Summary
The basal perforators comprise small direct arterial branches of the main cerebral vessels and supply the paramedian regions of the brainstem, diencephalon, and deep regions of the cerebrum. These delicate perforator vessels must be preserved during open skull base surgery to prevent significant postoperative neurological sequelae. An in-depth knowledge of perforator anatomy is essential when planning a surgical approach to complex skull base disease. Although microsurgical techniques and other intraoperative technologies have decreased the risk of perforator injury, it is imperative for the skull base neurosurgeon to thoughtfully plan out an individualized surgical approach for each patient based on the preoperative vascular imaging and specific pathology. Intraoperatively, the perforator arteries that are at risk of inadvertent injury must be meticulously identified, dissected, and displaced. Although injury to cerebral perforators is not entirely preventable, it can be greatly minimized through a combination of thoughtful planning, careful attention intraoperatively, and through the utilization of intraoperative technologies to assess perforator flow dynamics.
Key words
Basal perforators – choroidal arteries – lateral lenticulostriate arteries – medial striate arteries – microvascular anatomy – perforator injury – skull base surgery – thalamic arteries – vascular neurosurgery16 Perforator Injury During Open Skull Base Surgery
16.1 Key Learning Points
Knowledge of perforator microanatomy, its common variations, and the relationship of perforators to skull base pathology is essential for reducing morbidity and mortality in skull base neurosurgery.
Extensive preoperative planning using available vascular imaging is critical to understand each patient’s parent and perforator arterial anatomy and develop an appropriate individualized microsurgical approach to pathology of the skull base.
The skull base neurosurgeon must remain mindful that microvascular anatomy can be significantly altered in the setting of disease.
Various technologies can be used intraoperatively to assess perforator patency. These include micromirrors and endoscopes for visualization, microvascular Doppler ultrasonography, electrophysiological monitoring with evoked potentials (both motor and somatosensory), fluorescein or indocyanine green video angiography, and intraoperative digital subtraction angiography.
Optimal patient positioning and intraoperative brain relaxation can assist the neurosurgeon by minimizing the need for fixed and dynamic retraction, thereby decreasing the risk of inadvertent perforator flow disruption during skull base surgery.
The senior author commonly uses a dilute solution of papaverine (3 mg/mL) directly on the visualized perforating vessels during and after dissection to reduce manipulation-induced vasospasm, which may result in infarction.
16.2 Introduction
The basal perforating vessels (perforators) of the brain represent small direct arterial branches of the vertebrobasilar, internal carotid, and main cerebral arteries that supply the paramedian regions of the brainstem, diencephalon, and deep regions of the cerebral hemispheres (notably, the basal ganglia and internal capsules) (Fig. 16.1). These often-delicate perforator vessels serve the critical role of perfusing key areas of the cerebrum and brainstem and therefore must be preserved during open skull base surgery to prevent significant postoperative neurological sequelae. Despite significant advancements in neurosurgical techniques and intraoperative technologies, these small and tenuous vessels, which often measure less than 1 mm in diameter, remain at significant risk of damage during neurosurgical procedures, particularly during manipulation of and dissection around the delicate neurovascular structures of the skull base. An in-depth knowledge of perforator anatomy serves an indispensable role in skull base neurosurgery—because great care must be taken by the surgeon to navigate around and avoid damage of these critical structures. A comprehensive understanding of the microvascular anatomy of the cerebral circulation, including the origins of perforator arteries, their takeoff trajectories, subarachnoid course, anastomoses, and subsequent branching patterns and parenchymal distributions, is essential for selection of appropriate microsurgical or endovascular treatment modalities, particularly when the anatomy of the skull base is distorted by complex vascular or neoplastic disease. This chapter will discuss basal perforator anatomy, relevant skull base pathology, and complication avoidance when planning and performing an open surgical approach to complex pathology of the skull base.

