Summary
Congenital encephaloceles are a rare type of neural tube defect caused by a herniation of the cranial content through areas of arrested bone development in the skull. Nasal encephaloceles in particular are herniations localized to the anterior skull which include frontoethmoidal and basal subtypes. Although there have been no definitive factors implicated in the etiology of these defects, the prevailing theory on their pathogenesis centers around failure of the neuroectoderm to separate from the ectoderm surface during the fourth week post-conception, leading to an area of weakness through which brain and meninges can herniate. The majority of nasal encephaloceles is sporadic, although a small percentage occurs associated with other craniofacial malformations. Given their potential life-threatening complications, which include meningitis and infection of the central nervous system, an early diagnosis is mandatory. Treatment is primarily the surgical resection leading to favorable outcomes in most cases. The endoscopic transnasal approach offers an effective and safe surgical option in selected cases, without major consequences on craniofacial growth.
33 Surgery of Skull Base Meningoencephalocele
33.1 Definition and Historical Notes
Encephaloceles (ECs) are defined as protrusions of the cranial contents beyond the normal confines of the skull through a defect in the cranium and the facial bones.
They account for about 10% to 20% of all craniospinal dysraphisms. The incidence is approximately 1/3,000 to 1/10,000 live births, but the true incidence is likely considerably greater because about 70% of ECs result in loss of pregnancy. 1
The first documented case was described in the 16th century in a newborn who presented an encephalocele (EC) in the context of a serious clinical picture, today known as Robert syndrome. 2 After that, numerous references can be found in the literature concerning this condition, such as those of Le Dran (1740) who introduced the term hernia cerebri, Richter (1813) who described a case of nasal EC, and Spring (1854) who wrote what was probably the first extensive monograph on the subject. 3 Several theories have alternated in order to explain the pathogenesis. In 1827, Saint-Hilaire supposed that an increased intrauterin pressure could cause adhesions between the brain and the germinal membranes, arresting the development of the anterior cranium and allowing herniation of the brain through this opening. Other subsequent speculations pointed to increased organ bulk, ventricular dilatation, rickets, hydrocephalus, and olfactory bulbs residues as putative causes of ECs formation. 4 There have been numerous attempts at classification as well, such as those of Heinecke (1882), Browder (1932), Blumenfeld (1965), until the last more comprehensive classification by Suwanwela and Suwanwela in 1972 which is still used today with minimal rearrangements. 3
33.2 Classification and Distribution
Encephaloceles have been classified in several subtypes depending on different features.
Related to the onset, ECs may be congenital or acquired. Congenital (primary) ECs are the consequence of a defect in the development of the skull during embryogenesis, particularly in the closure of the neural tube. Prevalence is about 0.4–8/10,000 alive infants. 5 Acquired (secondary) ECs are usually a consequence of head traumas, even if this is a rare circumstance in children, thanks to the high plasticity of the skull at this age, with an estimated incidence of 0.2%–0.3% of all cerebrospinal fluid (CSF) leaks after trauma in the pediatric population. 6
Depending on the herniated content and its histology, ECs are divided into meningocele (herniation of meninges and CSF), meningoencephalocele (herniation of brain tissue and meninges) and hydromeningoencephalocele (herniation of a portion of a ventricle, brain tissue, and meninges). 5 Treatment approaches to these entities are functionally identical and a distinction is often not drawn in the clinical literature.
Lastly, ECs are classified based on the location and type of skull defect. They can occur either at the vault and the base of the skull. A commonly accepted system arranges ECs into (1) frontoethmoidal (also known as sincipital), (2) basal, (3) of the cranial vault, and (4) occipital (▶ Table 33.1).
Frontoethmoidal EC | Nasofrontal Nasoethmoidal Naso-orbital |
Basal EC | Transethmoidal Transsphenoidal Sphenoethmoidal Sphenomaxillary Frontosphenoidal/spheno-orbital |
EC of the cranial vault | Interfrontal Anterior fontanelle Interparietal Posterior fontanelle Temporal |
Occipital EC | |
Abbreviation: EC, encephalocele. |
Racial and geographic factors seem to influence the site and the frequency. Indeed, even differences in seasonal patterns or socioeconomic status are supposed to be responsible for the differences in distribution. 7
About 80% of all ECs occur in the occipital area of the cranial vault. 8 In Southeast Asia, parts of Russia and central Africa, sincipital defects are more common than occipital ones with an incidence of 1/3,500 live births. Contrarily, occipital lesion is the predominant type in the white races of North America, Europe, and Australia, representing almost the 85% of all observed ECs. 3 , 9
This chapter will focus predominantly on congenital frontoethmoidal and basal ECs, collectively known as nasal encephaloceles, with frontoethmoidal being the most common type of nasal EC encountered, especially in the nasofrontal region. 10 , 11 Overall, basal defects are rare in all racial groups (2–10% of all reported cases).
