21 Optical Coherence Tomography Diagnosis of Macular Diseases
Optical coherence tomography (OCT) provides new morphologic, qualitative, and quantitative data of all retinal layers that are valuable for diagnosis, development of the best treatment strategy, and monitoring treatment in most macular diseases.
The objective of this chapter is to review the use of OCT as a diagnostic tool in the most common macular diseases, such as vitreoretinal interface disorders of the posterior pole including macular hole, lamellar macular hole and pseudohole, epiretinal membrane (ERM), vitreomacular traction syndrome, and age-related macular degeneration (AMD).
21.1 Vitreoretinal Interface Disorders
21.1.1 Macular Hole
Idiopathic macular hole is a retinal disease characterized by a full-thickness defect of the foveal area with associated visual acuity reduction, metamorphopsia, and a central scotoma. OCT has improved the understanding and management of idiopathic macular hole. Its incidence is around 3 in 1000, and it is more common in women between the sixth and seventh decades of life. 1 The pathophysiology of the development of idiopathic macular hole has been extensively studied. 2 , 3 , 4 In the 1970s, recent posterior vitreous detachment was usually noted in eyes with macular holes, whereas eyes with preexisting vitreous detachment rarely developed a macular hole. This observation led to the conclusion that vitreous traction plays an important role in macular hole formation. 5 In 1983, Avila et al suggested that the main cause of macular hole formation is anteroposterior traction exerted by vitreous fibers on the center of the fovea. 5 In 1988, Gass 2 introduced the concept of tangential traction of the vitreous cortex at the foveolar edges as the cause of macular hole. Immediately, foveal dehiscence was followed by centripetal retraction of retinal elements. 2 The posterior hyaloid face of the vitreous remained attached to the foveola and surrounding macular region at the initial stages of hole formation. 6 In 1995, the same author suggested that most full-thickness macular holes arise from an umbo dehiscence without loss of foveal tissue. 7
Although slit-lamp observations and photographic documentation were provided, adequate imaging of the posterior hyaloid detachment and retinal layers was impossible before the OCT era. With the help of OCT, other investigators have proposed that the hole formation involves vitreoretinal separation with vitreofoveal adhesion exerting oblique traction on the fovea, followed by foveal cysts or foveal detachment, foveal dehiscence, and finally full-thickness hole formation. 2 , 3
With some modification, OCT staging can parallel the staging of macular holes with biomicroscopic examination. OCT has demonstrated changes in the vitreomacular interface that are not visible using biomicroscopy (Fig. 21.1). This imaging method has provided further detailed structure changes and improved our understanding of the mechanism of macular hole formation. It is now widely accepted that the early event leading to macular hole is the persistent adherence of the cortical vitreous to the fovea with adjacent vitreoretinal separation. The resultant traction on the fovea causes foveal detachment or an intraretinal space termed pseudocyst. Further traction leads to dehiscence of foveal tissue, resulting in full-thickness macular hole formation. 8 , 9

Classification of idiopathic macular holes as proposed by Gass 2 , 4 has always been the standard in staging macular holes. However, sometimes determination of the developmental stage of macular holes has been difficult to assess clinically. Since the introduction of the OCT, staging of macular holes has changed, especially with respect to the early stages.
The Gass classification 2 , 4 indicates that stage 1 holes (foveal detachment) can be distinguished by reduced or absent foveal pit and the presence of an optically clear space beneath the fovea suggesting a foveolar detachment. Vitreomacular traction is present as a result of posterior incomplete vitreous detachment lifting the foveal area and the formation of an impending macular hole. Clinically, this appears as a yellowish spot on the fovea. Depending on how soon the patient is examined after the onset of symptoms and the degree of elevation of the retina, either a yellow spot 100 to 200 µm in diameter (stage 1A) or a yellow ring approximately 200 to 350 µm in diameter (stage 1B) is present in the foveolar area. As the foveolar retinal detachment (stage 1A) progresses to foveal retinal detachment (stage 1B), the progressive stretching and thinning of the foveolar center may cause redistribution of the xanthophyll pigment into a ring configuration.
