Abstract
The auditory brainstem implant (ABI) is a neuroprosthetic device that provides hearing sensation to patients who are not candidates for cochlear implantation. The ABI provides meaningful sound detection that aids lipreading but overall outcomes are poor compared to the CI. ABI outcomes are highly variable and thought to be influenced by a number of factors related to patient characteristics, blind placement technique and ABI device design. The design of the ABI device has not changed significantly in about three decades and is thought to contribute to electric current spread and poor spatial specificity of stimulation. In this chapter we provide an update on ours and others work that is focused on improving ABI device design and exploring novel stimulation modalities. Specifically we will discuss (1) Conformable ABI array technology, (2) Novel electrode coatings and (3) Light-based neural stimulation modalities in the context of the ABI.
20 Future Developments: Conformable ABI Arrays and Light Stimulation of Auditory Brainstem Using Optogenetics
20.1 Introduction
The auditory brainstem implant (ABI) is a neuroprosthetic device that provides hearing sensations to patients who are not candidates for cochlear implant (CI) surgery due to injury or absence of the cochlea or cochlear nerve. 1 The first surgery to place an ABI array on the cochlear nucleus (CN) was performed by William Hitselberger and William House in 1984. 2 A multi-channel (21-electrode) ABI manufactured by Cochlear Corporation was approved by the Food and Drug Administration (FDA) in 2000 for use in patients with neurofibromatosis type 2 (NF2), an inherited condition that causes the growth of large benign tumors of the vestibular nerve that invariably cause a significant degree of hearing loss. 3 In parallel, the MED-EL Corporation developed a multichannel (12-electrode) ABI for use outside the US that received the CE (conformité européenne) mark in 2003.
The ABI can provide meaningful sound detection that aids in lipreading but overall hearing outcomes are poor compared to those achieved by the CI. Only rarely do ABI users achieve open-set speech recognition compared to the majority of CI recipients. 4 The variability of ABI outcomes are likely influenced by a number of factors (difficulty of surgical approach, duration of deafness, neighboring nonauditory axons of passage). 5 , 6 Other contributors to the poor performance of the ABI include (1) damage to the CN caused by the tumor or its treatment (surgery or radiation), (2) suboptimal positioning of the array as a result of the “blind” placement during surgery, (3) inability of the ABI to replicate the advanced processing of the neurons of the CN, (4) poor spatial resolution of ABI stimulation caused by spread of the electric current, and (5) mechanical mismatch between electrodes and neural tissue due to stiff and noncompliant array.
The overall design of the ABI has not changed significantly over the past three decades. The primary aims of our research group at the Massachusetts Eye and Ear are to (1) examine clinical outcomes of the ABI in NF2 patients and nontumor children and adults who are deaf and are not candidates for the CI and (2) engineer and test new ABI electrode array technology that we hypothesize will reduce current spread and improve spectral resolution over existing designs.
In the following sections, we provide an update on our progress to develop (1) conformable ABI arrays that improve the mechanical mismatch seen with the current stiff array design, (2) novel coatings to improve electrical properties in smaller electrodes, and (3) “optical” or light-based stimulation modalities using optogenetics to modulate responses of the central auditory pathways.
20.2 Electrical Implant Modifications
20.2.1 Conformable Electrode Arrays for the ABI
As the field of neuroprosthetics has evolved, several engineering groups have sought to study ways to improve the electrode–neural interface. Neural implant electrode arrays in current clinical use, including the ABI, are manufactured on thick platinum and silastic substrates. 7 , 8 These materials are generally rigid and do not conform to the curved surface of neural tissues. We hypothesize that ABI electrodes mounted on a stiff backing will have suboptimal contact to the surface of the CN resulting in spread of current to neighboring structures. We also hypothesize that continuous micro- and macromotion of the pulsating brainstem may influence the durability of the electrode as well as increase scarring of the neural surface. 9 Our recent studies of perceptual thresholds as a function of electrode array position in children and adult ABI users highlight this phenomenon. 6 Some ABI users have a central cluster of usable channels with a peripheral rim of electrodes that produce side effects or do not confer any perception (Fig. 20.1).

A conformable electrode array could theoretically solve this problem by improving contact with the brainstem surface. Conformability of implanted arrays made of a durable synthetic polymer called polyimide can be tuned by altering the thickness of the sheet (Fig. 20.2). 10 Thinner polyimide sheets demonstrate greater conformability. Collaborating with Professor Stephanie Lacour at the École polytechnique fédérale de Lausanne (EPFL), we have shown in acute experimental preparations that conformable microelectrode polyimide arrays can elicit robust inferior colliculus (IC) responses in acute rodent models of the ABI. 11 Potential drawbacks of conformable technology are (1) increased fragility and (2) unfavorable surgical handling characteristics. These issues can be addressed by adding a spine or “handle” to the array to ease insertion (similar to existing designs) as well as including a rigid polymer coating that subsequently dissolves after surgery, allowing for conformation of the array to the curved brainstem.