These categories, along with their subtypes, are defined based on the specific location of the associated bony defect.
Frontoethmoidal ECs, which are associated with a skull defect at the foramen cecum, are anterior to the cribriform plate and formed by a herniation of the soft tissues of the forehead and nose, and orbit through a defect in the frontal and ethmoid bones. These can be nasofrontal, nasoethmoidal, naso-orbital. 12
Basal ECs, in contrast, protruding posteriorly to the foramen caecum, present in the nasal cavities as opposed to external masses. Basal ECs include transethmoidal (herniation through the cribriform plate), sphenoethmoidal (herniation at the sphenoethmoid junction), transsphenoidal (herniation through the sphenoid body into the sphenoid sinus or nasopharynx), frontosphenoidal/spheno-orbital (herniation through the superior orbital fissure), sphenomaxillary (herniation through the junction of the sphenoid body and wing into the pterygopalatine fossa) 13 (▶ Fig. 33.1).

A separate mention should be made for the even rarer congenital temporal ECs that arise as a result of any malfunction in the normal bony genesis of the temporal bone. By far, the majority of them manifest in the area of middle ear tegmen and mastoid, where the petrous and squamous aspects of the temporal bone join. 14 Much more uncommon localizations involve the posterior plate of the petrous bone 15 and the petrous apex. 16 More in detail, petrous apex ECs, described either as meningocele or arachnoid cyst, shows connection to Meckel’s cave and may determine secondary erosion of the petrous bone and carotid canal. 17
Lastly, multiple ECs may coexist complicating the clinical picture.
33.3 Etiology and Embryological Considerations
To date, etiology is still poorly understood but a multifactorial theory, involving genetic and acquired factors during pregnancy, is the most accredited. A number of environmental elements have been reported as being associated with ECs, including maternal malnutrition deficiencies, exposure to various teratogens (such as aflatoxin), advanced paternal age, and long intervals between pregnancies. 18 Other factors have been investigated, such as hyperthermia, viral infection, hypervitaminosis, hypoxia, irradiation, and folic acid insufficiency, but their role is not yet proven. 11
Some preliminary embryological considerations are necessary in order to better understand developmental anomalies of a complex and not yet fully clarified system.
Summarily, neural development is one of the earliest systems to begin and the last to be completed after birth. The nervous system (NS) develops when the notochord induces its overlying ectoderm to become neuroectoderm and to develop into the neural plate. The neural plate folds along its central axis to form a neural groove lined on each side by a neural fold. The two neural folds fuse together and pinch off to become the neural tube, open initially at each end (rostral and caudal neuropore), both closing before the end of the fourth week (neurulation process). Practically, the original ectoderm divides into three sets of cells: (1) the internally positioned neural tube, which will form the brain and spinal cord, (2) the externally positioned epidermis of the skin (surface ectoderm), and (3) the neural crest (NC) cells in the region that connects the neural tube and epidermis 19 (▶ Fig. 33.2). Before neural folds completely merge to form the neural tube, the rostral population of NC cells migrates to embryonic pharynx leading to the formation of five pairs of arches, each containing ectoderm, endoderm, and mesenchyme. NC cells of the first two arches contribute to cranial skeletal elements and associated connective tissue. 20 Around the fourth week post-conception, mesenchyme, derived from the paraxial mesoderm and NC, condenses to form the base of the ectomeningeal capsule. This process takes place well after the primordial brain, cranial nerves, eyes, and major intracerebral blood vessels have begun differentiation. 9 The further development of the cranium originates from ossification centers within the mesenchyme of the rudimentary skull, consisting in membranous neurocranium (that will form the flat bones of the skull), cartilaginous neurocranium or chondrocranium (that will form most of the cranial base), and viscerocranium (that will form the facial skeleton), each characterized by a specific ossification mechanism. 21

Two predominant hypotheses are currently suggested to explain ECs formation.