Stage 2 holes are those in the early formation stage. In most patients, the yellow ring enlarges, and a full-thickness retinal dehiscence will develop within several weeks or months. This stage shows a partial break on the surface of the retina, but the operculum is still attached to the retina or separated from the underlying retina with a small full-thickness loss of retinal tissue of less than 400 µm in diameter.
Stage 3 holes have a full-thickness retinal dehiscence with a complete break in the outer retinal tissue and variable amounts of surrounding macular edema that increase retinal thickness and decrease reflectivity in the outer retinal layers. In this stage, separation of the operculum from the underlying retina is complete; hole diameter is greater than 400 µm.
Stage 4 holes are characterized by the complete loss of tissue greater than 400 µm in diameter and a complete posterior vitreous detachment with release of the anteroposterior tractional forces.
Stage 1 and 2 macular holes are difficult to differentiate ophthalmoscopically, and high-resolution OCT images can help to classify them. Stage 2 often progresses to stage 3 with some visual loss; therefore, appropriate staging with OCT can help to determine when surgery is indicated. 10
Vitreoretinal traction is important in the pathogenesis of macular holes, and it can be identified by OCT. 11 Duker et al 12 found vitreoretinal interface abnormalities by OCT in 21% of fellow eyes. Furthermore, OCT may be a useful method of assessing the risk for hole formation in the fellow eye of patients with a unilateral macular hole. Considering that the probability of developing a macular hole in the contralateral eye is 13% in 48 months, 13 it is then mandatory to perform bilateral tomographic imaging in patients affected by this pathology for early detection in the other eye. However, the concept of surgery in the normal contralateral eye of patients with macular holes is not acceptable.
For stage 2-4 macular holes, OCT is effective, and quantitative information may be directly extracted from the OCT tomograms, including the status of the vitreoretinal interface, the diameter of the hole, and the extent of surrounding subretinal fluid accumulation. 11 OCT is useful in differentiating simulating lesions and in allowing better counseling of patients regarding their disorder.
In addition, accurate assessment of macular hole size is important for both research studies and to guide clinical management. The size of a macular hole has been shown to affect anatomical and visual success. Macular hole size and postoperative resolution can be evaluated by OCT. 14 , 15 , 16 , 17 , 18
Altaweel and Ip 14 published the latest staging of idiopathic macular holes based on OCT findings. In stage 1A, OCT demonstrates perifoveal posterior vitreous detachment with continued foveolar adherence. Tractional forces are anteroposterior and tangential. Retinal tissue remains at the base of the pseudocyst. Pseudocyst formation (hyporeflective image on OCT) occurs without affecting all retinal layers and with an intact outer retina. In stage 1B, the foveal pseudocyst affects all retinal layers, including the photoreceptor layer with an intact roof. There is incomplete posterior vitreous detachment with persistent adhesion onto the fovea in stages 1A and 1B (Fig. 21.2). In stage 2A, the roof of the pseudocyst has been torn open and continues to have traction exerted by the vitreous attachment. There is persistent traction of the posterior hyaloid that is firmly attached to the inner retina. A break in the roof of the pseudocyst gives rise to a full-thickness macular hole. In stage 2B, the roof of the pseudocyst has completely separated from the retina. At this point, there is release of the anteroposterior tractional forces. The distance between the edges of the hole is less than 400 µm. In stage 3, the prefoveal operculum can still be appreciated, but the distance between the hole edges is greater than 400 µm. The posterior hyaloid is completely detached from the inner retina, as opposed to the description of Gass, 2 wherein the former remains attached to the perifoveal area. In stage 4, vitreous detachment is complete which cannot be seen tomographically and can be confirmed only by slit-lamp biomicroscopy or ultrasonography (Fig. 21.3 , Fig. 21.4 , Fig. 21.5 , Fig. 21.6).





