23 Parathyroid Imaging
23.1 Introduction
Much time has passed since the days when palpation and barium swallow studies were the preoperative localization methods of choice for primary hyperparathyroidism. Thermography, selenomethionine scintiscanning, and esophageal cineradiography have likewise joined their ranks in the history books. 1 Today, the modalities of choice for parathyroid imaging include ultrasound and technetium-99m (99mTc)-sestamibi (sestamibi) imaging, and, more recently, four-dimensional-computed tomography (4D-CT) scanning. In combination with the development of intraoperative parathyroid hormone measurement, advances in parathyroid imaging enabled the adoption of focal parathyroid exploration as a surgical strategy. At present, whether surgery is focal or bilateral exploration, 2 , 3 , 4 the role of parathyroid imaging remains critically important. Preoperative knowledge of single versus multigland disease, concomitant thyroid pathology, and ectopic gland location is critical to achieving operative success.
Parathyroid imaging must be performed in the correct context. It is used for localization, to supplement and aid surgical dissection, but never to help attain a diagnosis. The biochemical diagnosis of hyperparathyroidism should be made prior to embarking on localization, and imaging should be pursued in those patients who are surgical candidates. The number and type of imaging studies to perform are based largely on surgeon preference and institutional availability. Study performance and sensitivity are also important, although one must remember when interpreting data on imaging performance and sensitivity that the sensitivity of all modalities is dependent on intraoperative findings, which are influenced by whether or not bilateral exploration was pursued. The prevalence of multigland disease influences the perceived accuracy of localizing studies, 5 and it varies with the thoroughness of the surgical exploration. 6
Imaging must also be interpreted with a thorough understanding of parathyroid embryology and anatomy. Most patients have four glands, although supernumerary glands have been reported. 7 The superior glands are derived from the fourth branchial pouch along with the lateral lobes of the thyroid; the inferior glands arise from the third pouch along with the thymus. Superior glands are located in a more posterior plane when compared with inferior glands, and this plays an important role when reviewing imaging, especially cross-sectional or sagittal images. Although superior glands are typically located behind the midportion of the superior thyroid lobe near the cricothyroid junction, they can also descend into a relatively inferior cervical location. Recognizing this posterior position in the neck can help distinguish them from inferior parathyroid glands, which are typically also found in the low neck in close proximity to the inferior pole of the thyroid, although in a more anterior plane. Likewise, inferior glands that reside within cervical or mediastinal thymic tissue will remain in a relatively anterior plane.
This chapter focuses on the most common parathyroid imaging modalities used today and their roles in both primary and reoperative cases.
23.2 Ultrasound
Reports from 1975 and 1978 were the first to detail the use of ultrasonography to identify parathyroid glands > 5 mm in size, 8 , 9 and Barraclough et al provided additional support in 1981. 1 For many years now, it has often been the first modality used to detect abnormal parathyroid glands and can be performed in the outpatient office setting. Although radiologists have classically performed or interpreted ultrasounds, surgeon-performed ultrasound is becoming increasingly common.
The patient is positioned supine on the examination table with a pillow positioned at the level of the shoulders to allow for neck extension. A high-frequency linear array transducer of 7.5 to 15 MHz is used to systematically scan the neck to identify both thyroid and parathyroid abnormalities. Normal parathyroid glands are typically too small and structurally similar to adjacent thyroid tissue for sonographic detection, suggesting that almost any gland identified is inherently pathological in nature. On gray-scale imaging enlarged parathyroid glands are uniformly hypoechoic and classically ovoid or teardrop in shape with well-defined margins created by a hyperechoic line of adventitia along the anterior and posterior surfaces (Fig. 23.1). 10 Large adenomas can appear bilobed or irregularly shaped. 11 , 12 Cystic degeneration can be found in 1 to 2% of enlarged glands. 13 Color Doppler imaging is used to identify the vascular pedicle, the enlarged, extrathyroidal feeding artery that typically arises from branches of the inferior thyroidal artery. This feeding artery typically supplies the adenoma at the pole of its long axis, in contrast to lymph nodes, which have a central, hilar blood supply. 10 , 11 , 12 , 14 Vascular arcs surrounding parathyroid glands from 90 to 270° have also been described in more than 50% of adenomas. 15 These arcs arise from arborization of the feeding artery around the periphery before smaller braches penetrate deeper, and were first described in angiography literature (Fig. 23.2). 12 , 16 Although these features can be confirmatory in cases of large, more obvious parathyroid adenomas, their value is often greatest for lesions that are smaller and less readily identifiable as an enlarged parathyroid. 11 , 12 , 14


Gradually applying enough pressure with the ultrasound probe to gently deform the subcutaneous tissue and strap muscles can help elucidate glands that might be otherwise difficult to see, such as those deep in the tracheoesophageal groove or laterally in the carotid sheath. 12 This technique can be valuable even when glands are obvious; enlarged glands may be compressible and can therefore be seen to “move” or change shape during ultrasound with gradual compression by the sonographer. Conversely, enlarged lymph nodes do not exhibit this same deformability.
