centers and improve the precision of strabismus diagnosis.
Pulley shifts with greater extraocular muscle (EOM) sideslip and atypical globe translation during shifts of gaze position have been demonstrated in Marfan’s syndrome. 7 It would make sense that gravity could cause pulley shifts and play a role in strabismus in patients with weak collagen, but this has not been studied. If MRI could be performed in an upright posture, this could reveal such a mechanism, if present, and allow precise correction.
Modification of surgical beds and anesthesia delivery methods to allow strabismus surgery with the patient in an upright position would also be an advance that would allow us to better identify and correct gravity-related pulley shifts.
36.3.1 Extraocular Muscle Scan Analysis
Joel M. Miller, PhD, predicted the existence of EOM pulleys with biomechanical modeling and confirmed their function in determining muscle paths using novel MRI analyses, before and after muscle transposition surgery. 8 , 9 , 10 Since that time, orbital MRI has been used to many ends, and refinement of his original technique is necessary to improve accuracy and reduce bias. Although currently suitable only for research, these methods can likely be automated for use in the clinic. The rest of this section is Dr. Miller’s summary of his work.
Many studies have been performed using the MRI image analysis techniques we introduced 8 to estimate extraocular muscle (EOM) paths, shapes, and sizes, demonstrating, for instance, EOM pulleys 9 and the hypertrophy resulting from bupivacaine injection. 3 Unfortunately, some studies have also used the presumptive precision and objectivity of MRI to draw dubious conclusions. Although no methodology can ensure good science, almost three decades of experience suggest refinements that would improve the reliability of quantitative extraocular MRI. Four aspects of scan analysis must be refined to address errors and biases that can affect MRI quantification. 11
36.3.1.1 Scan Planes Perpendicular to the Muscle’s Long Axis
Analyzing muscles in tomographic images begins by segmenting the structures of interest by delineating muscle outlines and perhaps outlines of other structures. High spatial resolution and high contrast between the object and its surround are essential for accurate segmentation. Tightly framing a region of interest (such as one or both eyes) in the scanner’s field of view maximizes spatial resolution. High contrast is achieved when the EOMs are viewed against contrasting orbital fat (as with quasi-coronal sections of rectus muscles), rather than against the poorly contrasting globe or optic nerve (which occurs with sagittal sections of the horizontal rectus muscles).
MRI data is not isotropic. The best spatial resolution, perhaps 1/3 × 1/3 mm, is obtained in scan planes, which are typically separated by about 2 mm. Although a suitable stack of scan planes can be reformatted for viewing at any angle, resolution is lost. Each 1/3 × 1/3 mm pixel in a scan-plane image represents the average MRI signal from a 2 mm thick block of tissue, and if structures of interest change position in the scan plane as they traverse that distance, the result will be a blurry average across affected voxels. Thin slices suffer less from such volume averaging, but provide weaker signals with more noise. If elongated structures, such as EOMs, are imaged in planes perpendicular to their long axes, there is little structural variation through the thickness of a voxel, and volume averaging contributes only to signal strength. Even where it is possible to image longitudinal views of a muscle against orbital fat, volume averaging blurs contours and distorts muscle outlines. Quasi cross-sectional or quasi-coronal scan planes should therefore always be used for imaging EOMs. 12 Unfortunately, extraocular muscle studies using transverse or sagittal sections are still performed under the mistaken assumption that they provide more direct, complete pictures. 13 Actually, because of volume averaging, apparent muscle sizes, shapes, and paths are so strongly dependent on thickness and position of slice planes that such images can be unreliable.
