3 Physiology of the Thyroid Gland
3.1 Introduction
The mature thyroid gland lies anteriorly, inferiorly, and laterally to the laryngeal thyroid cartilage in the neck. The shape of this cartilage, reminiscent to some of an ancient Greek shield (thureos), is the source of the name thyroid, which was used for the first time in 1656 by Thomas Wharton. 1
Although Graves’ disease findings were first documented at the end of the 18th and beginning of the 19th centuries, it was only near the close of the 19th century, with the publication of the report of the Myxoedema Commission of the Royal Society of London, 2 that the thyroid gland’s function began to be understood. The concept that a very small gland was capable of producing an “internal secretion” that could regulate animal and human metabolism stems from this period.
We now know that the thyroid gland concentrates iodide, synthesizes and stores the iodine-rich hormones tetraiodothyronine, also referred to as thyroxine and l-thyroxine (T4), and triiodothyronine (T3), and releases them into the circulation by highly regulated processes. These hormones have effects in most cells of the body, controlling oxidative metabolism and the expression of many genes that are specific to differentiated cells.
This chapter reviews the physiology of the thyroid, from its cell structure and organization, to its cellular processes and the regulation of its secretion. It also introduces how the measurement of hormones from the thyroid axis can aid in the diagnosis of thyroid diseases, and reviews how special circumstances in normal physiology, such as aging, pregnancy, and diet, can affect the function of the thyroid hormone axis and the tests that we use to measure that function.
3.2 The Cellular Structure of the Thyroid
The secretory functions of the thyroid are carried out by two distinct cell types of differing origin. The classic thyroid hormones T3 and T4 are products of the follicular cells. These are embryologically derived from the endoderm of the primitive foregut in the region of the posterior pharynx at the base of the tongue. They migrate to their mature position along a tubular stalk, the thyroglossal duct, which ultimately is reabsorbed and disappears. 3 Follicular cells, the bulk of the thyroid secretory cell population, form follicles that are the basic secretory and storage units for thyroid hormone. Follicles are composed of tightly adherent cuboidal to columnar epithelial cells, which form a “picket fence” structure around a lumen. Each cell is oriented with a basolateral membrane adjacent to the microvasculature and an apical border composed of microvilli (the “brush border”) extending into the lumen. The microvilli increase the surface area between the cells and the lumen, which contains thyroglobulin. It is here that iodide (I–), which has been concentrated within the follicular cell cytoplasm, is combined with the thyroglobulin to form the active thyroid hormones (Fig. 3.1).

Interspersed between the follicles are the parafollicular C cells, which produce the calcium-modulating hormone calcitonin. Embryologically, C cells are of neural crest origin. They form bilaterally from the ultimobranchial bodies, which are derived from the fourth pharyngeal pouches and then migrate to lie in their mature position between the follicles (see Fig. 3.1). 4
3.3 Thyroid Hormone Synthesis and Release
Thyroid hormones are the only iodine-containing human hormones and require an adequate dietary supply of this environmentally scarce element for their biosynthesis. Iodide (I–) is the essential rate-limiting substrate for thyroid hormonogenesis. Sophisticated biomechanisms have evolved for the uptake and concentration of iodide into the thyroid follicular cell, its insertion into tyrosine to form mono- and diiodotyrosine (MIT and DIT) and their subsequent coupling into the thyroid hormones T3 and T4 (Fig. 3.2).

