34 Drug and Light Ocular Toxicity
Retinal toxicity may occur secondary to a variety of systemic medications. Fortunately, in many cases, the loss of visual function is mild, or is reversible with discontinuation of the inciting drug. Nevertheless, in some instances, permanent or progressive visual loss may occur. We present only those medications known to produce a well-described fundus abnormality. Others, which have not definitely been proven to cause retinal toxicity, are not included in this discussion.
Changes in fundus appearance and retinal function can also follow exposure to light waves. This can occur with visible (solar retinopathy, operating microscope toxicity, laser) as well as invisible (radiation retinopathy, welding arc retinopathy) light and radiation. These entities are presented along with suggestions for prevention.
34.1 Systemic and Topical Medication Toxicity
34.1.1 Disruption of the Retina and Retinal Pigment Epithelium
Phenothiazines
Thioridazine
Acute toxicity with thioridazine (Mellaril) is characterized by symptoms of blurred vision, dyschromatopsia (reddish or brownish discoloration of vision), and nyctalopia. In the earliest stages, the fundus appearance may be normal or display only mild granular pigment stippling posterior to the equator. An intermediate stage can then develop consisting of circumscribed nummular areas of retinal pigment epithelial (RPE) loss from the posterior pole to the midperiphery (Fig. 34-1a). 1 Fluorescein angiography reveals disruption of the choriocapillaris in these zones (Fig. 34-1b). In the late stages, more wide-spread areas of depigmentation alternating with hyperpigmented plaques, vascular attenuation, and optic atrophy are observed (Fig. 34-2).


Retinal toxicity from thioridazine depends more on the total daily dose than on the cumulative amount received. 2 With higher daily doses, toxicity can develop rapidly, even within the first 2 weeks of therapy. Toxicity is rare at dosages of less than 800 mg/d, although a few cases have been reported with low doses given over several years.
In the initial stages, visual field testing may reveal mild constriction and paracentral or ring scotomas. The electroretinogram (ERG) is either normal or shows decreased oscillatory potentials. In the later stages, both the rod and cone functions of the ERG as well as the electrooculogram (EOG) are markedly abnormal. If the drug is stopped early, ERG testing improves within the first year. 3 Histologic studies demonstrate that atrophy and disorganization of photoreceptor outer segments occur before the loss of the RPE and choriocapillaris.
The early fundus changes associated with thioridazine often progress despite discontinuation of therapy following recognition of acute toxicity. 1 It is unclear whether this degeneration represents continued toxicity of the drug, or rather a nonspecific, delayed expansion of chorioretinal scarring to areas of subclinical, preexisting RPE damage. 3 Visual function, in contrast to fundus appearance, usually improves within the first year following a toxic reaction; this likely would not occur if thioridazine caused persistent toxicity. Nevertheless, in a few cases, there does appear to be a late, slow decline in functional ability as well as anatomic appearance. The precise mechanism of thioridazine-mediated toxicity is unknown, but mechanisms have been postulated involving dopamine receptor blockade or pathways leading to oxidative stress. 4 , 5 Many phenothiazines are known to bind to melanin granules of the RPE and uveal tissue, but not all commonly cause retinal toxicity. A compound, NP-207 (piperidyl chlorophenothiazine hydrochloride), has a very similar chemical composition to that of thioridazine, including the same piperidyl side chain. This drug was never marketed because of the pronounced pigmentary retinopathy that developed during clinical trials. This may account for the more retinotoxic effect of thioridazine in comparison with other phenothiazines, such as chlorpromazine, which do not have a piperidyl side chain. Experimental studies have shown an alteration of enzyme kinetics during phenothiazine administration and toxic inhibition of oxidative phosphorylation with subsequent abnormalities in rhodopsin synthesis. Further study is needed to determine whether these observed effects are involved in the pathogenesis of thioridazine toxicity.
The evaluation of patients on thioridazine should include a review of the daily and cumulative dose. Baseline fundus photography and possibly ERG testing may be helpful if future toxicity develops. Given the many antipsychotic medications available today, consideration of alternative agents may be discussed with the patient’s psychiatrist. At the earliest sign of toxicity, thioridazine should be discontinued.
Chlorpromazine
Chlorpromazine (Thorazine) is a piperazine similar to thioridazine, but it lacks the piperidyl side chain, as mentioned earlier. The compound binds strongly to melanin and can cause pigmentation in the skin, conjunctiva, cornea, lens, and retina. 6 Other ocular effects include oculogyric crisis, miosis, and blurred vision secondary to paralysis of accommodation. Usual doses range from 40 to 75 mg/d, but doses up to 800 mg/d are not uncommon.