The main differences between the biomicroscopic and the OCT staging of idiopathic macular holes are the presence of a tight focal foveolar adherence of the posterior hyaloid versus a perifoveal vitreomacular detachment, the formation of a foveal pseudocyst versus a detachment in Gass’ stage 1 hole, and the subdivision of stage 2 into two distinct anatomical types.
In cases with a base diameter less than 400 µm as measured by OCT, an anatomical closure was achieved in 92%, and in those with a base diameter greater than 400 µm, this value decreased to 56%. Several variables—like preoperative visual acuity, symptoms’ duration, hole diameter by OCT (as a single variable), and stereoscopic funduscopy—may predict the final visual outcome. 15 , 16 One study demonstrated that OCT measurement is predictive not only of the possibility for anatomical closure but also of postoperative visual acuity outcome. By using the maximum hole diameter, minimum hole diameter, and height as reference values, the authors use a formula to infer macular hole prognosis. 16
Likewise, OCT is useful in evaluating anatomical closure after macular hole surgery (Fig. 21.7). The flattening of the retina and the disappearance of the retinal cysts may be appreciated postoperatively. Closure of the horizontal component of a hole wherein there is complete detachment usually takes place in the first postoperative days. Absence of closure within the first postoperative month entails poor prognosis. 7 In the normal course of an idiopathic macular hole, in about 2% of cases, the macular hole may close by itself spontaneously when there is release of vitreomacular traction. In such cases, the photoreceptors may be affected as a result of the previously existing traction and, as a consequence, may give rise to an absolute central scotoma.

In conclusion, OCT imaging is a highly important tool in the diagnosis, in evaluation of the etiopathogenesis, for postoperative follow-up, and as a predictive factor in the prognosis of idiopathic macular holes.
21.1.2 Non–Full-Thickness Macular Holes
The description and pathogenesis of non–full-thickness macular holes have been better understood with the advent of OCT. Lamellar macular hole (LMH) and macular pseudohole (MPH) can be different clinical manifestations of the same disease.
Lamellar macular hole was first described by Gass 17 in 1975 as an abortive process of full-thickness macular hole formation resulting from deroofing of cystoid macular edema. Instead, MPH was described as being caused by centripetal contraction of previously present ERM, which surrounds but does not cover the foveal area. 18 However, coexistence and possible evolution from a MPH to lamellar macular hole after progressive ERM contraction are possible. 19
Since the development of OCT, interest in diagnosis of LMHs has been renewed because high-resolution images can detect a lamellar macular defect that is not always visible clinically. 20 , 21 Data based on OCT proved that only 28% to 37% of LMHs are correctly diagnosed by biomicroscopy. 21 , 22 OCT enables clear differentiation between macular pseudoholes (MPHs) and LMHs. 21
In OCT, LMHs have been described as non–full-thickness defects of the macula having an irregular foveal contour with a dehiscence in the inner fovea, separation of the inner from the outer retinal layers, and absence of a full-thickness foveal defect with intact outer retinal layers at the base of the hole 22 (Fig. 21.8, Fig. 21.9).