Another important advantage of conformable materials is that their mechanical properties more closely match those of neural tissue. 9 Neural tissues are mechanically heterogeneous and exhibit Young’s moduli (measure of the ability of tissue to be deformed elastically) in the kilopascal range. 12 In contrast, stiff electronics currently used clinically for central stimulation exhibit Young’s moduli that are orders of magnitude higher. This mechanical mismatch is thought to contribute to inflammation at the electrode–brainstem interface and potentially cause higher rates of electrode failure. One material used for conformable electrode arrays is polydimethylsiloxane (PDMS), 13 a silicone-based organic polymer that has been tested in rodent and primate models of spinal cord injury. 14 , 15 Multichannel electrode arrays made with PDMS have been used to stimulate motor spinal cord rootlets for the rehabilitation of lower limb paralysis in animal models with durable responses observed several months post implantation. 9 , 15 Empirical data from our laboratory suggest that these materials can reliably evoke activity in a rodent model of the ABI for up to 1 month post-op (Fig. 20.3). 16 Minev et al 9 also showed that conformable spinal cord implants with elastic moduli more closely resembling those of neural tissue reduced the neuroinflammatory response and extent of spinal cord deformation. In a pilot study, our group has recently demonstrated that conformable arrays can be scaled up to the size of a clinical device and implanted in cadaveric human specimens. We plan to use this model to determine optimal device dimensions and quantify differences in mechanical properties between novel conformable ABI arrays and those in clinical use (Fig. 20.4).


20.2.2 Novel Electrode Coatings and Electrode Array Density
The contemporary ABI array approved for use has 12- (MED-EL, non-FDA approved) or 21-electrode contacts (Cochlear Corp, FDA approved) that ranges in diameter from 550 to 700 μm, respectively. 17 Reducing electrode size has historically been limited by higher impedances associated with smaller contact diameters. To overcome this, a number of novel electrode coatings have been proposed to reduce impedance and increase safely injectable current levels with smaller contact sizes. This approach can improve the electrical properties of platinum electrodes by (1) optimizing the effective area of contact by creating a rough surface and (2) utilizing coatings that have excellent charge injection capacity. Conducting polymers such as poly(3,4-ethylenedioxythiophene) (PEDOT) and polypyrrole (PPy) can achieve this, and we have exploited these properties in an acute preparation of a rat ABI model. 18 Most conducting polymers, however, are stiff and can alter the mechanical properties of the array. Recent studies have examined the use of a platinum elastomer mesocomposite that is more compatible with conformable implant technologies. 19 Studies of durability of these electrode coatings in vivo are lacking and will be the focus of our chronic studies.
Whether increasing the number of electrodes will improve performance of the ABI is unresolved but channel interaction from electrical current spread is well described. Kuchta et al studied perceptual performance in ABI users with different numbers of active channels and found that the best performing patients had more than three active electrodes but those with five or more active electrodes had no additional benefit in sound perception abilities. 20
20.3 Light-Based Stimulation Modalities
20.3.1 Infrared Neural Stimulation
Infrared neural stimulation (INS) is a term used to describe the activation of unmodified neural tissue by radiant energy emitted from a pulsed infrared laser source. 21 INS has been studied in various applications in both the peripheral and central nervous system. 22 , 23 , 24 , 25 For example, INS of the sciatic nerve can evoke more spatially restricted neural activity when compared to electrical stimulation. 22 In the peripheral auditory system, Richter and colleagues have demonstrated that INS of the cochlea has been shown to evoke multiunit firing in the auditory midbrain (IC). 26 INS has not yet been shown to generate far-field responses (optically evoked auditory brainstem response or ABR) in a deafened ear. In the context of the ABI, our group has demonstrated that INS of auditory brainstem does generate neural responses of the auditory system in deafened rats but the mechanism may be related to an optophonic effect. 27 Delivery of INS via flexible optical fiber generates an intense broadband acoustic click due to the stress-relaxation wave created by pulsed radiant energy and so continued study of this modality must include deafened animal models to rule out the possibility of acoustic artifact. 