Retinal toxicity from chlorpromazine is rare. When massive doses are given, such as 2,400 mg/d for 12 months, they may cause pigmentary changes in the retina with attenuation of retinal vessels and optic nerve pallor. As with thioridazine, the development and extent of toxicity are more closely related to the daily dose than total amount of drug taken.
Chloroquine Derivatives
Chloroquine
Chloroquine (Aralen) was first used as an antimalarial drug in World War II. Currently, it is used for treatment of amebiasis, rheumatoid arthritis, and systemic lupus erythematosus. Retinal toxicity with degeneration of the RPE and neurosensory retina secondary to long-term daily use of chloroquine has been well described. 7 Most of the cases of retinopathy, however, developed when a higher than currently recommended dosage of 250 mg/d or 4 mg/kg per day was used. A daily dose exceeding 250 mg with a total dose of between 100 and 300 g is usually needed to produce toxicity. 8 One study showed a 19% incidence of chloroquine retinopathy (mean daily dose of 329 mg), which was increased in those older than 40 years and those with higher cumulative doses. 9 Conversely, with strict adherence to a low daily dose, the incidence of retinal abnormalities is minimal, even when total doses reach more than 1,000 g.
The earliest clinical sign of retinal toxicity may be a paracentral scotoma, which can precede the development of any ophthalmoscopic or ERG abnormality. 10 A high percentage of patients with chloroquine retinopathy also demonstrate color vision defects, predominantly blue-yellow deficiencies. 11 Subtle macular pigment stippling with a loss of the foveal light reflex usually appears on fundus examination before the development of a classic bull’s-eye maculopathy, in which a ring of depigmentation surrounded by an area of hyperpigmentation is seen centered on the fovea (Fig. 34-3). When the RPE abnormalities involve the center of the fovea, the visual acuity is affected. The peripheral retina can display pigment mottling, which may, in severe cases, develop the appearance of a primary tapetoretinal degeneration, with narrowed retinal vessels, optic disc pallor, and eventual blindness.

After the cessation of chloroquine treatment, early, subtle macular changes can revert to normal and advanced cases may progress (Fig. 34-4a, b), but the large majority remain stable even with long-term follow-up. 12 Chloroquine is very slowly excreted from the body. It has been found in the plasma, red blood cells, and urine of patients up to 5 years after the last known ingestion. It is not surprising, therefore, that delayed onset of chloroquine retinopathy can be seen at an interval of up to 7 years or longer after discontinuation.

Fluorescein angiography can be helpful in the early demonstration of pigment abnormalities in the macula. There is minimal evidence of damage to the choriocapillaris on the fluorescein angiogram in the areas of pigment disturbance. The ERG and EOG may be abnormal early, although the EOG is sometimes supernormal initially. Multifocal ERG is capable of demonstrating evidence of toxicity before abnormalities develop on full-field ERG. 13 Histopathologic sections demonstrate loss of RPE pigmentation, with an accumulation of pigment-laden cells in the outer retinal layers and damage or reduction of photoreceptors. Electron microscopic studies reveal more widespread damage to the retina, with most of it occurring in the ganglion cell layer.
The mechanism of chloroquine-mediated retinal toxicity has not been fully elucidated. Like the phenothiazines, chloroquine is bound by melanin and concentrated in the RPE and uveal tissues. In vivo and in vitro rat models have revealed that chloroquine disrupts lysosomal function in retinal neurons and RPE. 14
The American Academy of Ophthalmology (AAO) has issued toxicity screening recommendations for chloroquine and hydroxychloroquine (see “Hydroxychloroquine” section). If any abnormalities are detected, chloroquine therapy should be stopped.