Superior parathyroid glands are generally found posterior to the upper or middle third of the thyroid lobe and can sometimes be more readily identified with the probe in longitudinal orientation. Inferior glands are typically found immediately adjacent to the lower border of the thyroid lobe or in the fibrofatty central neck tissue. 12 Lymph nodes can also be frequently identified in the central neck spaces and may be difficult to distinguish from enlarged parathyroid glands. One clue to distinguish lymph nodes from parathyroid glands is to examine the echogenicity of the thyroid gland. Coarse thyroid echogenicity can signify an underlying thyroiditis, such as Hashimoto’s thyroiditis. In these cases it is not uncommon to find multiple enlarged lymph nodes in the central neck spaces. In general, lymph nodes tend to be more round in shape than parathyroid glands, and those associated with subacute thyroiditis may demonstrate central, linear vascular flow that corresponds to the hilum. Lymph nodes in patients with Hashimoto’s thyroiditis typically lack any blood flow, reflecting the chronic nature of the inflammatory process. 17
Given its low cost, ease of administration, and lack of ionizing radiation, ultrasound is rapidly becoming the preferred parathyroid imaging modality; many view it as a mandatory localization strategy. 18 , 19 This is especially true among surgeons because the superior anatomical resolution provided by real-time ultrasound can be invaluable in operative planning and execution. Sensitivity of surgeon-performed ultrasound is high, ranging from 76 to 88%, 20 , 21 , 22 and has been reported by some to be higher than that of radiologist-performed ultrasound. 23 , 24 , 25 Gland size, weight, and volume can predict the likelihood of sonographic detection, as can sonographer skill. Patient obesity; small, multiple, hyperplastic, or ectopic glands; and thyroid nodular disease are all limitations to successful ultrasound. 26 However, visualization of concomitant thyroid pathology, which occurs in 25 to 84% of primary hyperparathyroidism patients and is malignant in nearly 20% of cases, can also be beneficial in the preoperative setting (Fig. 23.3). This knowledge can alter the planned operative approach, help to avoid unnecessary thyroid surgery for lesions first encountered intraoperatively, and potentially avoid future reoperative neck surgery for missed pathology. 27 , 28 Milas et al determined that preoperative detection and biopsy of thyroid nodules decreased concomitant thyroidectomy to 6%, as opposed to 30% in those who had thyroid nodules discovered intraoperatively at the time of parathyroid surgery. 27 Doppler flow can help differentiate thyroid nodules (which lack a polar vascular pedicle) from parathyroid adenomas, as can identification of a well-defined tissue plane separating a parathyroid gland from the thyroid. Thyroid nodules are more likely to be heterogeneous in echotexture, as opposed to the uniform hypoechoic nature of a parathyroid adenoma. Ultrasound can also be useful in identifying intrathyroidal parathyroid glands and facilitating fine-needle aspiration biopsy if necessary. Finally, ultrasound can help differentiate nodular thyroid disease from parathyroid activity, which may be difficult to distinguish on nuclear scintigraphy imaging alone.