27 Consistent with these observations by our lab, Thompson et al reported that INS of the cochlea does not evoke auditory activity in a deafened guinea pig. 28 These results suggest that evoked neural activity from INS in subjects with residual hearing is likely generated by an opto-acoustic effect and transduced by inner hair cells rather than a primary neural response. More recently, Bin et al reported that in a deafened rat model of the ABI, auditory responses from CN stimulation could be evoked if carbon nanoparticles are applied to the CN to enhance the thermal effect of INS. 29 Spike rates achieved by INS in hearing and deafened animals in this report differ significantly, signifying the need for further studies to discern the effect of an optoacoustic phenomenon from actual neural stimulation. While the concept of improving spatial resolution using light without the need to genetically alter CN neurons is intriguing, it is important to keep in mind some notable limitations of INS. The effects of repetitive thermal application from chronic INS on underlying tissue have not been well characterized. 23 Auditory implant usage requires continuous acoustic input from the environment and the cumulative thermal energy generated by an infrared laser at high rates of stimulation may have a detrimental effect on neural tissue in the long term. Contemporary implant and battery technology also cannot support the size and energy requirements needed to power a portable infrared device. 30
20.3.2 Optogenetic Stimulation
Optogenetics is a powerful tool that has been extensively used by neuroscientists to probe neural circuits with millisecond precision 31 (Fig. 20.5) and relies on genetic transduction of neurons to enable expression of light-sensitive ion channels called “opsins.” 32 Optogenetics was developed in 2005 when Boyden et al reported the successful transduction of hippocampal neurons with channelrhodopsin-2 (ChR2), an opsin expressed by the bacterium Chlamydomonas reinhardtii, using a lentiviral vector. 33 Neurons that express ChR2 are rendered photosensitive to blue light. In contrast to electricity, light can be focused to extremely narrow beams and can theoretically enable micrometer scale spatial selectivity. 34 Another advantage that is particularly useful in the complex cellular environment of the CN is that opsin gene delivery strategies can be modified to allow for the selective transduction and opsin expression of specific cell populations. Optogenetic stimulation also offers a more physiologic method of neural stimulation by recruiting smaller diameter nerve fibers first in contrast to electrical stimulation that preferentially stimulates large bore nerve fibers. 35 The spectrum of naturally occurring and synthetic opsins has increased dramatically since optogenetics was first developed a little over a decade ago. 36 Excitatory and inhibitory opsins that respond to a wide range of photon wavelengths are now available for research purposes. 32 Optogenetics has been extensively used in basic and translational research settings to achieve targeted control of neural circuits 37 , 38 and promises unprecedented selectivity of stimulation for ABI candidates. In this section we will provide an overview of the potential and challenges to incorporate optogenetics in future auditory implants such as the ABI.

Opsin Delivery
In animal models of optogenetics, opsin expression is usually achieved by viral vector delivery or through transgenic strategies such as the cre-lox recombinase system. 39 Safe, efficient, and selective introduction of opsins into neurons of interest presents the most prominent hurdle to clinical translation. The most promising avenue toward achieving safe expression of opsins in the CN is the use of virally mediated gene delivery. Adeno-associated virus (AAV) constructs have been shown to be safe and efficacious in alleviating genetic defects in clinical trials for hemophilia B and inherited blindness. 40 , 41 Darrow et al and Hight et al from our group showed that direct pressure micro-injections of AAV constructs into CN carrying opsins ChR2 or Chronos allow for robust expression in multiple cell types. 42 , 43 Preliminary results from our group also demonstrate that systemic injections of AAV into mouse temporal or tail vein are associated with robust opsin expression in the auditory brainstem. 44 Another notable hurdle toward translating optogenetics is the limited ability of current vector strategies to selectively target auditory neural networks. In optogenetics, promoter sequences carried by viral vectors allow for the targeting of specific neural subtypes. Opsin expression in mouse models of an optogenetic ABI is widespread throughout many cell types and involves many neural networks in the brainstem and cortex. The size of many promoter sequences far surpasses the carrying capacity of contemporary viral vectors, limiting the number of promoters that can be used to effectively target specific neuronal populations. 45 In a pilot study we studied the physiological responses and histological expression in transgenic lines designed to drive opsin expression using different promoters: Bhlhb5, VGLUT-2, Atoh-1, parvalbumin, and Nestin. 46 Preliminary data characterizing expression patterns and multiunit responses in the IC demonstrate variability among these transgenic lines. Our findings suggest that advancements in vector engineering coupled with improvements in our understanding of the genetics and physiology of the CN are needed to achieve more selective activation of neurons in an optogenetic ABI. The theoretical ability of optogenetics to transfect different cell types with opsins that respond to different wavelengths can also lead to the development of more complex stimulation paradigms that may allow for further improvements in auditory performance with future optical implants.
