Summary
Vascular injury is one of the most feared complications in skull base surgery due to its potential to result in significant morbidity. Immediate feedback concerning cerebral perfusion is crucial for optimized management. This real-time feedback can be provided using multimodality intraoperative neurophysiologic monitoring (IONM). In this chapter, we will review the principles, technical aspects, interpretation, and limitations of each of the different IONM electrophysiological modalities that might be helpful in this context.
Key words
Neurophysiology – intraoperative neuromonitoring – skull base surgery – vascular injury – cerebral blood flow – ischemia21 Neurophysiologic Monitoring and Its Role During Cerebrovascular Injury
21.1 Key Learning Points
Somatosensory evoked potentials (SSEPs) and electroencephalography (EEG) recordings can be obtained continuously in real time during surgery.
SSEP and EEG are sensitive to changes in cerebral perfusion during surgery.
Significant changes in SSEPs, an increase in the latency and decrease in amplitude of the cortical responses, can be utilized as a criterion to warn the surgical team of impending neurological injury.
Significant decrease in the amplitude of the background EEG can serve as an indicator for cerebral perfusion and warn the surgical team.
SSEP and EEG can serve as a neurophysiological biomarker of cerebral perfusion and postoperative neurological deficits.
Motor evoked potential (MEP) and brainstem auditory evoked potential (BAEP) are especially useful in subcortical hypoperfusion related to perforator injury.
21.2 Introduction
Skull base surgery faces unique challenges including intimate relationships between tumors and cerebrovascular structures. Resection of tumors located along the path of the internal carotid artery (ICA) carries the risk of injuring the vessel and will be a significant concern. Another example of major arterial risk during skull base tumor resection is the basilar artery. Lesions involving the clivus and foramen magnum may also place the basilar artery, its tributaries, or its branches, at risk. In a similar way, lesions involving the anterior skull base can be intimately related to the anterior communicating artery complex vessels, including the anterior cerebral arteries, and frontopolar and fronto-orbital arteries. 1
Vascular injury is one of the most feared complications due to its potential for significant morbidity. 2 , 3 , 4 Intraoperative neurophysiologic monitoring (IONM) with somatosensory evoked potentials (SSEPs), electroencephalography (EEG), and motor evoked potentials (MEPs) can detect significant cortical and subcortical cerebral hypoperfusion leading to potential corrective action. Real-time information about the functional status of the integrity of the nervous systems secondary to perfusion plays an essential role in the management of vascular complications guiding immediate corrective action before the onset of permanent neurological deficit. 2 , 5 , 6 , 7 , 8 , 9
In addition to direct vascular injury, which can usually be visually identified by the surgical team, other mechanisms can contribute to vascular complications such as cerebral hypoperfusion related to hypotension or hemodilution, and embolic phenomenon associated with vessel manipulation. 7 , 10 In these cases, an ongoing injury can be overlooked unless continuous functional assessment is in progress.
21.3 Cerebral Perfusion Principles and Neurophysiologic Tools for Measurement
Normal cerebral blood flow (CBF) in awake adults is approximately 50 mL/100 g/minute, with cortical regions requiring higher levels compared with subcortical regions. Animal studies indicate that a decrease in CBF to 16 to 20 mL/100 g/minute results in reversible cellular dysfunction (ischemia) and a significant decrease in neurophysiological response amplitude. CBF below this level risks “electric failure” (functional threshold) and a loss of neurophysiological responses is an immediate precursor of “ion pump failure” and cellular death. The flow threshold for ion pump failure or infarction occurs at 10 to 12 mL/100 g/minute (lesion threshold). This means that there is a time window, as CBF decreases, wherein restoration of flow will avoid permanent injury. 6 , 7 , 8
There is wide variability in the individual susceptibility to hemodynamic changes related to drop in systemic blood pressure, embolic events, direct vascular injury, or arterial cross-clamping. This susceptibility depends on self-regulatory mechanisms, including, but not limited to, the status of the circle of Willis, other collateral circulation, the presence of atherosclerosis, and autoregulation. Thus, during a vascular complication it is often necessary to determine intraoperatively if the hemodynamic situation during the complication is compatible with sufficient cerebral perfusion in each individual. 2 , 11
Numerous tools have been proposed that allow for intraoperative assessment of CBF such as Doppler, flow probes, which use ultrasonic measurements, stump pressure, EEG, and SSEPs, among others. 8 , 11 , 12 The distinct advantage of neurophysiological methods is the ability to evaluate the consequence of hemodynamic changes while Doppler, flow, and pressure provide local data without information on how it impacts the individual patient. 8 , 11 Moreover, EEG and SSEP are noninvasive and technically easy to implement and record in the operating room (OR).