Neurulation disorder: Congenital ECs share the common finding of a median skull defect, which corresponds to the site where the neural tube closes along the midline. A disturbance at this level would result in sustained connections between neuroectoderm (brain) and surface ectoderm (epidermal layer of the skin). 22 In addition, Hoving postulated that the separation of neural and surface ectoderm occurs focally in the midline through apoptosis mechanisms and not in a zipper-like fashion. 23 Since an imbalanced separation process could happen at any site of the neural tube closure, various kinds of ECs might result.
Recently, several genes, such as SHH (Sonic Hedgehog), have been identified as possible players in the pathogenesis of ECs. SHH, a morphogenetic molecule produced by the notochord, represents an inductive signal for cell growth and differentiation, especially in the brain and spinal cord. During development, a ventrodorsal gradient of SHH directs the neural tube modeling and, by binding to its receptor, it prevents apoptosis. 24
2. Post-neurulation disorder: Since ECs are always covered with skin, mucosa, or at least an epithelial layer, the neural tube must have already closed before EC formation. 22 , 25 Indeed, Marin-Padilla suggested that a mesodermal insufficiency might be the first event leading to a growth impairment of the chondrocranium and to a delay in the closure of the membranous neurocranium. The subsequent explosive growth of the NS may eventually surpass the accommodative capacity of the neurocranium, and neural tissue may then herniate through the mesenchyme and extracranially. 26
33.4 Clinical Presentation
Nasal ECs manifest across a broad clinical spectrum, from barely perceptible midface, intranasal or intrapharyngeal masses to severe craniofacial deformity, often evident at birth. However, they may sometimes be asymptomatic.
In detail, most of frontoethmoidal ECs present as skin-covered masses or visible protrusions on the face, over the nose, glabella, or forehead. They show a positive Furstenberg sign, in which compression of bilateral internal jugular veins or Valsalva maneuver leads to enlargement of the protruding mass due to its connection to the subarachnoid space. In other cases, frontoethmoidal ECs present as intranasal, transilluminative, compressible masses near the nasal bridge often associated with hypertelorism. 13
Basal ECs, on the other hand, are not clinically visible due to their location within the nasal cavities and may present with upper airway obstruction. Other symptoms include snoring, oral breathing, feeding difficulties, and CSF rhinorrhea. 22 As these symptoms may be common in children for other reasons, the definitive diagnosis might be delayed, increasing the risk of dangerous complications. Meningitis or encephalitis develops due to direct communication of the central NS with the external environment, facilitating the entry of pathological microorganisms. The bacteria most commonly associated with such patients are Streptococcus pneumoniae, followed by Staphylococcus aureus and Neisseria meningitidis. 3 It is worth to note that recurrent episode of meningitis, even in absence of an active rhinorrhea, has to induce the suspect of a CSF leakage.
Herniation in transsphenoidal ECs may moreover result in endocrine dysfunction or disruption in optic pathways. 22 , 27 Hypothalamic–pituitary dysfunctions have been observed above all in growth hormone (GH) and antidiuretic hormone (ADH) levels and to a lesser extent in gonadotropins, thyroid stimulating hormone (TSH), and prolactin. Possible causes may be the stretch of pituitary stalk and hypothalamus, with a mechanism similar to the empty sella syndrome, and other unknown congenital conditions. 28
The majority of nasal ECs is sporadic, although a small percentage occurs associated with other craniofacial malformations (▶ Fig. 33.3). About one-third is associated with midline defects such as cleft lip and palate. 5 Additional intracranial anomalies have been reported, mainly observed with frontoethmoidal ECs, with hydrocephalus and corpus callosum agenesis being the most frequent. 10 , 29

ECs may also manifest as a part of recognized clinical syndromes, among which the most frequently described are briefly reported here.
Septo-optic dysplasia (or de Morsier syndrome) is a rare, congenital, and very heterogeneous syndrome, with a reported incidence of 1 in 10,000 births. The diagnosis can be reached when two or more features of the classical triad of optic nerve hypoplasia, pituitary hormone abnormalities, and midline brain defects (including agenesis of the septum pellucidum and/or corpus callosum) are present. 30 , 31
Morning glory syndrome is a rare congenital malformation of the optic disc. It is characterized by an enlarged, funnel-shaped optic disc and an elevated pigmented peripapillary tissue annulus, often associated with craniofacial abnormalities, optic nerve atrophy, coloboma, and megalopapilla. 28
Moyamoya disease is a progressive, steno-occlusive arteriopathy involving the internal carotid artery terminus and its branches that can cause recurrent strokes in children. 32 It is a rare disorder, with an estimated annual incidence of 0.35 to 0.94/100,000 people, often associated with ocular malformations and midline cranial defects. 29 , 33
Furthermore, the literature reports cases of nasal ECs associated to other much rarer syndromes, such as craniosynostosis, 5 Dandy–Walker complex, 34 Hunter, 35 Aicardi, 36 Goldenhar 37 syndromes, and lastly with cardiovascular and kidney malformations. 34
33.5 Diagnosis
A suspect of nasal EC can derive from a detailed clinical evaluation, although a diagnostic confirmation can be reached only through further investigations.