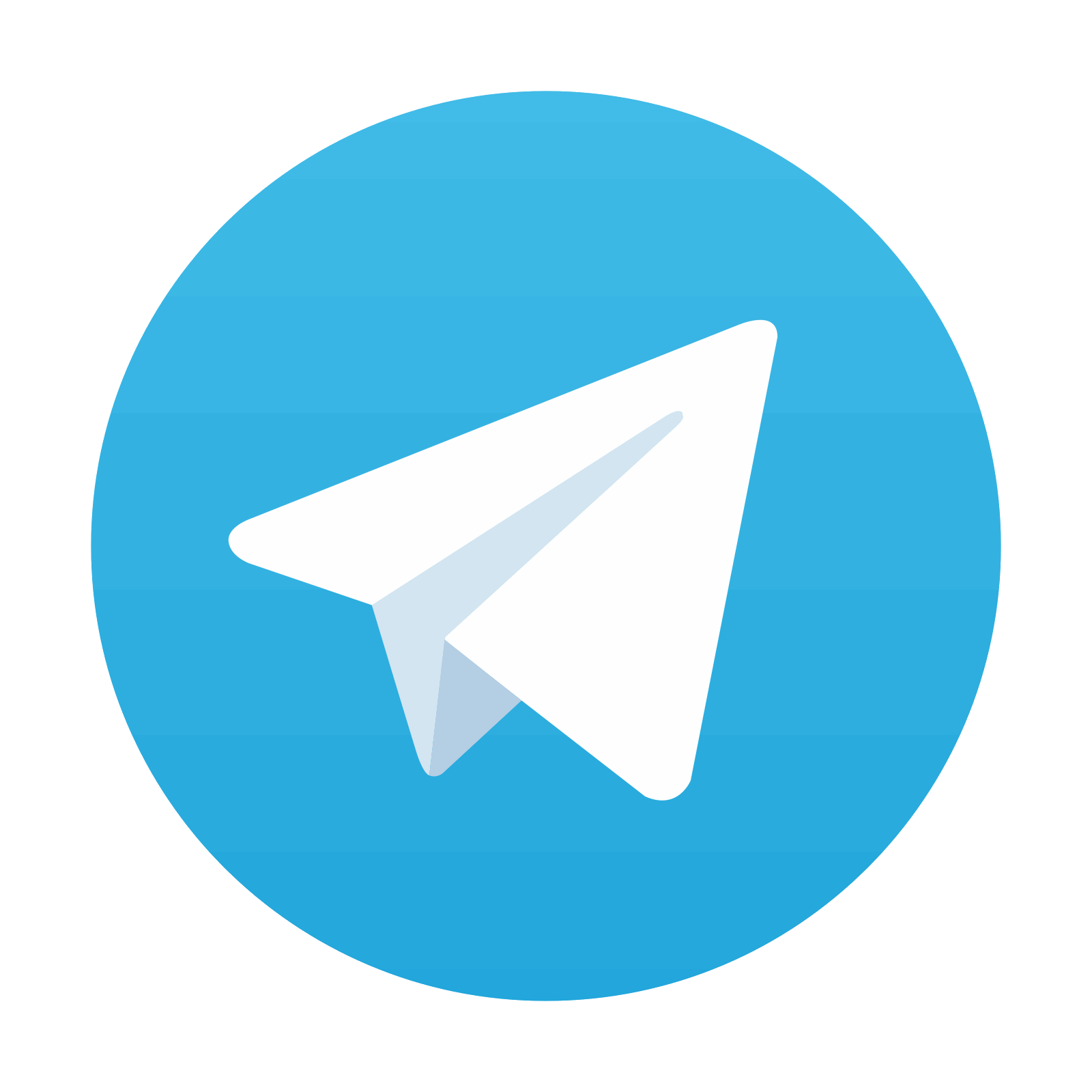
Stay updated, free articles. Join our Telegram channel

Full access? Get Clinical Tree
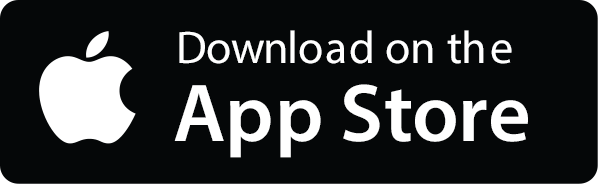
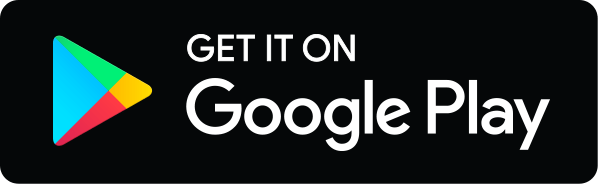