Hydroxychloroquine
Hydroxychloroquine (Plaquenil) is also used for the treatment of rheumatoid arthritis and systemic lupus erythematosus. This medication can produce a retinopathy identical to that associated with chloroquine. With a daily dose of less than 6.5 mg/kg/d and lack of systemic renal disease, hydroxychloroquine is typically well tolerated. In many cases, cessation of therapy can result in stabilization of retinopathy; thus, regular surveillance is critical to detect early toxicity. The overall prevalence of hydroxychloroquine retinopathy is approximately 7.5%. Risk factors for the development of toxicity include daily dose per real body weight, daily dose per ideal body weight, duration of use, kidney disease, and concurrent tamoxifen treatment. 15
Adjunctive testing now allows for earlier detection of hydroxychloroquine toxicity. Fundus autofluorescence (FAF) has become widely used imaging tool with a fairly high sensitivity for recognition of early toxicity. Mild retinopathy may show a pericentral ring of increased FAF, while more advanced stages may present as pericentral mottled loss of FAF with increased FAF in the adjacent retina. Late stages may demonstrate a complete loss of pericentral FAF. 16 Many regard multifocal ERG as the most definitive test of toxicity. An analysis of multifocal ERG findings in long-term hydroxychloroquine users revealed multiple patterns of abnormalities—paracentral loss, foveal loss, peripheral loss, and generalized loss (Fig. 34-5). 17 The optical coherence tomographic (OCT) abnormalities associated with hydroxychloroquine toxicity include discontinuity of the inner segment/outer segment junction, thinning of the outer nuclear layer, and hyperscattering at the outer segment level (Fig. 34-6). 18 However, perifoveal thinning of the inner plexiform and ganglion cell layers on OCT may precede structural changes to the photoreceptors. 19


In 2011, the AAO recommended baseline examination within 1 year of starting the medication, including a comprehensive dilated examination, 10–2 white Humphrey Visual Field testing, and at least one of the following objective examinations: spectral domain OCT, multifocal ERG, or FAF. Amsler grid may have some utility in monitoring for a paracentral scotoma, but should not replace formal perimetry. Annual screening should begin at 5 years with the same testing regimen, or sooner in high-risk patients. 20
In 2016, the American Academy of Ophthalmology task force on screening recommendations for hydroxychloroquine retinopathy published updated screening guidelines. The newest recommendations lowered the recommended safe dosage to <5.0 mg/kg per day of real body weight. Humphrey 10-2 visual field and SD-OCT were recommended annual screening methods, starting at 5 years after initiation of therapy. Ophthalmologists should be aware of perifoveal retinopathy sometimes seen in patients of Asian descent, as well as an increased risk of retinopathy with coexisting renal disease and concurrent tamoxifen usage.
Quinine
Quinine was first used for the treatment of malaria in World War II, but currently it is prescribed for the management of nocturnal muscle cramps. The recommended daily dose is less than 2 g. Signs of systemic toxicity occur with doses greater than 4 g, and the fatal oral dose is 8 g. Ocular toxicity with quinine develops after an overdose, either by accidental ingestion or by attempted abortion or suicide. A syndrome known as cinchonism is rapidly produced and consists of nausea, vomiting, headache, tremor, and sometimes hypotension and loss of consciousness. When patients awake, they are often completely blind with dilated, unreactive pupils. 21 In the acute stages, fundus examination reveals mild venous dilation with minimal retinal edema and normal arterial caliber. The fluorescein angiogram displays minimal abnormalities. ERG testing shows an acute slowing down of the a-wave with increased depth, loss of oscillatory potentials, and a decreased b-wave. EOG and visual-evoked potential (VEP) testing are also abnormal.
During the next few days, visual acuity returns, but the patient is left with a small central island of vision. A progressive attenuation of the retinal arterioles with the development of optic disc pallor occurs during the next few weeks to months (Fig. 34-7). The increased depth and slowing down of the a-wave revert to normal. The b-wave first normalizes, and then slowly decreases. The VEP remains abnormal, and dark adaptation reveals little or no rod function. 21 OCT demonstrates inner retinal atrophy. 22

Early investigators believed the mechanism of quinine toxicity to be vascular in origin. This was based primarily on the fundus appearance several weeks after ingestion, which displays marked arteriolar attenuation and optic disc pallor. Recent experimental and clinical studies have demonstrated minimal involvement of the retinal vasculature in the early stages of quinine toxicity. 21 Furthermore, ERG and histologic studies show that the site of toxicity is likely the retinal ganglion, bipolar, and photoreceptor cells. The exact mechanism of quinine toxicity is unidentified, but some have suggested that it may act as an acetylcholine antagonist and disrupt cholinergic transmission in the retina. 23
Clofazimine
Clofazimine is a red phenazine dye that has been used to treat dapsone-resistant leprosy, psoriasis, pyoderma gangrenosum, discoid lupus, and recently Mycobacterium avium-intracellulare complex infections in patients with AIDS. With treatment lasting several months, clofazimine crystals may accumulate in the cornea. Cases of bull’s eye maculopathy with pigmentary retinopathy have been reported in patients with AIDS with doses of 200 to 300 mg/d (total dose of 40–48 g). Visual acuity was mildly affected, with reduced scotopic, photopic, and flicker ERG amplitudes. Cessation of treatment may result in the clearance of corneal deposits but does not appear to affect the retinopathy.