16.3 Vascular Challenges
The vascular challenges related to perforator injury during open skull base surgery stem from the delicate nature of the basal perforator vessels, their complex and highly variable anatomy, the abundance of numerous ramifying extracerebral branches, and the often close association with skull base pathology. These vascular challenges of skull base surgery can be further complicated by multiple reoperations, radiation therapy, and chemotherapy—distorting normal anatomical planes and obscuring skull base anatomy. ▶ Table 16.1 outlines the basal perforator vessels derived from each of the major cerebral arteries and summarizes the literature regarding their parent segments, numbers (mean and range), vessel diameters (mean and range), takeoff trajectories, and structures supplied.
16.4 Perforator Injury Avoidance
16.4.1 Fundamentals of Perforator Flow Monitoring and Injury Avoidance
To avoid inadvertent injury to perforator arteries, numerous intraoperative technologies have been developed to either visualize hidden perforator branches or evaluate perforator vessel patency intraoperatively. These modalities include micromirrors or endoscopes, intraoperative digital subtraction angiography (DSA), microvascular Doppler ultrasonography, electrophysiological monitoring of motor and somatosensory evoked potentials, and fluorescein or indocyanine green (ICG) video angiography. Although these technologies have significant utility, particularly when there is concern for possible parent or perforator artery occlusion, they each have relative advantages and disadvantages and cannot always perfectly monitor for blood flow disturbance in all neighboring perforating arteries during skull base surgery.
Micromirrors and endoscopes prove particularly useful for direct visualization of critical neurovascular structures, such as perforator vessels that remain out of view when using the operating microscope (e.g., perforators located behind the dome of an aneurysm or on the deep aspect of a tumor). 13 These modalities prove particularly useful after placement of a microsurgical clip, as they allow the surgeon to visualize the backside of the aneurysm, evaluate the aneurysm’s hidden anatomical features, and ensure optimal clip placement that does not incorporate any perforators or other critical neurovascular structures into the clip construct. In addition, angled endoscopes can be useful when attempting to visualize vasculature displaced or hidden by tumors near the skull base, which can parasitize branches from the main cerebral arteries and perforators that surround them.
Microvascular Doppler ultrasonography is a valuable tool for real-time assessment of blood flow through vessels in a noninvasive manner based upon their flow velocity. 14 Although the probe can measure as small as 1 mm, it can prove to be difficult to accurately assess perforator vessel flow dynamics when placing the probe on target perforator vessels in a deep surgical field without also detecting the flow of nearby vessels, thereby confounding intraoperative assessment of vessel patency. 15 , 16 Further, microvascular Doppler ultrasonography cannot always reliably discern whether blood flow within a perforator vessel is sufficient to avoid infarction and cannot reliably assess flow within vessels smaller than 0.5 mm in diameter. 17 In contrast to the conventional microvascular Doppler ultrasonography probe, a microvascular ultrasonic flow probe (Microvascular Flowprobe, Transonics Systems Inc., Ithaca, NY) has been shown in both in vitro and in vivo studies to provide the operator with both a quantitative and a qualitative assessment of vessel flow dynamics that is not confounded by factors such as hematocrit or vessel wall thickness. 18 , 19
Electrophysiological monitoring with motor evoked potentials can detect decreased blood flow through the anterior choroidal artery (AChA), medial striate artery (MSA), or lenticulostriate artery (LSA) perforator branches within 60 seconds, 20 , 21 , 22 but detection of flow disturbance within perforators of the posterior communicating artery (PComA) or posterior thalamic arteries cannot be monitored in this fashion, as they may not supply the pyramidal tract. Furthermore, variant anatomy, collateralization, and certain highly variable perforating vessels (e.g., recurrent artery of Huebner [RAH]) can defy consistent localization, and therefore, disturbance of their flow cannot always be reliably detected via electrophysiological monitoring. The use of motor and somatosensory evoked potentials allows the operator to intraoperatively assess for compromised cerebral blood flow and infarction by observing either a decrease or a loss of evoked potentials. It should be noted, however, that electrophysiological monitoring can be influenced by anesthetic technique and in some instances can have either false-negative or false-positive evoked potential changes in the setting of ischemic and no ischemic injury, respectively. 23 , 24
Traditionally, intraoperative DSA has been used in combination with direct visualization to assess for patency of nearby parent or branching vessels and remains widely used in vascular and skull base neurosurgery. 25 , 26 Its advantages include evaluation of the entire cerebral circulation, and it can reveal adequacy of aneurysmal clip placement or residual arteriovenous malformations and fistulas. However, this method can be logistically difficult, invasive, time-consuming, and costly and can have limited resolution—making confirmation of perforator vessel patency difficult or impossible.