The sodium (Na)/iodide symporter (NIS), a 643 amino acid 108 kDa dimerized transmembrane glycoprotein, is located at the basolateral membrane of the thyroid follicular cell adjacent to the microvasculature. 5 , 6 It is an adenosine triphosphate (ATP)-driven active transport protein that simultaneously transports Na+ and I– (in a 2:1 ratio) into the cytoplasm. This concentrates I– against an electrochemical gradient and results in an intra- to extracellular I– concentration ratio of up to 40:1. 7 NIS activity, demonstrable as early as the onset of fetal thyroid hormonogenesis at about the 12th week of gestation, 8 is stimulated by thyrotropin-stimulating hormone (TSH) of pituitary origin and inhibited by anions, such as thiocyanate (SCN–) and perchlorate (CLO4 –). 9 Importantly, when excessive iodine exposure leads to high intrathyroidal I– concentrations, a block of I– oxidation and organification occurs, leading to reduced hormone synthesis (the Wolff–Chaikoff effect). 10 It has been proposed that this same increased intrathyroidal I– concentration also inhibits NIS activity, resulting in decreased I– transport into the cytoplasm, thereby reducing intrathyroidal I– levels and enabling an “escape” from the Wolff–Chaikoff effect. 11 In this manner, I– itself is able to autoregulate NIS activity. While crucial to thyroid function, NIS activity has also been identified in other tissues, such as the lactating breast, salivary glands, stomach, and small intestine. 9 NIS-mediated iodine uptake and concentration within the follicular cell is the basis for thyroid radioiodine diagnostic testing as well as treatment of those differentiated thyroid cancers expressing adequate NIS activity. Recent reports have suggested that facilitation of radioiodine treatment by upregulation of poor NIS expression may be possible in some tumors with the use of tyrosine kinase inhibitors. 12
From the follicular cell cytoplasm, I– is further translocated across the apical cell brush border membrane into the follicular lumen. The characterization of the apical membrane transporter(s) is yet to be fully defined but is thought to involve the transmembrane transporter pendrin (also known as the sodium-independent chloride/iodide transporter), 13 a 780 amino acid 115 kDa glycoprotein, and possibly other anion transport channels, such as anoctamin-1. 14 As is true for NIS, pendrin activity is stimulated by TSH. Mutations in the gene encoding pendrin have been linked to Pendred syndrome of goiter, hypothyroidism, sensorineural hearing loss, and impaired I– organification. 15 , 16
Within the lumen at the apical border, I– is oxidized and then organified 17 onto tyrosyl residues at their 3- and 5-phenyl ring positions within the colloid matrix, which is composed of the rough endoplasmic reticulum (RER)-processed 660 kDa dimerized glycoprotein thyroglobulin. 18 This oxidation and organification is catalyzed by the heme-containing apical enzyme thyroperoxidase (TPO) in the presence of hydrogen peroxide (H2O2), resulting in the formation of the biologically inactive MIT and DIT. 19 The required H2O2 is generated by the calcium-dependent 180 to 190 kDa glycoprotein dual oxidases DUOX1 and DUOX2, which are co-localized with TPO at the apical border. 20 , 21 DUOX activity is upregulated by TSH and low I– concentrations and downregulated by high I– concentrations. This has been proposed as a partial explanation of the Wolff–Chaikoff phenomenon. 22 TPO then further catalyzes the coupling of MIT and DIT by placing an ether bond between two phenyl rings, forming the active thyroid hormones T3 and T4, which are still attached to the thyroglobulin protein backbone. 17 TPO enzymatic activity is also stimulated by TSH, whereas it is inhibited by the antithyroid thionamide drugs propylthiouracil (PTU), methimazole (MMI), and its metabolic precursor carbimazole (CBZ). 23 This thionamide inhibition of TPO activity forms the basis for the oral antithyroid drug treatment of hyperthyroidism.
The iodothyronines (T3, T4, MIT, and DIT) remain stored within the thyroglobulin colloid reservoir until needed and are then brought into the cytoplasm by endocytosis and proteolytically hydrolyzed within lysosomes. The resulting T3 and T4 are secreted into the vasculature through the basolateral membrane by the active transmembrane monocarboxylate transporter 8 (MCT8). 24 MCT8 is expressed in many tissues, including thyroid, brain, kidney, heart, and skeletal muscle. The Allan–Herndon–Dudley syndrome of mental retardation, hypotonia/dystonia, and abnormal thyroid hormone levels has been linked to a loss-of-function mutation in the gene encoding MCT8. 25 , 26 Those MIT and DIT residues remaining in the cytoplasm undergo dehalogenation by the enzyme DEHAL1, with the liberated I– recycled into the colloid for further hormonogenesis. 27 The complex intracellular pathways accomplishing these tasks are shown schematically in Fig. 3.3.

T4 and T3 are secreted by the thyroid in molar proportions variously calculated as 14:1, 28 or more recently as 17:1. 29 However, this direct thyroidal secretion accounts for only 20% (~ 6 µg) of the circulating T3, whereas the other 80% (~ 25 µg) is the result of peripheral conversion of T4 to T3 by 5´-deiodination of T4. 28
3.4 Thyroid Hormone Deiodination
Secreted T4 may be considered a prohormone and is converted to the active T3 form by deiodination at the 5´ position on its outer phenyl ring. This is accomplished by the selenodeiodinases type 1 (D1) and, more robustly, by the higher-affinity type 2 (D2). Deiodination at the 5 position on the inner ring is accomplished by type 3 (D3), and to a lesser extent by D1, and leads to the inactive molecule reverse T3 (Fig. 3.4). These significantly tissue-specific deiodinases maintain thyroid hormone homeostasis and regulate thyroid-mediated intracellular activity. They are all structurally similar membrane-bound proteins of 29-33 KDa. D1 and D3 have a transmembrane domain at the cell surface, whereas D2 is located at the endoplasmic reticulum. All have their functionally active centers in the cytosol. D1 is present in several tissues, such as liver, kidney, thyroid, and pituitary, but it is not present in the central nervous system (CNS). D2 activity is present in the CNS as well as in the peripheral tissues, such as thyroid, heart, skeletal and vascular smooth muscle, kidneys, placenta, and pancreas. D3 is found in the CNS as well as placental and fetal tissues. 30 , 31 , 32 Alterations in deiodinase activities are thought to play an important role in such pathophysiological conditions as the nonthyroidal illness syndrome.