Dideoxyinosine
A midperipheral pigmentary retinopathy (Fig. 34-8) has been noted in children with AIDS receiving high-dose therapy with the antiviral 2′,3′-dideoxyinosine. The cases were associated with ERG and EOG changes. Similar phenotypes have been observed in adults, with chorioretinal atrophy anterior to the vascular arcades with corresponding hypoautofluorescence or mottled hyperautofluorescence and hypoautofluorescence. OCT confirms severe chorioretinal atrophy in these regions. 24 The retinal toxicity typically stabilizes after discontinuation of the medication.

Deferoxamine
Intravenous and subcutaneous administration of deferoxamine (Desferal) has been used to treat patients who require repeated blood transfusions and in whom complications of iron overload subsequently develop. High-dose intravenous and subcutaneous therapy has produced visual loss, nyctalopia, peripheral and central field loss, reduced dark adaptation, ERG, and EOG responses. 25 Fundi can be normal initially, or there may be a faint graying of the macula.
Pigmentary changes in the macula and periphery develop within a few weeks. In some instances, toxicity may present as vitelliform macular lesions. 26 Accumulated material has been shown to deposit within the outer retina or in the Bruch’s membrane–RPE complex on OCT. 27 Visual function returns with cessation of therapy. Deferoxamine forms chelates with many metals other than iron, and it is possible that the mechanism of toxicity may involve the removal of copper from the RPE. 25 Histopathologic changes occur primarily in the RPE and include loss of microvilli from the apical surface, patchy depigmentation, vacuolation of the cytoplasm, swelling and calcification of mitochondria, and disorganization of the plasma membrane.
Cisplatin and Carmustine
Cisplatin (Platinol) and carmustine (BCNU) are used for the treatment of malignant gliomas and metastatic breast cancer. Retinal toxicity of three different types has been reported with these agents. A pigmentary retinopathy of the macula with markedly decreased visual acuity and abnormal ERG and EOG testing has been reported after administration of intra-arterial cisplatin and carmustine, and with cisplatin alone for malignant glioma. 28 In another study, intra-arterial cisplatin with intravenous carmustine caused macular pigmentary and decreased vision, but with a normal ERG. 29 These findings may be the result of platinum toxicity to the retina, as is seen with other metals, such as iron and copper. A retinopathy consisting of cotton-wool spots, intraretinal hemorrhages, and macular exudate and optic neuropathy with disc swelling have been reported after high-dose chemotherapy with cisplatin, cyclophosphamide, and carmustine and autologous bone marrow transplantation for metastatic breast cancer. 30 These changes were thought to be associated with a 1.2-fold higher cisplatin dose given to affected patients.
In approximately 65% of patients receiving intra-arterial carmustine alone or combined with cisplatin for malignant glioma, a vascular retinopathy or optic neuropathy develops that can include arterial occlusion, vasculitis, and papillitis. 28 These fundus changes are associated with profound visual loss that begins about 6 weeks after the start of therapy. Other ocular effects may include orbital pain, chemosis, secondary glaucoma, internal ophthalmoplegia, and cavernous sinus syndrome. Injection of the medication above the ophthalmic artery can still result in toxicity. Visual loss is usually progressive, and no treatment is known.
34.1.2 Vascular Damage
Cisplatin and Carmustine (BCNU)
Oral Contraceptives
Oral contraceptives have been implicated in some cases of central retinal vein occlusion, retinal and cilioretinal artery obstruction, and retinal edema. The synthetic estrogen and progesterone contained in contraceptive pills are thought to affect coagulation factors adversely and induce a hypercoagulable state leading to thromboembolic complications. Most of the studies reporting ocular complications are from the 1960s and 1970s, when the estrogen concentrations used in the contraceptive pills were much higher. Recent prospective studies have not shown an increased incidence of ocular pathology in patients taking oral contraceptives. 31
Interferon
Interferons α2a and α2b were originally used as an antiviral agent, but it is currently used for Kaposi’s sarcoma, hemangiomas of infancy, and chronic hepatitis C, in chemotherapy protocols, and experimentally for the treatment of choroidal neovascular membranes. Interferon therapy has been associated with the development of multiple cotton-wool spots and retinal hemorrhages (Fig. 34-9). 32 Visual acuity is usually not affected. Changes are noted within the first 4 to 8 weeks of therapy and are seen more frequently in diabetic and hypertensive patients.

Miscellaneous Agents
Ergot alkaloids in higher than recommended doses have been reported to cause retinal vasoconstriction, and over-the-counter phenylpropanolamine used in appetite suppressants and decongestants has been implicated in one case of central retinal vein occlusion.