The SSEP evaluates the entire somatosensory neural axis which includes the peripheral nerve, spinal cord dorsal columns, the brainstem medial lemniscus pathways to the contralateral thalamus, and connections to the primary somatosensory cortex. These structures are represented by a sequence of scalp recorded SSEP components named according to the polarity (positive [P] or negative [N]) and peak latency. Thus, for the upper limb SSEPs, the N9, N13, and N20/P22 components of the SSEP are generated at the brachial plexus, cervicomedullary junction, and anterior parietal somatosensory cortex, respectively, while for lower limb SSEPs, the equivalent SSEP components are the N20, N30, and N37/P45 components, respectively. 7 , 13
Adequate CBF is necessary for the generation of SSEP responses by viable neurons. As stated earlier, decreases in CBF below 16 to 20 mL/100 g/minute causes a reversible decrease in the amplitude of cortical SSEP responses due to desynchronization of cortical neurons and a reduction in the number of functional neurons. 8 Cortical SSEP responses disappear with corresponding CBF values between 12 and 15 mL/100 g/minute as a result of the “electrical failure.” Considering that the flow threshold for ion pump failure or infarction occurs at 10 to 12 mL/100 g/minute, these changes in SSEPs can be a precursor of ion pump failure. 6 , 7 , 8 , 14 , 15 , 16
The EEG is a graphical representation of global neuronal electrical activity within the entire cerebral cortex. Physiologically reversible EEG changes such as a decrease in amplitude and frequency alterations also occur with decrease in CBF. For example, decrease in α (8–13 Hz)/β (≥14 Hz) frequencies and increase in θ (5–7 Hz)/δ (0.5–4 Hz) frequencies occur when CBF decreases to ≤22 mL/100 g/minute, while isoelectric (i.e., flat) EEG (absence of cerebral activity of over 2 microvolts [μV]) can be observed following more significant reductions in CBF (7–15 mL/100 mg/minute). 8 , 11 , 17 To facilitate interpretation and comparison of EEG activity across different time-points during surgery, processed spectral EEG activity can also be used, which use fast Fourier transformation of the raw EEG data. 12
Other neurophysiologic modalities that may be useful during vascular procedures that pose a risk for vessel injury include brainstem auditory evoked potential (BAEP) and transcranial motor evoked potentials (TcMEPs). BAEPs are generated in response to independent auditory stimulation delivered to the ear and consist of a sequence of deflections called Jewitt Waves I, II, III, IV, and V which originate from the distal cochlear nerve, cochlear nucleus, superior olivary complex, lateral lemniscus (LL), and inferior colliculus (IC), respectively. Thus, the BAEP can assess the integrity of the cochlear nerve and the auditory structures of the brainstem from the pontomedullary junction to the lower midbrain. 6 , 13 , 18 Primate studies on the effect of focal brainstem ischemia on BAEPs demonstrate that ischemia increases the latency and reduces the amplitude of BAEP waveforms. The gradient of sensitivity to ischemia where the latency of BAEP responses from the LL was increased at CBF was 12 to 15 mL/100 g/minute, and the CBF was above 20 mL/100 g/minute for inferior colliculus. 6
TcMEPs assess the integrity of the descending corticospinal tract (CST) from the primary cortex of the frontal, precentral cortex to the skeletal muscles, traversing through the corona radiata, the posterior limb of the internal capsule, the medullary pyramid, the anterior and lateral tracts of the spinal cord, the alpha motor neuron, and the neuromuscular junction. The subcortical CST pathway can be selectively affected by perforating artery injuries which may be undetected as they can occur while other neurophysiologic modalities such as SSEPs and the EEG remain unchanged. 2 , 22 For example, during clipping of a cerebral aneurysm in the anterior and middle cerebral arteries, inadvertent occlusion of the perforating arteries (e.g., anterior choroidal artery) can lead to change in TcMEPs without significant change in SSEPs and EMPs.