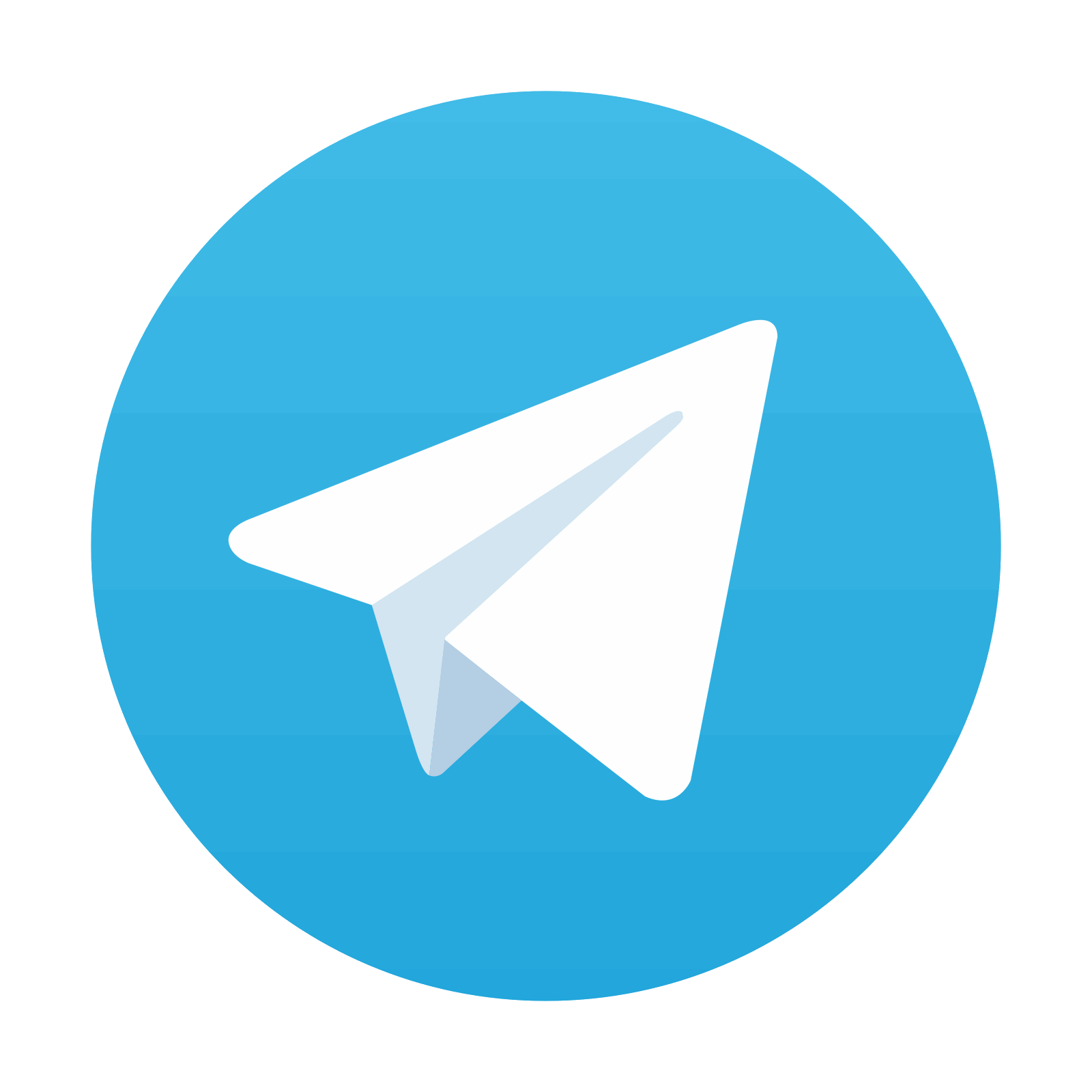
Stay updated, free articles. Join our Telegram channel

Full access? Get Clinical Tree
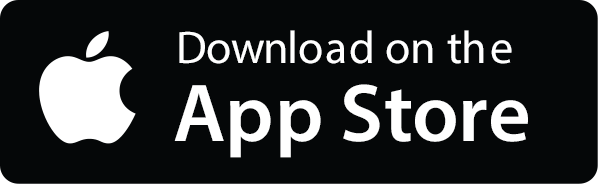
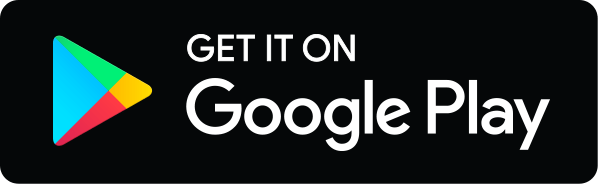