Fluorescein or ICG angiography has proven to be a particularly useful tool in many tumor and vascular neurosurgical cases. Fluorescein and ICG video angiography are rapid and repeatable modalities (after 20- to 30-minute washout delay) that are readily incorporated into the operating microscope and use intravascular fluorescence that enables visualization of small perforator vessels that can be difficult to discern with conventional intraoperative DSA. However, appreciable fluorescence of perforators can be restricted to the operative field and can be obscured by blood, pathology, or normal brain parenchyma. Moreover, fluorescein or ICG video angiography does not provide a quantitative assessment of perforator flow. Despite this, Raabe et al 27 demonstrated that ICG video angiography was comparable with intra- and postoperative DSA in 90% of cases and provided neurosurgeons with clinically significant intraoperative information leading to aneurysmal clip correction in 9% of cases.
Intraoperative assessment of perforator flow dynamics with the abovementioned modalities can provide critical information to the skull base neurosurgeon and can help with complication avoidance. However, it is our experience that these technologies must be combined with clinical judgment, adequate perforator dissection, and careful intraoperative inspection of the perforator branches to minimize the risk of postoperative perforator artery distribution infarctions.
16.4.2 Technical Nuances
The ideal neurosurgical skull base approach is one that allows the surgeon to successfully access and treat the underlying disease while leaving surrounding brain tissue and vessels completely undisturbed. Accordingly, preoperative planning regarding the optimal surgical approach, patient positioning, and use of gravity retraction and natural anatomical planes must be carefully thought out beforehand.
Brain relaxation can be particularly important with respect to skull base surgery and perforator artery injury avoidance, especially in cases of intracranial aneurysm rupture with elevated intracranial pressure, hyperemia, and hydrocephalus. This can be accomplished through judicious intraoperative drainage of cerebrospinal fluid via an external ventricular drain or lumbar drain, administration of intravenous hypertonic saline or mannitol at a dose of 1 to 2 g/kg body weight, preoperative administration of 10 mg of dexamethasone intravenously, mild intraoperative hyperventilation (PaCO2 30–35 mm Hg), and optimal positioning with the head elevated slightly above the heart and without excessive flexion or lateral rotation of the neck to minimize venous engorgement. Collectively, these modalities minimize cerebral volume and lessen the need for dynamic and fixed retraction by improving visualization and exposure using normal anatomical planes—thereby decreasing the risk for inadvertent flow disruption within the delicate perforator vessels by inadvertent or excessive manual brain retraction. It is also important for mean arterial pressure to be monitored closely during surgery, as manipulation of the vessels may induce local vasospasm. The deleterious resultant ischemia and ischemic penumbra may be reduced with permissive hypertension when vasospasm is encountered. It is the senior neurosurgeon’s preference to maintain or increase mean arterial pressure during dissection of vascular structures, with routine use of dilute papaverine solution (3 mg/mL in 1-mL aliquots) applied in the presence of visualized spasm of the vessels.
16.5 Related Pathologies
16.5.1 Open Skull Base Surgery for Pathology Near the Anterior Circulation
The anterior communicating artery (AComA) complex constitutes the most common site of intracranial aneurysms and is associated with approximately 30% of ruptured aneurysms. 28 , 29 Given the frequent heterogeneity of the vascular anatomy in this area (with anomalies present in up to 60% of cases), the high degree of variability in angioarchitecture of aneurysms of this region (i.e., aneurysm neck, dome size, sac morphology, and projection orientation), and the proximity of these aneurysms to neighboring perforator vessels and other critical neurovascular structures of the skull base, they prove to be some of the most challenging cases to treat surgically. 29
Aneurysms of the AComA can be approached from interhemispheric, supraorbital, orbitozygomatic, subfrontal, pterional, or extended skull base approaches. The laterality of the approach to this region often depends on symmetry of the A1 segments. If the A1 segments are symmetric, a right-sided approach is often favored to avoid involvement of the speech-dominant hemisphere. However, if one of the A1 segments is hypoplastic (i.e., <1.5 mm), then an approach on the dominant A1 side is preferred to ensure early proximal control and optimal viewing of the aneurysmal neck and dome. Regardless of surgical approach, careful dissection of the surrounding structures, particularly the optic nerve, internal carotid artery (ICA), ipsi- and contralateral A1 segments, ipsi- and contralateral frontopolar and orbitofrontal arteries, and neighboring perforator vessels must be methodically carried out. The aneurysmal neck must be carefully defined, and cleavage planes should be dissected around the neck of the aneurysm after proximal and distal control has been attained. Neighboring perforator arteries, namely, the MSA and RAH, should be dissected proximally and distally and then carefully displaced to allow clip application without occlusion of these vessels. If clip application cannot be accomplished without occlusion of adherent or intimately associated perforator vessels, a fenestrated aneurysmal clip should be employed to incorporate these delicate vessels into the construct without disturbing their flow.