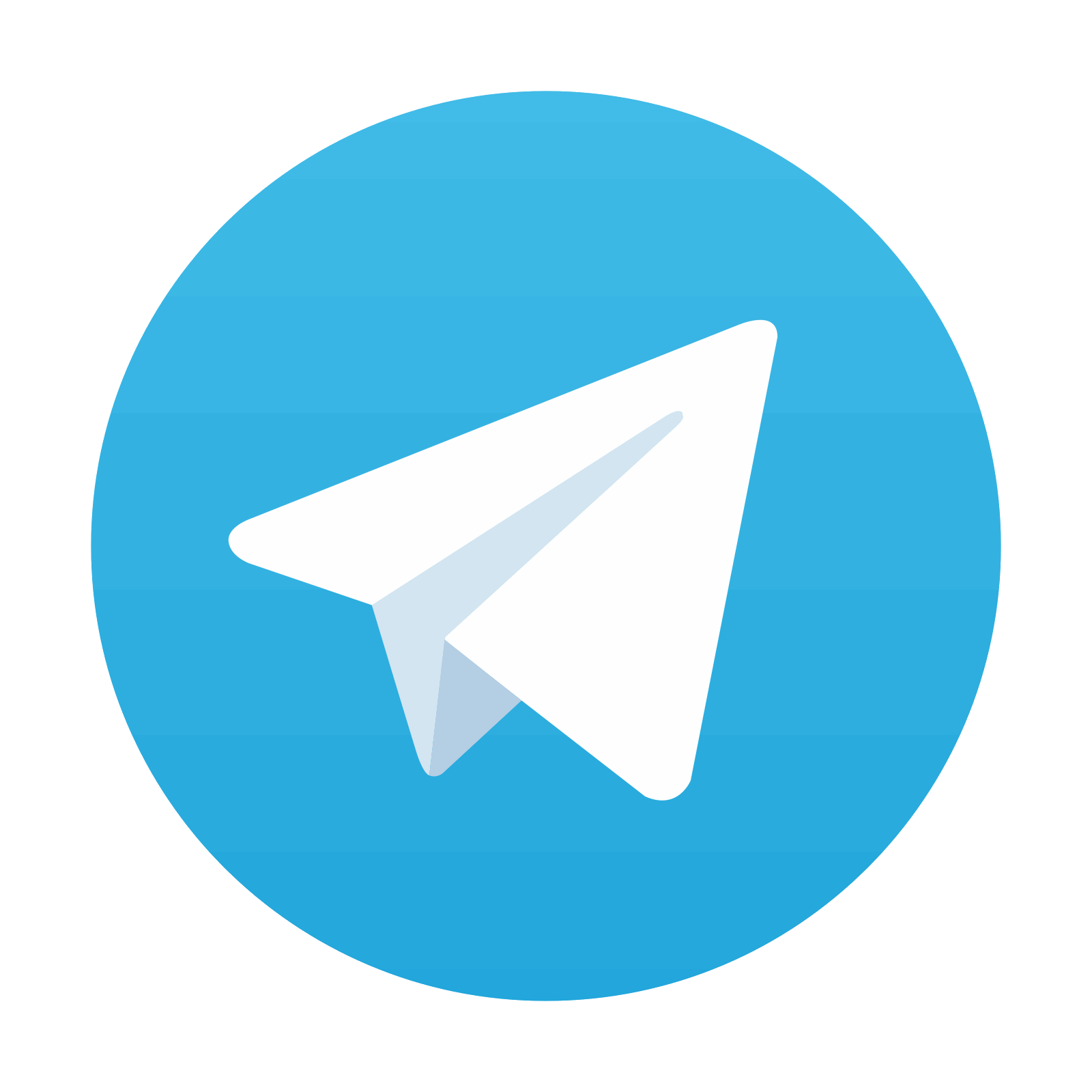
Stay updated, free articles. Join our Telegram channel

Full access? Get Clinical Tree
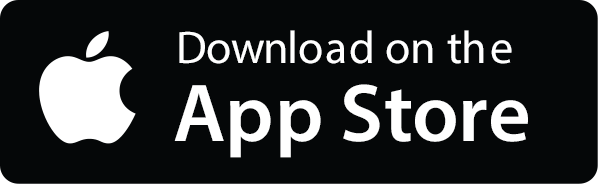
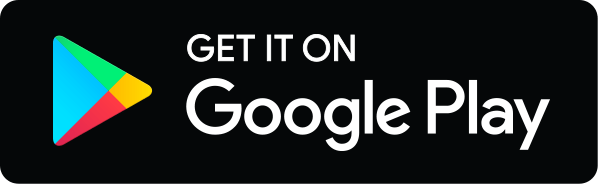