Electromyography (EMG) is another neurophysiological modality which is often used during skull base surgeries for monitoring the motor branches of certain cranial nerves. However, EMG monitoring has no direct role in the management of vascular injury during skull base procedures. For this reason, cranial nerve EMG monitoring is not discussed in this chapter.
Each IONM modality has its strengths and limitations; thus, multimodality monitoring is essential in identifying ischemia during skull base procedures. The selection of IONM modalities should be guided considering the risks of the surgical procedure for each patient and the location of the pathology (▶ Table 21.1).
21.4 IONM Modalities: Technical Considerations
21.4.1 SSEP
SSEPs are recorded in response to stimulation of the median or ulnar nerves at the wrist and the tibial or peroneal nerves at the ankle or fibular head, respectively, using surface or needle electrode pairs placed bilaterally. Subdermal recording electrodes are placed on the scalp at multiple locations, including P3 or CP3, P4 or CP4, Pz, and Fz, according to the international 10–20 system. 20 Cortical SSEP waveforms are recoded using P3–CP3/Fz and P4–CP4/Fz montages for upper extremity stimulation and at Pz/Fz or P4–CP4/P3–CP3 montages for lower extremity stimulation. Subcortical responses are recorded from electrodes placed on the skin surface over C2–C7 spinous process and referenced to scalp electrode Fz. In addition, brachial plexus generated potentials are recorded from electrodes placed over the Erb’s point bilaterally and referenced to one another. Interleaved upper and lower extremity stimulation is performed at a rate of 2 to 4 Hz with a pulse duration of 0.2 to 0.3 milliseconds. Typical stimulation intensity ranges from 20 to 40 mA for the upper limbs and from 30 to 60 mA for the lower limbs. 6 , 7 , 13 , 15
The sensitivity range to display the waveform varies according to the amplitude of the obtained waveforms, which is usually 0.3 to 1 μV. The time base is set at 10 msec/div for upper and lower limbs. Band-pass filters are set from 10 to 300 Hz for cortical recordings and from 30 to 1000 Hz for subcortical and Erb’s point recordings. Technical difficulties can be encountered in the collection, display, and analysis of the SSEP waveforms. One problem in stimulation and collection of data is to make sure the electrodes necessary for the process are plugged in appropriate slots in the IONM equipment. Labeling the electrodes can help identify them during the procedure when there is reduced access. Significant noise can be encountered during the collection of the data and averaging SSEPs at either 128 or 256 stimulated trials, depending on the signal quality, 6 , 7 can alleviate the issue.
21.4.2 EEG
According to the 10–20 international system, 8 to 16 subdermal electrodes are placed on the scalp, and a longitudinal bipolar montage can be assembled to cover the anterior and posterior aspects of each hemisphere adequately. The sensitivity of EEG is partly related to the number of EEG channels and the inter-electrode distances for each channel. Electrode impedance should be below 5 kΩ. The bandpass filter is from 1 Hz to at least 30 Hz, but preferably 70 Hz. A 60 Hz notch filter is generally necessary. Time base is set at 30 mm/second or slower, 15–to 10 mm/second and the sensitivity at 2 to 3 μV/div to better appreciate asymmetries. 5 , 12 , 13 Common technical challenges in the collection of the EEG is the placement of electrodes in the head. Due to the intrusion of the surgical field, the placement location can be compromised resulting in two electrodes being too close to each other. Since most of the EEG collection is based on a potential difference between two electrodes, identifying a reference electrode significantly far from the primary electrode will help address the concern.
21.4.3 BAEP
Auditory stimulation is delivered using a click in an alternating fashion using expanding foam earbuds placed in the external auditory canal at 80 to 100 dBpeSPL, at a rate between 10 and 40 Hz, while white noise is applied to the contralateral ear at about 65 dBpeSPL. About 512 to 1,024 responses are averaged for each BAEP epoch depending on the signal quality and signal-to-noise ratio. Recording channels on the scalp include Cz-A1, Cz-A2, A1-A2, and Cz- Cv2, placed as per the international 10–20 system. Amplifier bandpass is 100 to 1000 Hz for all channels. 6 , 13 Technical concerns secondary to fluid drainage from the surgical site during surgery can reduce the auditory stimulation. Placing a soft insert in the ear and sealing it will be helpful during the procedure to reduce these conduction problems.