Tumors of the anterior skull base and suprasellar compartment are a diverse group that encompass pathologies such as meningioma, hemangiopericytoma, pituitary adenoma, craniopharyngioma, chordoma, esthesioneuroblastoma, sarcoma, and lymphoma, among many others. These tumors may involve the anterior skull base’s contents and can displace or even encase critical neurovascular structures of this region. Accordingly, maximal safe or gross total resection can be difficult without sacrificing or disturbing flow of associated perforators. Some tumor types, such as pituitary adenomas, tend to encase nearby cerebral vasculature (e.g., ICAs) but in most cases can be separated relatively easily, whereas other tumors, like aggressive fibrous meningiomas, have a proclivity for invasion of nearby vessel adventitia—making the surgical separation of tumor from involved vessels difficult or impossible without sacrifice or leaving residual tumor behind. Preservation of involved perforators should be attempted at all costs and mandates the skull base neurosurgeon to understand the involved parent and branching perforator vessels by studying each patient’s preoperative magnetic resonance imaging (MRI) and vascular imaging.
In the case of large clinoidal meningiomas, which tend to encase neighboring vessels and perforator branches, surgical treatment of these typically benign tumors can prove particularly difficult. 30 , 31 In our experience, a pterional transylvian approach is favored, with careful dissection of the middle cerebral artery in distal-to-proximal fashion toward the ICA bifurcation. Encased perforators (e.g., LSA, MSA, and AChA) must be meticulously dissected and freed from the tumor using a combination of microdissection, bipolar electrocautery, and microscissors to facilitate their separation. The surgeon should carefully explore the entrance points of the perforator vessels into the tumor along with their exit points (when applicable) and dissect along the anatomical layer that separates the vessels from the tumor. Knowledge of the parent vessel course relative to the tumor and perforator entrance points is essential when dissecting these small, often friable perforators free, as intratumoral vasculature can further complicate their identification and dissection. When identification of these branches is difficult, dividing the tumor into several segments or carefully debulking can improve visualization and ease of identification of involved perforator vessels—aiding in their safe dissection and separation from the tumor. Carefully debulking a tumor that encases the perforators can help minimize the risk of progressive compression and disruption of normal perforator flow dynamics, leading to vessel thrombosis and stroke.
Understanding of the preoperative anatomy is essential for intraoperative decision-making, because inadvertent sacrifice of a perforator branch will likely lead to a significant postoperative neurological deficit. Leaving behind a small amount of tumor that can be treated postoperatively with adjuvant radiotherapy or chemotherapy is almost always preferable to an aggressive gross total resection with significant postoperative neurological deficit.