Endonasal endoscopy, either with flexible or rigid-angled scopes, can reveal the presence of an intranasal, usually translucent and pulsating, mass occasionally associated with evident CSF rhinorrhea. β2-transferrin or β-trace protein dosage on nasal secretion may represent an additional non-invasive and highly predictive tool to confirm CSF leakage, even in absence of a nasal neoformation. However, limits of this test are that rhinorrhea has to be active and that it is not always so easy to collect nasal fluid samples, especially in pediatric patients.
To better visualize the soft tissue mass, and its contents and boundaries, high-resolution computed tomography (CT), or cone beam CT, in conjunction with magnetic resonance (MR) is recommended in almost all the cases, considering the complementarity of the information provided. 38 Multislice CT scan produces details about the craniofacial skeleton morphology and often allows the identification and the site of a bony defect. Moreover, it functions as operative guide for the surgeon. MR, giving details about soft tissues, is more accurate in enhancing the presence of a brain herniation and a CSF leakage and in excluding other possible coexisting encephalic malformations. In particular T2-weighted, T2-flair, and CISS sequences provide excellent contrast between CSF and other encephalic structures, and they are essential to differentiate between CSF and inflammatory signals in the nose and paranasal sinus cavities; gadolinium sequences allow to rule out tumors. Moreover, and this applies to both the radiological examinations mentioned, the entire skull base (including middle ear roof and mastoid, middle, and posterior cranial fossa) must be checked out in order to detect any multiple skull base defects 6 (▶ Fig. 33.4).

Biopsy is absolutely contraindicated before radiologic evaluations are completed and a diagnostic hypothesis advanced, as it can provoke severe complications, such as CSF leak and ascending infection due to the persisting intracranial communications between the mass and the cranial content. 38 Preoperative intrathecal fluorescein test is rarely necessary.