Ectopic glands, especially those located in retroesophageal, intrathymic, and mediastinal locations, as well as multigland disease, reduce the overall success of ultrasound. The upper mediastinum and posterior clavicular spaces may be better imaged with a curved probe. Deglutition during examination can help elevate glands in these locations into the field of view. 29 Color flow Doppler imaging is less beneficial for ectopic glands in the superior mediastinum because these glands are frequently supplied by the thymic branch of the internal mammary artery and are not sonographically accessible. Adjunctive measures, including axial rotation of the patient’s head, may be helpful when searching for ectopic glands. However, interference by osseous structures and those poorly penetrated by ultrasound (esophagus and trachea) can still ultimately limit identification. 30 Care must also be taken to evaluate the high cervical region near the carotid bifurcation and along the thyrothymic ligament in the central neck. 11
Multigland hyperplasia can be difficult to image because these glands are typically smaller than adenomatous glands, and all involved glands are rarely visualized. In patients with asymmetric hyperplasia or double adenomas, finding of a single enlarged gland on ultrasound may lead to the incorrect conclusion that only one diseased gland is present. 4 , 31 Conversely, multigland disease in the setting of secondary and tertiary hyperparathyroidism can lead to four sonographically identifiable glands.
23.3 Nuclear Scintigraphy
Radioisotope imaging of the parathyroid glands requires a source of radioactivity, known as the tracer, as well as a camera with which to capture the emitted radiation. Following marginal success with selenium-75-methionine, thallium-201 (201Tl) was the first radiotracer to gain widespread acceptance for parathyroid localization. Originally used for myocardial perfusion imaging, 201Tl is a potassium analogue that is rapidly integrated into the intracellular potassium pool. 32 Densely hypercellular tissues, such as enlarged parathyroid glands, as well as thyroid tissue, accumulate 201Tl. Today, use of 201Tl has been abandoned in favor of technetium-99m (99mTc)-sestamibi (sestamibi) due to superior image quality, improved accuracy, and more favorable dosimetry. 32 A lipophilic compound that becomes trapped intracellularly, primarily by sequestration in the mitochondria, 33 sestamibi was originally designed to be a myocardial perfusion agent; its uptake by parathyroid tissue was serendipitously discovered. Coakley et al reported the first use of sestamibi in parathyroid imaging in 1989. 34 Although, like 201Tl, it is taken up by both parathyroid and thyroid tissue, sestamibi washout time from parathyroid tissue is longer due to mitochondrial-rich oxyphil cells in hypercellular parathyroid glands. 35 Additionally, owing to the superior physical characteristics of sestamibi for gamma camera imaging, the radiation dose to the patient per unit administered is approximately 20 times less than that for 201Tl. 34
99mTc-tetrofosmin (tetrofosmin), another myocardial perfusion agent, has also been used for parathyroid scintigraphy. Parathyroid tissue avidly takes up tetrofosmin but dissipates it more quickly than sestamibi. 36 , 37 Despite this rapid clearance, some have indicated that the isotopes have similar sensitivities. 38 , 39 Most, however, feel tetrofosmin is significantly less sensitive 40 and less accurate in orienting the surgeon to the diseased gland, 41 and therefore use sestamibi instead.