In OCT, MPH has been described as having a steep fovea contour and a normal or slightly increased central and paracentral retinal thickness 22 (Fig. 21.10 , Fig. 21.11). Thus, before the OCT era, many cases might have been misdiagnosed. In full-thickness macular holes, the percentage of coexisting ERMs confirmed with SDOCT is about 13%. 23 Different authors have reported on ERMs coexisting with LMH in 62 to 73% of cases using OCT. 24


Witkin et al 21 reported that with ultrahigh-resolution optical coherence tomography, ERMs are visible in 89% of LMH cases. Other observations demonstrate the presence of a hyperreflective linear structure in all the cases of LMH. 25 LMH is generally regarded as a clinically stable disorder. Theodossiadis et al 26 studied 41 patients with LMH and reported a mean decline of 6.4% in visual acuity after 37.1 months of observation. Indications for surgical treatment of LMH remain controversial. Only a limited number of reports on vitrectomy results in LMH patients have been published, and most studies reported favorable outcomes. 19 , 27 , 28 , 29 Recent reports on vitrectomy with removal of the ERM-ILM with or without gas injection demonstrated benefit both functionally and morphologically. 30 , 31
Bottoni et al 32 support previous indications 26 in planning treatment strategies; thus, vitrectomy could be indicated in LMHs showing a progressive thinning of foveal thickness and/or visual acuity deterioration during the follow-up of the natural course of the disease.
Recently, Lee et al 33 indicated that poor initial visual acuity, the presence of a disrupted inner segment/outer segment (IS/OS) junction (ellipsoid zone), or a thin fovea on preoperative spectral-domain (SD) OCT predicted poor vision outcome after LMH surgery. However, whether it is worthwhile to attempt surgical treatment for LMH remains a controversial issue.
21.1.3 Epiretinal Membranes
Epiretinal membrane is a disorder in which the vitreomacular interface induces a tangential tractional force on the retina, which can result in distortion of the macular architecture and development of vascular leakage with associated macular edema and vision loss. Visual symptoms range from mild to severe and usually are manifested by blurred vision or metamorphopsia.
Epiretinal membranes occur in 2.2% to 18.5% 34 , 35 , 36 , 37 of the population and can be either primary idiopathic, in which the incidence among elderly patients increases with age, or secondary to intraocular inflammatory conditions, retinal vascular disease, or surgical intervention. ERMs may also appear in otherwise healthy eyes as a result of incomplete vitreous detachment and mostly is characterized by the anatomical location of the ERM between the internal limiting membrane (ILM) and the vitreous body interface. 38
Epiretinal membranes are caused by the migration of cells through small focal defects in the internal limiting membrane after posterior vitreous detachment or by retinal breaks and detachments. These cells proliferate and create a thin veil of tissue at the retinal-vitreous interface. Purely glial cells are reported to occur in the earlier form of ERM, whereas a prominent fibrous, nonglial component has been reported in membranes, causing traction.
Gass 39 proposed grading the severity of ERMs on the following clinical scale: grade 0, translucent membranes unassociated with retinal distortion; grade 1, membranes causing irregular wrinkling of the inner retina; and grade 2, opaque membranes causing obscuration of the underlying vessels and marked full-thickness retinal distortion.
The early form of ERM, called cellophane macular reflex, is usually asymptomatic; therefore, most patients have normal or nearly normal vision with occasional metamorphopsia. However, the more severe form, known as macular pucker, can cause significant loss of visual acuity and visual symptoms such as distortion and metamorphopsia. 37 In severe cases, macular edema and retinal detachment have been known to occur.
Mori et al 40 and Wilkins et al 41 classified ERM based on a scale secondary to OCT findings. OCT images of ERMs may be classified into two broad categories: globally adherent membranes or partially nonadherent membranes. Both types of ERMs are usually visible on OCT images as a taut hyperreflective line contiguous with or anterior to the inner retinal surface. The secondary effects of the membrane, such as loss of the normal foveal contour, variable irregularity of the inner retinal layers, and macular thickening, are used to establish the presence of the membrane.
In addition, OCT provides a means to evaluate the cross-sectional characteristics of ERM, allowing a quantitative measurement of retinal thickness, membrane thickness, and the separation between the membrane and inner retina. Quantitative measurements of membrane thickness and reflectivity can be used to establish the degree of membrane opacity. The OCT tomogram can help to distinguish between membranes globally adherent to the retina and ERMs separated from the inner retina. 41
The appearance of such an ERM might be mimicked by a partially detached posterior vitreous surface. However, ERMs tend to be thicker and more reflective than the posterior vitreous. The reflection from an ERM may measure up to 60 µm thick; this is rarely observed with a partially detached posterior vitreous. Complete posterior vitreous detachment (PVD) is visible on the OCT image as a linear reflectivity suspended above the retinal surface (Fig. 21.12). On OCT, ERMs are visible as a highly reflective layer on the inner retinal surface (Fig. 21.13 , Fig. 21.14).