21.4.4 TcMEP
TcMEPs are elicited in response to transcranial electric stimulation using a high-frequency train technique: multipulse stimulation (three to nine stimuli) delivered with a short interstimulus interval (ISI) (2–4 msec), duration of 0.05 to 0.5 msec and an inter-train frequency of 1 to 2 Hz. Subdermal stimulating electrodes are placed at C3, C4, C1, C2, Cz, and 6 cm anterior to Cz allowing multiple montages: C1/C4, C2/C3, C3/C4, C3–C4/Cz and Cz, Cz/Cz + 6 cm. The intensity of stimulus usually ranges from 40 to 200 mA. Responses are recorded from muscles in the form of a compound muscle action potential from all four limbs. Muscle recordings are typically from abductor pollicis brevis and abductor hallucis which have optimal cortical representation. Additional muscle groups can be utilized depending on the procedure. Recording parameters include sweep length of 100 msec, bandpass filter from 100 to 2,000 Hz, and a sensitivity of 15 to 500 μV/div according to the amplitude of the obtained responses. 13 , 21 , 22 , 23 In comparison to SSEPs, BAEP, and EEG, which are usually continually monitored throughout the procedure, TcMEPs are usually acquired at certain periodic intervals due to patient movement induced by excessive muscle contraction, and thus they are monitored intermittently. Tongue laceration and seizures have noted to be risks of TcMEPs during a surgical procedure. Placing a soft bite block in between teeth after intubation, and screening patients for preoperative seizures can reduce the risk. Care should be taken to communicate to the anesthesia team about the need for total intravenous anesthesia and use of neuromuscular blocking agents for intubation. This will help the neurophysiology team obtain baseline data before major surgical manipulation and use it as a reference to compare with data collected during surgery.
21.5 Anesthetic Considerations
Effects of anesthesia on IONM modalities are well documented in the literature. 13 , 16 , 24 , 25 , 26 Theoretically, any drug has some potential to compromise IONM while IONM may be realized under any anesthetic regimen. It depends on the dose used, the stability of the infusion, and the neurophysiologic modalities being used. Therefore, proper interaction between the anesthesiology and neurophysiology teams is crucial.
In general, cortical, polysynaptically generated responses are more affected than subcortical or peripheral responses. Anesthetic changes typically result in a symmetric decrease in the amplitude as well as a prolongation of latency of responses. It is possible, however, for previously damaged pathways to display more pronounced paradoxical, unilateral changes which can be misleading during interpretation of systemic effects of various anesthetic agents.
MEPs are particularly adversely affected by volatile anesthetic agents, while BAEP are least susceptible and SSEPs have intermediate vulnerability. TcMEPs are readily abolished with the use of pharmacological paralytic agents, inhalational agents, barbiturates, and benzodiazepines. Thus, total intravenous anesthesia (TIVA) with steady infusion rates of opioid and propofol are recommended when multimodality monitoring will be used as the TIVA technique has the least deleterious effect on MEP and SSEP recordings. Conversely, some drugs can actually enhance the evoked potential responses. These include ketamine, etomidate, or even neuromuscular blocking agents which can improve EEG, SSEP, and BAEP responses by optimizing the signal-to-noise rate due to the reduction of muscle activity. 26 , 27
Regarding EEG, at lower anesthetic levels, fast frequencies are common with induction agents, followed by a dose-dependent reduction in frequency and amplitude. The anesthetic-induced fast frequencies are the first to be eliminated during ischemia, which may aid in identification of perfusion asymmetries. Further increase in anesthetic concentrations results in a burst suppressed pattern of EEG and even complete cessation of all EEG activities which precludes accurate analysis of cerebral perfusion.