The techniques used in parathyroid scintigraphy have evolved over time, and there are multiple protocols that remain in use. The two basic protocols are dual-isotope single-phase (subtraction) and single-isotope dual-phase (washout). Both techniques serve to isolate activity attributable to just the parathyroid glands because 201Tl, sestamibi, and tetrofosmin all have affinity for both thyroid and parathyroid tissues. Subtraction was popularized by the introduction of 201Tl for parathyroid scintigraphy in 1983, and relied on concomitant use of technetium-99m (99mTc)-pertechnetate chloride (pertechnetate), which accumulates in the thyroid but not the parathyroid glands. 201Tl and pertechnetate are administered in a single session, without moving the patient. The pertechnetate, or thyroid image, is then subtracted from the 201Tl, or parathyroid image. Residual activity represents abnormal parathyroid tissue. 42 Iodine-123 (123I) is also used for subtraction, which, along with pertechnetate, is concentrated in thyroid cells via the thyroid Na+/I- symporter, although only 123I is organified in thyroid follicles. 43 Because of its similar half-lives in both thyroid and parathyroid tissue, tetrofosmin requires that a subtraction protocol be used, as opposed to a washout protocol, for which sestamibi is suitable. 44
Subtraction imaging may be most useful in patients with thyroid abnormalities because thyroid nodules are the most frequent cause of false-positive results in parathyroid scintigraphy. 32 , 45 Many thyroid lesions that accumulate sestamibi or tetrofosmin also accumulate pertechnetate and iodine and can therefore be subtracted. The subtraction technique, though useful, has certain limitations. Patient motion during data acquisition may lead to registration errors of the sestamibi/tetrofosmin and pertechnetate/iodine images, resulting in a false-positive study. Another pitfall of the subtraction technique is decreased or absent thyroid uptake of pertechnetate or iodine, which may render the subtraction image invalid. A detailed thyroid history is valuable, including thyroid hormone supplementation or recent computed tomographic (CT) scan with iodinated contrast. Very intense sestamibi or tetrofosmin uptake by a parathyroid lesion may occasionally cause “shine-through” on the pertechnetate/iodine image and be erroneously interpreted as a thyroid nodule. Occasionally, a parathyroid lesion will be situated immediately behind a thyroid lesion and is eliminated when the thyroid image is subtracted. 32
Taillefer et al introduced the single-isotope dual-phase imaging (washout) technique in 1992 based on the observation that the washout of sestamibi from abnormal parathyroid tissue is slower than that from surrounding thyroid tissue. 46 This simple and easy-to-perform study requires only a single injection of sestamibi followed by imaging approximately 15 minutes and 1.5 to 3 hours later. A persistent focus of activity on delayed imaging is indicative of a parathyroid lesion (Fig. 23.4). Unfortunately, some parathyroid lesions do not retain sestamibi, whereas some thyroid lesions, and even cervical lymph nodes, accumulate and retain sestamibi, resulting in both false-negative and false-positive studies. 32 , 43 , 47 This technique also lacks sensitivity for detecting multigland disease. 48