Characterization of the ERM with OCT may help in preoperative planning for membrane peeling. 36 In cases with separation between the membrane and retina, the surgeon may be directed to these areas to initiate membrane dissection. When the membrane is globally attached to the inner retina, the surgeon may anticipate more difficulty in peeling the membrane (Fig. 21.15 , Fig. 21.16 , Fig. 21.17). The surgeon may also proceed with particular caution when extensive intraretinal edema leaves a thin, friable inner retinal layer beneath the membrane. In most ophthalmology centers, macular surgery for ERM removal is advocated if the best-corrected visual acuity falls below approximately 20/40, but there are no reports of objective indications for surgery, such as OCT findings.



Metamorphopsia induced by ERM may be related to the edematous areas of the inner nuclear layer (INL) detected with SD OCT. The classification of ERM based on INL thickness is a potentially useful indication for surgery. 35
High-resolution OCT has several advantages for a detailed evaluation of vitreomacular traction (VMT) and ERM disease: raster scans provide a precise two- and three-dimensional (3-D) image of the vitreomacular interface at each location. The high-resolution representation of all intraretinal layers identifies the level and location of alterations in functionally relevant structures, such as the neurosensory architecture and the photoreceptor layer. 34
Several factors, such as preoperative visual acuity, 42 duration of symptoms before surgery, 43 and the presence or absence of cystoid macular edema, 44 have been suggested as prognostic factors influencing the postoperative visual acuity. Investigators have suggested that the macular microstructure, such as macular thickness and appearance of the photoreceptor layer, may be associated with postoperative visual acuity. 45 , 46 The introduction of SD OCT has improved the speed and sensitivity of the examination, allowing scanning at a higher resolution. Therefore, postoperative OCT imaging can be used to document surgical response. Usually, retinal thickness decreases after successful ERM peeling, visual acuity improves, and the distorted vascular pattern returns to its normal morphology.
The photoreceptor layer can be imaged with SD OCT as a hyperreflective line showing the IS/OS junction above the retinal pigment epithelium. An intact IS/OS junction can be defined as a continuous hyperreflective line, and the diagnosis of a disrupted IS/OS is made based on loss or irregularity of the hyperreflective line corresponding to the IS/OS junction. Existence of a correlation between photoreceptor IS/OS junction disruption and decreased postoperative visual acuity has been reported using time-domain (TD) OCT. 45 , 46 Macular edema resulting from the formation of ERM and some other artifacts may prevent a clear delineation of the IS/OS junction in TD OCT. However, SD OCT allows more precise visualization of the intraretinal morphologic features, such as the external limiting membrane and the photoreceptor layer, thereby enabling clear measurements of the IS/OS junction, even in the presence of a thickened retina caused by the presence of an ERM. 47 , 48
21.1.4 Vitreomacular Traction Syndrome
As the vitreous liquefies with age, it detaches from the macula; this natural progression has been demonstrated using OCT in normal eyes. 49 However, in some people, an abnormally strong adhesion is present between the vitreous cortex and the macula, and as the vitreous detaches peripherally, it continues to pull on focal areas of the macula. This configuration appears identical to the vitreous attachment identified in idiopathic macular hole and cystoid macular edema or submacular fluid. Macular thickening caused by continued pathologic adherence of the vitreous to the retina in the setting of a peripheral vitreous detachment has been termed vitreomacular traction syndrome (VMTS). 50