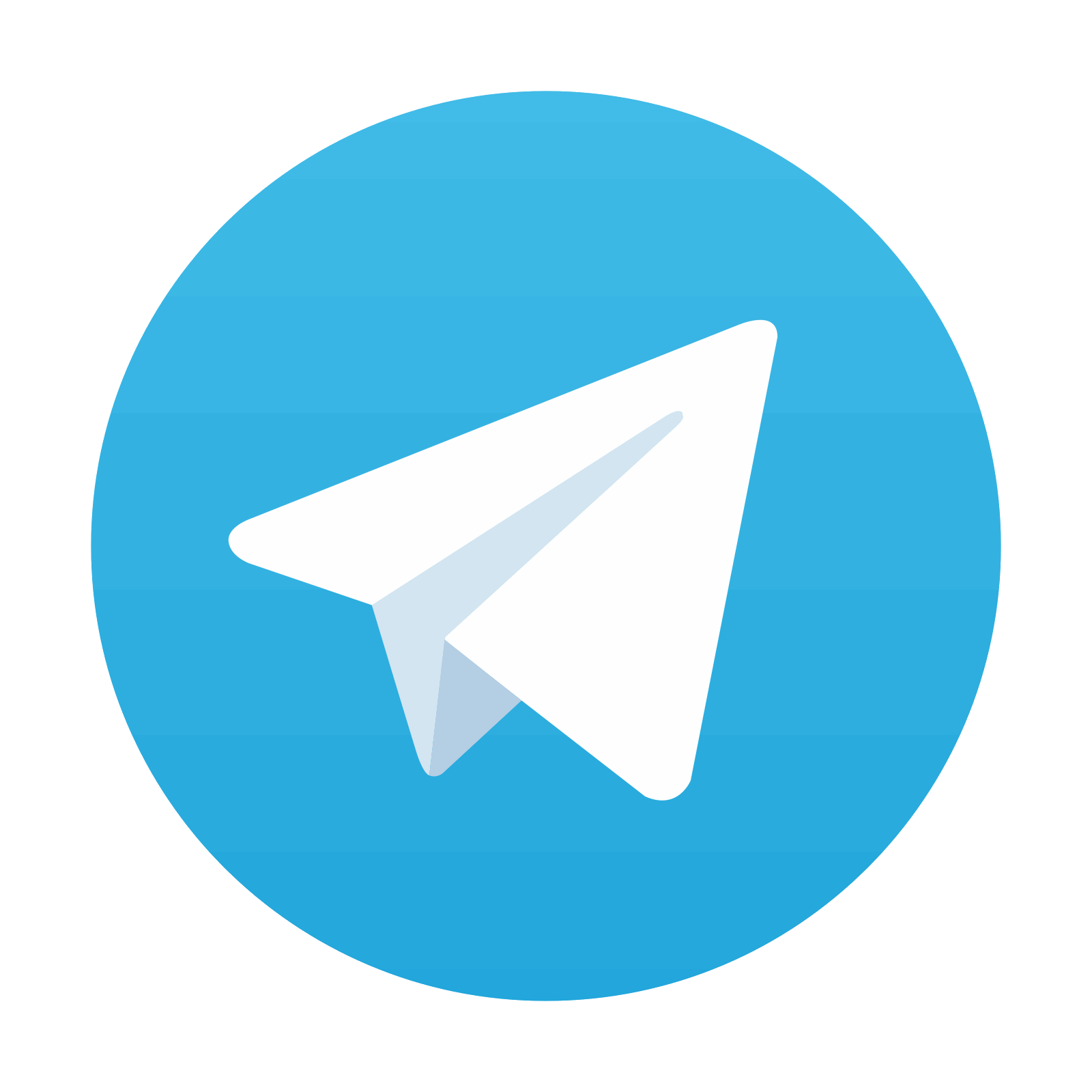
Stay updated, free articles. Join our Telegram channel

Full access? Get Clinical Tree
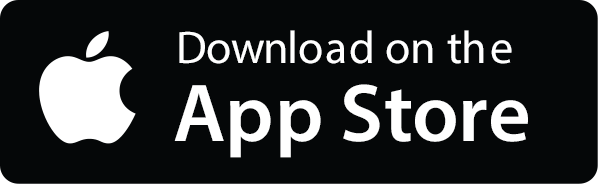
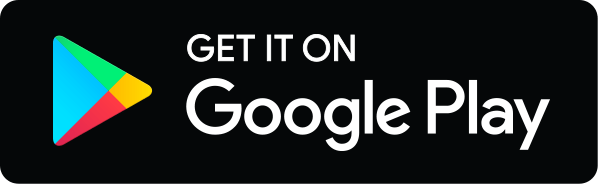