Of course, not all anesthetic agents follow these general principles. However, details about the effect of specific agents are beyond the scope of this chapter. For further information, targeted literature is recommended. 12 , 24 , 25 , 26 , 27
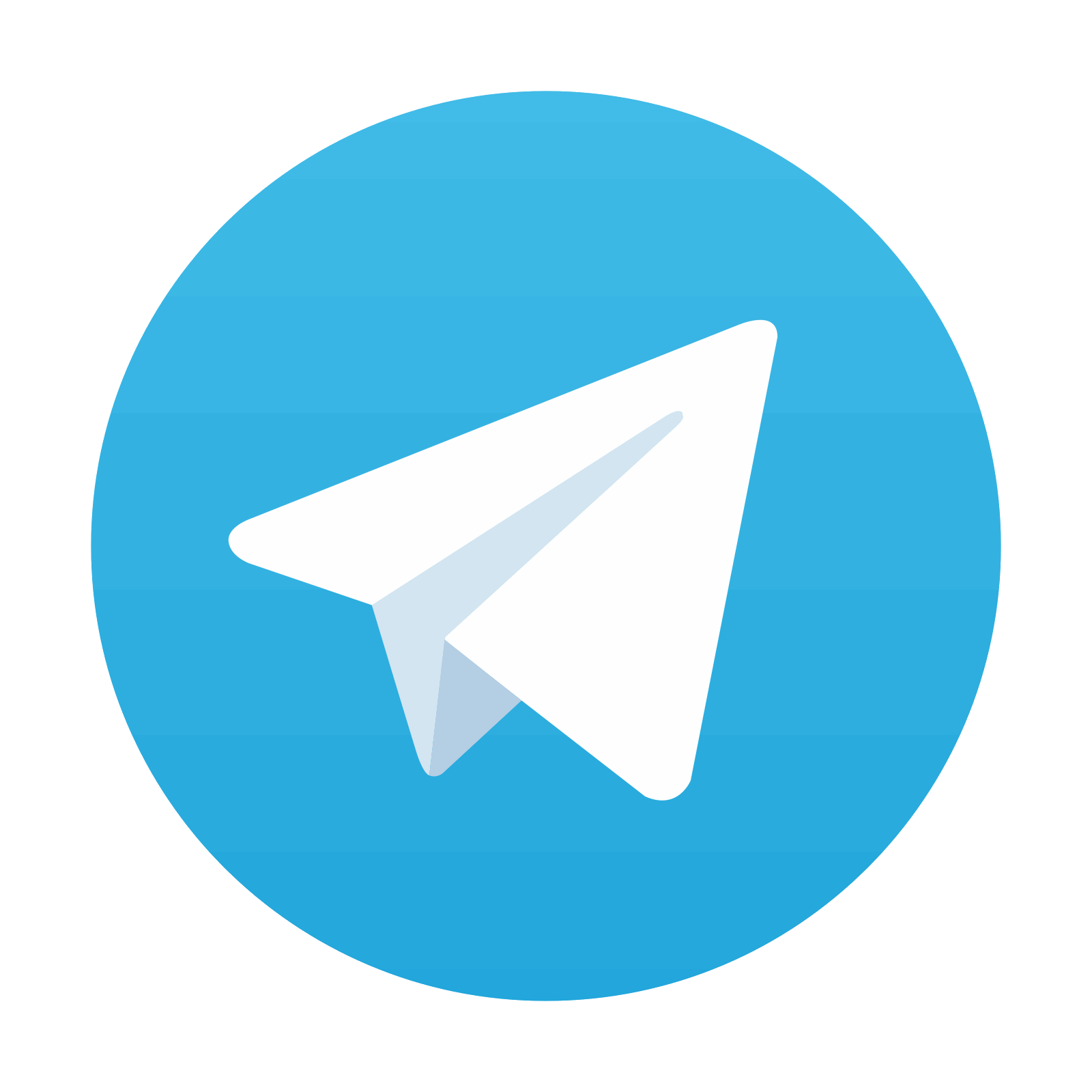
Stay updated, free articles. Join our Telegram channel

Full access? Get Clinical Tree
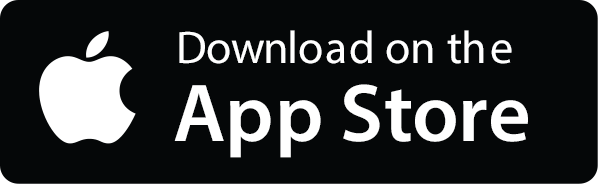
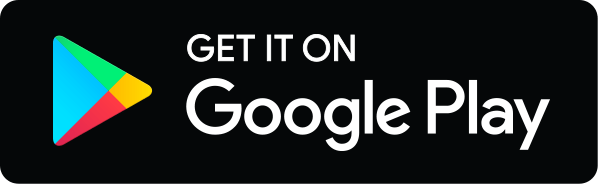