First described in 1865 by Iwanoff, 51 VMTS refers to conditions in which retinal changes develop from incomplete posterior vitreous detachment with persistent vitreous adhesion to the macula leading to morphologic distortion of the retinal surface as a result of the proliferation of myofibroblasts and the contractile element of the ERM, 52 followed by functional changes such as metamorphopsia and visual deterioration. In a few of these eyes, the vitreous eventually separates from the macula on its own, leading to spontaneous resolution of vitreoretinal traction with normalization of the retinal contour and restoration of visual acuity. These changes have been documented with OCT. 53 However, in some eyes, vitreomacular traction persists and can lead to progressive retinal edema, metamorphopsia, and/or visual deterioration. In these cases, vitrectomy may be an effective treatment option for patients with persistent and symptomatic vitreomacular traction diagnosed biomicroscopically. 54 Although the vitreous attachment to the macula usually appears broad on clinical examination, OCT typically shows an incomplete v-shaped posterior vitreous detachment temporally and nasally to the fovea but with the fovea remaining attached (Fig. 21.18). Early stages of PVD not detected biomicroscopically have been shown using OCT in normal subjects. 49 Therefore, in all cases with vitreoretinal interface abnormalities, OCT is extremely useful for diagnosis and follow-up after treatment of these diseases. OCT images of the interface between the macula and vitreous are very well defined because of the difference in reflectivity of the relatively acellular vitreous and parallel-fiber orientation of the inner retina. 9 PVD is visible on the OCT image as a thin, moderately reflective band suspended above the macular surface when it separates from the retina (Fig. 21.19). 55


Vitreomacular traction syndrome differs from idiopathic ERM in that the posterior hyaloid, rather than being totally detached from the posterior retina surface, remains attached to the perifoveal region. It is also frequently attached at the optic nerve or at multiple other points along or inside the vascular arcades. Sometimes the vitreous adherence can be difficult or impossible to identify directly on clinical examination (Fig. 21.20). In VMTS, the PVD frequently is less reflective than ERMs (Fig. 21.21) and is associated with substantial foveal traction, intraretinal cystoid changes, cystoid macular edema, and foveal detachment. These changes result in central vision loss and metamorphopsia.


Other VMTS cases can have a PVD temporally to the fovea but no posterior vitreous detachment nasal to it. In these cases, prominent cystoid macular edema may develop, which may result in a macular hole or macular atrophy. 56 On the other hand, persistent traction can lead to progressive retinal edema and thickening. Quantifying such changes with OCT can be valuable in determining the need and timing of surgical intervention. As with ERMs, OCT can provide useful information in counseling VMTS patients preoperatively with regard to visual potential. Eyes demonstrating massive traction, distortion of retinal architecture, intraretinal edema, and foveal detachment may be anticipated to have a relatively poor ultimate outcome compared with eyes not exhibiting these features. After surgery, OCT can be used to evaluate the anatomic response (Fig. 21.22). Case reports in the literature have documented improved retinal anatomy in association with increased visual acuity after vitrectomy surgery. 49 , 57

High-resolution OCT has several advantages for a detailed evaluation of VMTS: raster scans provide a precise 2- and 3-D image of the vitreomacular interface at each location. The high-resolution representation of all intraretinal layers identifies the level and location of alteration in functionally relevant structures, such as the neurosensory architecture and the photoreceptor layer. 34 , 58 Other advantages of SD OCT are improved axial resolution, higher scan speed, and the possibility of scanning the entire macular area at each location using the raster mode. These improvements enable better reproducibility of the same area of interest at each visit and therefore enable superior results in a study aimed at evaluating distinct morphologic changes over time.