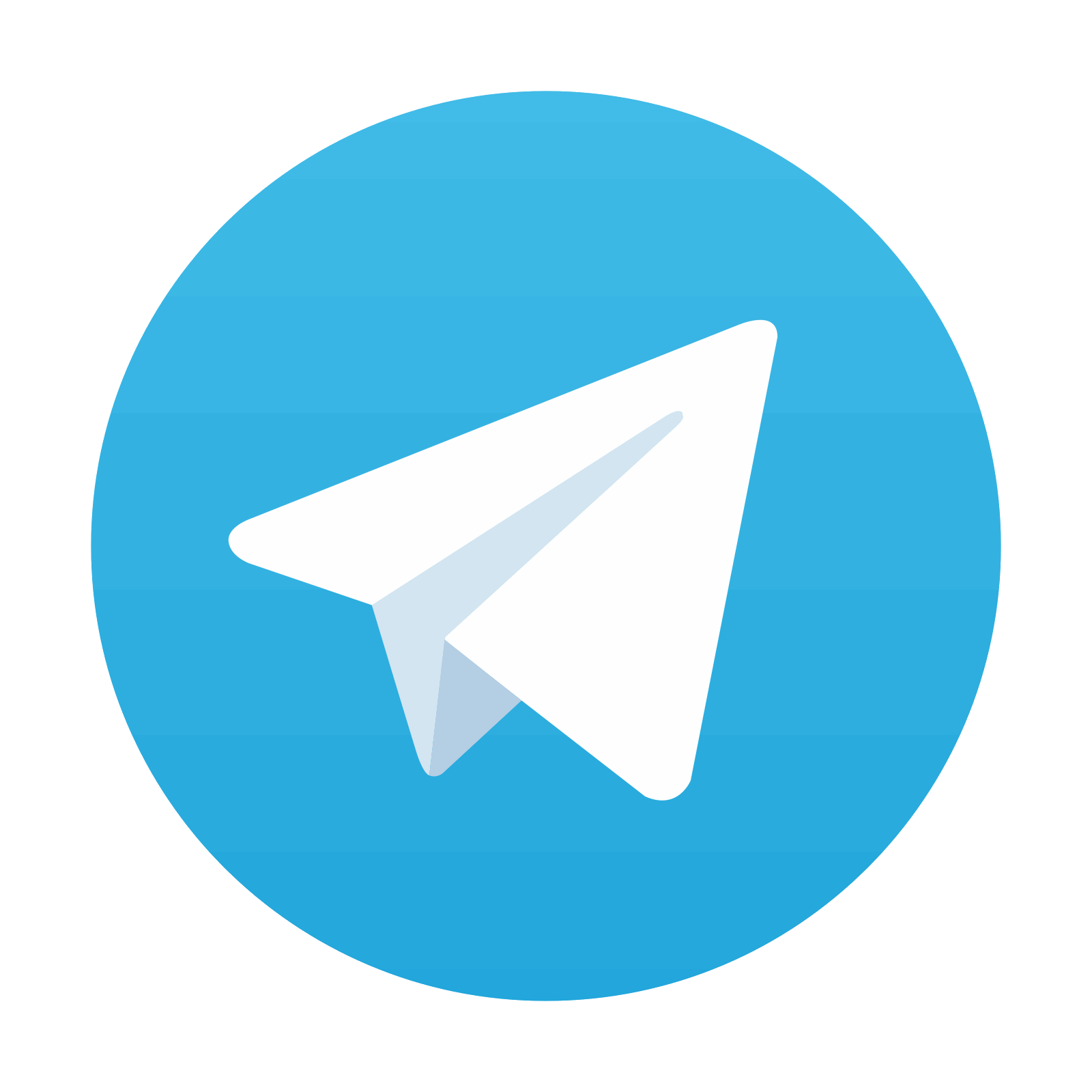
Stay updated, free articles. Join our Telegram channel

Full access? Get Clinical Tree
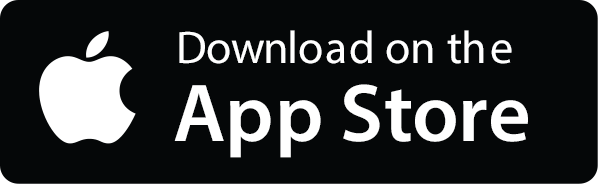
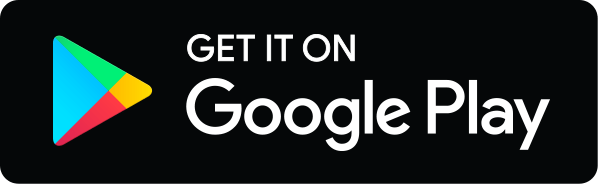