The acquisition technique can also affect sensitivity of scintigraphy. Use of a fixed or mobile gamma camera will determine whether planar (two-dimensional) or three-dimensional images are acquired, respectively, whereas the type of collimator (lens equivalent of a gamma camera) used determines their resolution. Collimators filter emitted gamma rays into a singular direction so that a clear image is produced and spatial orientation is maintained, similar to a polarizing lens. Parallel-hole collimators filter a stream of parallel rays allowing for simultaneous imaging of both the neck and the chest. Pinhole collimators provide the highest resolution, limit imaging to the neck only, magnify the structures being imaged, and can also be positioned so as to obtain oblique views. 32 They are significantly more sensitive than parallel imaging for glands of all sizes, including multigland disease. 49 , 50
The addition of single-photon emission computed tomography (SPECT) allows for three-dimensional imaging by using a mobile gamma camera to collect images at multiple angles around the patient (Fig. 23.5). The multiple images are compiled by a computer and displayed as three-dimensional images, similar to positron emission tomography (PET) imaging. SPECT increases the sensitivity of scintigraphy compared to planar imaging alone. 51 , 52 The additional detail is useful for not only detecting but also localizing ectopic parathyroid glands, because tomography provides more detailed anatomical information about the gland, its size, and its relationship to other structures, including the sternum, spine, and heart. 53 It is also helpful in differentiating thyroid lesions from parathyroid lesions. Because the parathyroid glands are typically found posterior to the thyroid they can subsequently lie directly behind a thyroid nodule. Planar imaging alone is unlikely to distinguish an anterior thyroid lesion from a posterior parathyroid gland; tomography is able to differentiate these spatial relationships. 32

The latest adjunct to scintigraphy is hybrid SPECT/CT imaging, a modality combining nuclear medicine and CT technology into a single physically integrated instrument (Fig. 23.6). The CT portion provides an anatomical map, which, when merged with the radiotracer uptake demonstrated on SPECT images, aids in demonstrating the relationship of sestamibi-avid lesions to adjacent structures. Because CT and SPECT acquisition can be performed in a single imaging session without moving the patient, there is near-perfect coregistration of the images. Attenuation correction afforded by SPECT/CT improves the sensitivity for the detection of deeper lesions, and visualization of corresponding anatomy improves the differentiation of abnormal from physiologic sestamibi accumulation. 47 These advantages can lead to a significant reduction in false-positive findings over SPECT imaging alone. 54

The success of scintigraphy is dependent on the particular scanning technique used, and therefore reported sensitivities vary widely. Subtraction scanning has been demonstrated to be superior to single-agent dual phase washout. 55 , 56 , 57 Many advocate for the combined use of both planar and SPECT imaging. Nichols et al 58 noted that the use of SPECT imaging alone did not afford increased sensitivity from planar imaging, 88% versus 83%, respectively. However, the addition of SPECT to planar imaging led to the greatest sensitivity, specificity, and accuracy (90%, 89%, and 89%, respectively). Multigland disease continues to remain a major challenge in parathyroid imaging, and in this study every technique was significantly less sensitive in the detection of multigland disease (MGD) than in the detection of single-gland disease (SGD). 58 Specificity was also significantly lower for MGD than for SGD for interpretation of all images (73% vs. 98%, p < 0.001). 58 Lavely and colleagues also found that SPECT was not more sensitive than planar imaging, but they did find that SPECT/CT was significantly more sensitive than both planar and SPECT imaging (dual-phase imaging; subtraction protocol not used). 59 Sharma et al, in a study of 833 patients, found that, although accuracy for identifying SGD was equivalent between planar and SPECT modalities (SPECT, SPECT/CT, and SPECT 123I), all SPECT-based scans had a statistically higher rate of disease detection (i.e., lower false-negative rate) than planar imaging. 60