Recently, Sayegh et al 58 published a prospective analysis of the functional and morphologic parameters of retinal integrity over a 2-year follow-up of 30 patients undergoing vitreoretinal surgery for VMTS guided by high-resolution SD OCT imaging. They observed that the best-corrected visual acuity did not correlate significantly with central retinal thickness (CRT) or retinal volume (RV); thus, a specific reduction of CRT and RV has no significant effect on visual function. This result is consistent with the study by Haouchine et al, 22 who measured central foveal thickness and reported no correlation with best-corrected visual acuity (BCVA). Furthermore, no correlation was found between the shape of the foveal contour, the presence of foveal cysts, or other morphologic parameters with BCVA.
The presence of the ERM induces folding and contraction of the retinal surface and surgical release, including the internal limiting membrane, results in a rapid and impressive restoration of the retinal surface with disappearance of folds and reformation of the foveal contour. These morphologic changes seem to precede functional recovery. Visual rehabilitation proceeds over several months, reaching significance as late as 6 months after surgery. The integrity of the inner and, most importantly, the outer retinal layer follows a prolonged pattern of recovery as well. Recovery of inner and outer retinal layer proceeds slowly, reaching significance at 12 months, and correlated strongly with visual function. Therefore, reconstitution of neurosensory layers may be the most relevant parameter for visual improvement.
Vitreomacular Traction and Age-Related Macular Degeneration
Vitreomacular traction alone is perhaps not able to induce AMD. However, it would seem sensible to consider vitreous changes when diagnosing and treating AMD patients because of the high coincidence of VMT and choroidal neovascularization (CNV) and the often successful treatment of other diseases of the vitreoretinal interface by vitrectomy. The concept of the pathogenesis of AMD should therefore be extended to include the influence of the vitreous, especially where therapeutic concepts such as pharmacologic vitreolysis and vitreous separation have been established as causative treatment of late forms of AMD. 59
Krebs et al described the presence of vitreomacular adhesions in 36% of eyes with exudative AMD, significantly more frequently than in eyes with nonexudative AMD or control eyes (Fig. 21.23). 60 These results were confirmed by Robison et al, who found vitreomacular adhesion (VMA) in 38% of eyes with exudative AMD 61 and by Lee et al, 62 who found VMA in 22.3% associated with neovascular AMD. These studies used primarily Stratus OCT examinations to verify VMA. Owing to the TD technology of Stratus OCT, differentiation between adhesion and traction was difficult, although traction was suspected in more than 50% of the cases. Signs of traction could clearly be identified in both SD OCT machines, the Spectralis and Cirrus OCT. Especially in the high-definition scans (five lines 6 mm long each in the Cirrus OCT and one single line 8 mm long each in the Spectralis OCT) the angulation of the posterior vitreous cortex was clearly visible. Furthermore, 3-D OCT shows splitting within the posterior vitreous cortex in the area of vitreopapillary adhesion and traction from different directions. 3-D OCT imaging was possible in most (83.3%) of the Cirrus OCT cube scans, but with Spectralis OCT volume scans, only 13.3% was sufficient scan density possible.

Krebs et al 63 identified traction with adhesions in 73.3% of cases with exudative AMD, and they showed that the location and the direction of the traction forces visualized by high-technology OCT and 3D-OCT correspond in 100% with the origin of the CNV.