Histologically, ECs have shown to contain glial cells, cerebral tissue, non-functional neural tissue, choroid plexus, and ependymal cells. Cystic structures with ependymal cells are a distinguishing feature of EC in comparison to gliomas. Glial tissue can be visualized using immunoreactivity for GFAP, S-100 protein, and NSE. Nerve and nasal septum cartilage may also be present. 8
Differential diagnosis should include mainly nasal polyps, nasal gliomas, or dermoid cysts. 39 A special mention has to be made for these latter two entities, since they are supposed to share the same pathogenetic mechanism with frontoethmoidal ECs. As discussed above, skull base and facial skeleton development results from a complex interaction of cellular proliferation and regression involving migration of NC cells through ectodermal and mesodermal derived structures. Anteriorly, as the frontal, nasal and ethmoid bones, and nasal capsule fuse together, potential spaces are created that normally regress by birth. Persistence of these spaces including the fonticulus nasofrontalis (region between frontal and nasal bones), the prenasal space (region between nasal bones and nasal capsule), and the foramen cecum (region between frontal and ethmoid bone) may lead to the herniation of intracranial contents or glial tissue, forming either ECs or gliomas. Alternatively, ectodermal and mesodermal tissue may be entrapped in these spaces, leading to the formation of dermoids. 40 From a clinical standpoint, dermoids are the most common midline congenital nasal mass and can present as a non-pulsatile cyst, sinus, or fistula on the external nose (30% of dermoids can communicate with the dura). On the other hand, gliomas represent herniated glial tissue along the skull base and facial skeleton fusion planes but do not have any communication with the CSF. They present clinically as non-pulsatile masses frequently extranasal, but they can be also intranasal or combined (up to 15% of gliomas has attachments to the dura). 41
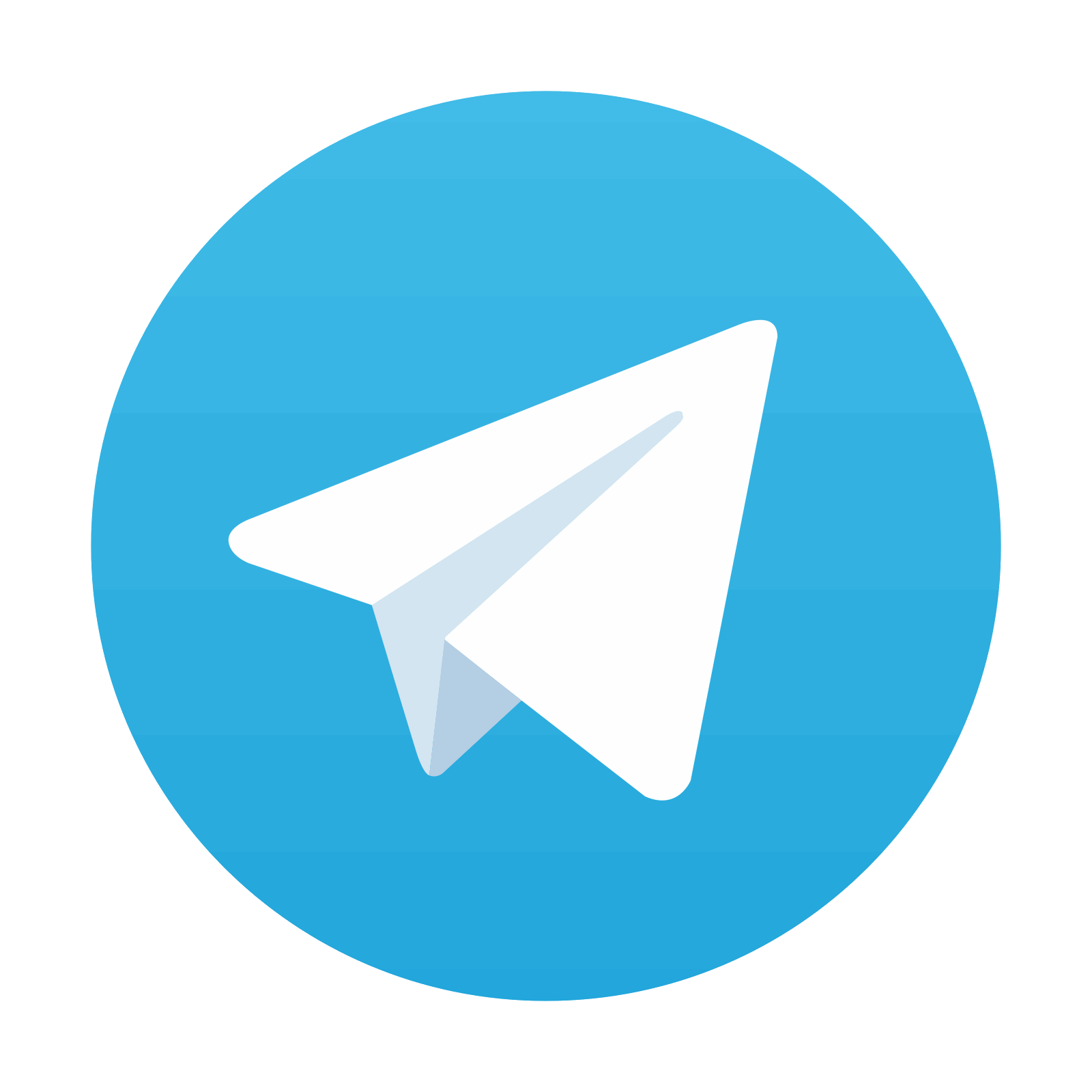
Stay updated, free articles. Join our Telegram channel

Full access? Get Clinical Tree
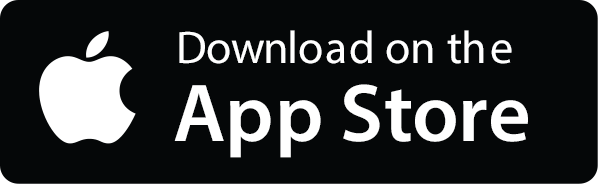
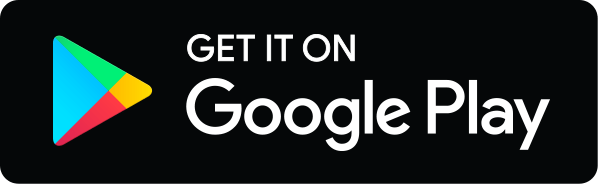