Regardless of the type of study performed there will always remain biological factors beyond the control of surgeons and radiologists that contribute to the sensitivity and specificity of scintigraphy. These factors, including size, weight, and oxyphil content of parathyroid glands, in addition to P-glycoprotein expression, have all been demonstrated to affect detection by scintigraphy. 41 , 61 , 62 , 63 , 64 , 65 Although positive scans are associated with adenomas of significantly larger volume and weight, many equally large glands can be missed, whereas glands of much smaller stature are identified. 26 , 58 , 61 , 63 , 64 These findings suggest there are other factors at play. Successful scintigraphy can be related to an adenoma’s oxyphil cell content; oxyphil cells are rich with densely packed mitochondria and rapidly sequester sestamibi. 61 , 63 , 64 Oxyphil content in parathyroid adenomas is variable, although pure oxyphil cell adenomas are rare. 66 Erbil et al demonstrated that oxyphil cell content > 20% was necessary to obtain positive sestamibi scan results in glands that were < 600 mg, whereas in glands > 600 mg, low oxyphil cell content did not affect imaging results. 61 In a similar fashion, expression of P-glycoprotein (P-gp) or multidrug resistance-related protein (MRP) can also affect parathyroid scintigraphy results independent of parathyroid size. Specifically, the retention of sestamibi in parathyroid cells depends on the activity of the P-gp coded on the multidrug resistance (MDR1) gene, which functions as an adenosine triphosphate (ATP)-dependent efflux pump, preventing the accumulation of lipophilic, cationic radiopharmaceuticals, including tetrofosmin and sestamibi. 41 Mitchell and colleagues elegantly demonstrated that it was not size, but rather another factor, such as P-gp expression, that was the primary determinant of parathyroid retention of sestamibi. 67 Sun and Kao, in two separate studies, went on to demonstrate that either P-gp expression, MDR expression, or both were present in every single parathyroid with a corresponding false-negative sestamibi scan. 62 , 68 Biochemistry is also important, although the correlation between preoperative parathyroid hormone (PTH) and calcium levels and scintigraphy results is variable. Some have found no statistically significant relationship. 26 , 61 , 64 , 65 , 69 , 70 Mehta et al suggested that PTH expression was greatest in glands consisting of predominantly chief cells, and therefore, given the corresponding reduced oxyphil cell concentration, tended to lead to negative studies. 63 Conversely, others have found higher preoperative calcium and PTH values are predictive of positive scans. 71 These biological factors are inherently nonmodifiable. However, use of levothyroxine to enhance the sensitivity of sestamibi imaging in euthyroid patients, and discontinuation of calcium channel blockers in patients with primary hyperparathyroidism, have both been suggested as ways in which the sensitivity of imaging can be altered at a biological level. 72 , 73
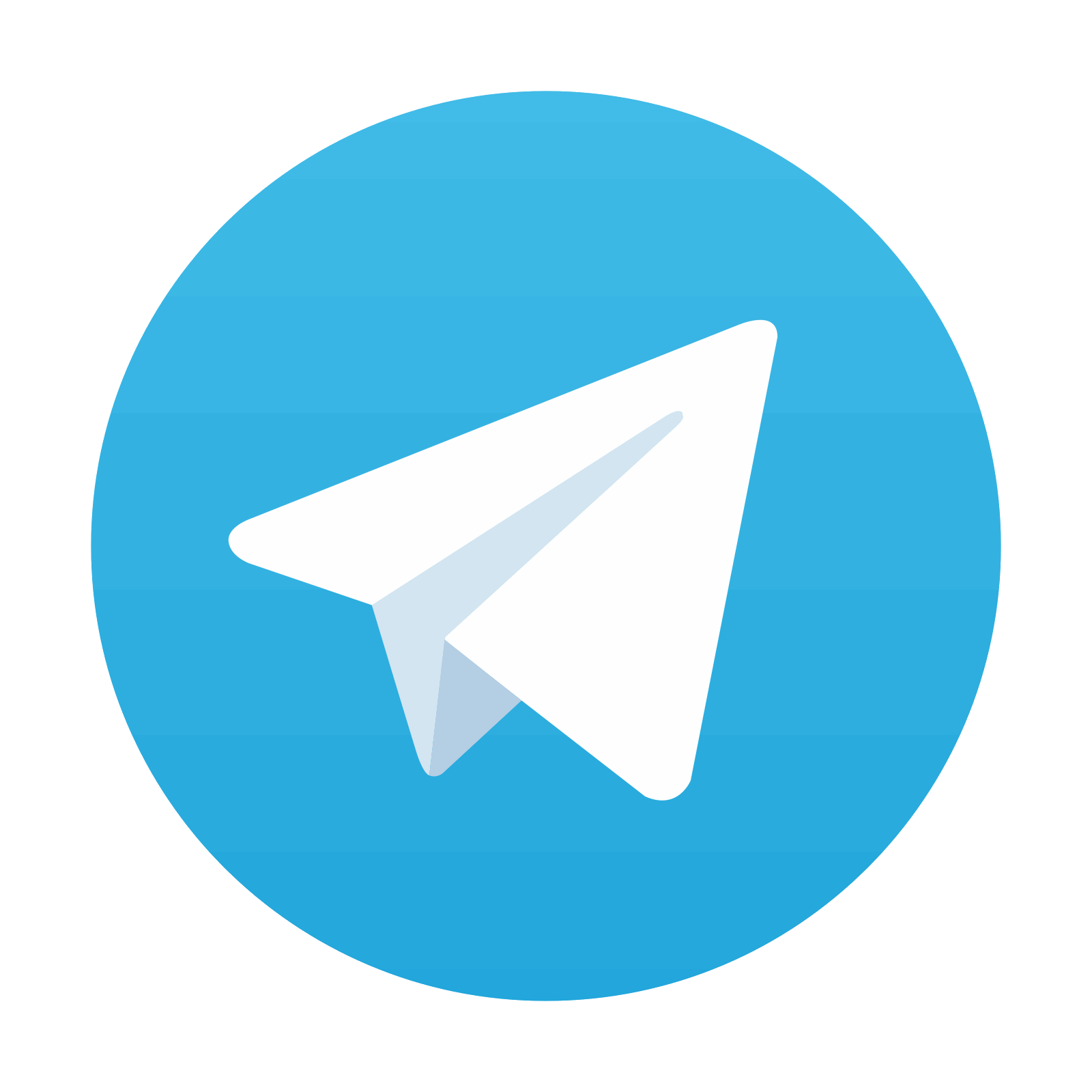
Stay updated, free articles. Join our Telegram channel

Full access? Get Clinical Tree
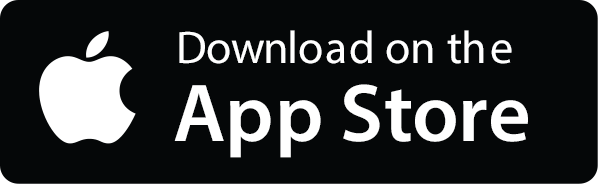
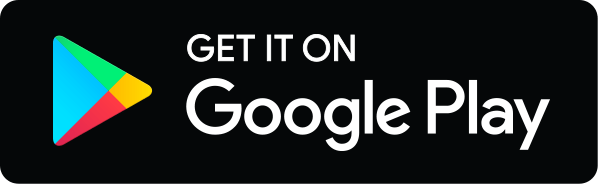