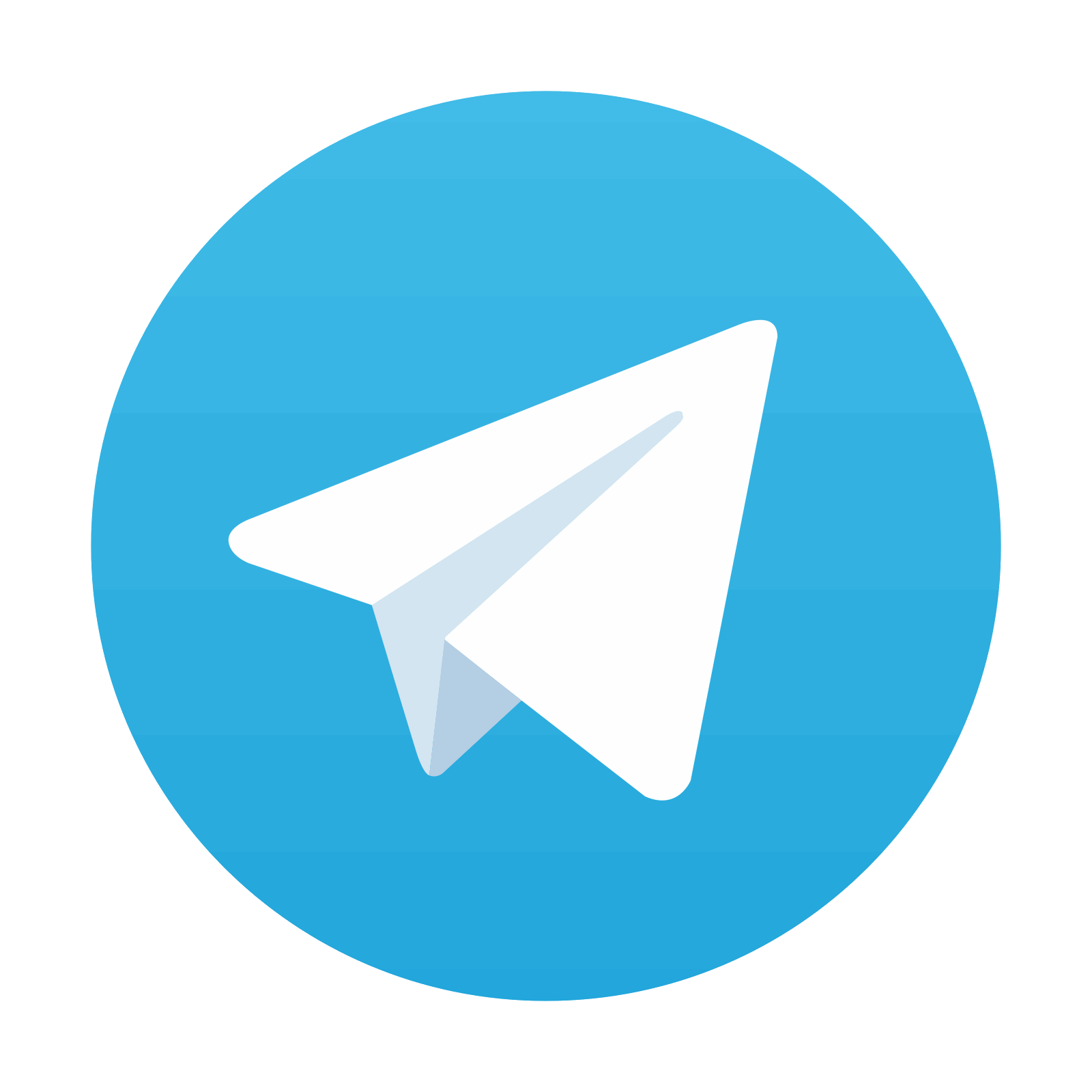
Stay updated, free articles. Join our Telegram channel

Full access? Get Clinical Tree
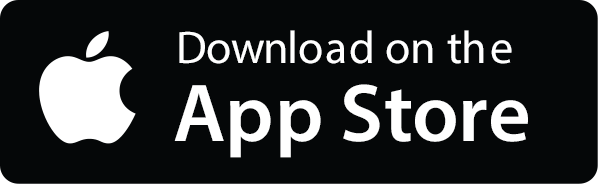
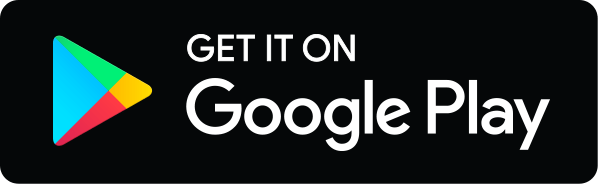
