10 Molecular Advances in the Diagnosis and Treatment of Thyroid Cancer
10.1 Introduction
Significant work has been done over the last few decades to illuminate the genetic basis of thyroid neoplasia. These efforts, accelerated in recent years by the availability of increasing amounts of data generated through next-generation sequencing technologies, have led to the elucidation of the cancer genes underlying the pathogenesis of the majority (> 90%) of thyroid tumors. Indeed, thyroid tumors are among the few tumors in which this level of understanding has been achieved to date. This knowledge (1) allows the accurate diagnosis of tumor, (2) aids in tumor classification, (3) provides guidance in the clinical management of patients with thyroid tumors, (4) offers potential targets for therapy, and (5) forms a framework for future discoveries of new cancer genes or biomarkers.
This chapter reviews the molecular mechanisms underlying thyroid cancer, which primarily involve the mitogen-activated protein kinase (MAPK) and phosphatidylinositol 3-kinase (PI3K) pathways. Recent data from studies examining multiple genes mutated in thyroid cancer as well as data from more than 400 thyroid carcinomas sequenced by The Cancer Genome Atlas initiative suggest that, for most well-differentiated follicular cell–derived thyroid tumors, a single mutation in a driver gene is sufficient for tumor development. Aggressive or poorly differentiated tumors, on the other hand, are more likely to have multiple mutations. These additional acquired mutations are likely late events that promote tumor progression and aggressiveness. Other molecular alterations are found typically only in benign nodules, and can be helpful in differentiating tumor from benign disease.
In addition to playing a role in thyroid cancer diagnosis, molecular findings are increasingly being used to determine surgical management of thyroid nodules, or, in the case of familial medullary thyroid cancer, to determine the need for prophylactic surgery. Examples of how molecular findings can be used to guide medical and surgical management of patients as well as potential targeted therapies will also be discussed in this chapter.
10.2 Gene Alterations in Thyroid Disease
The genetic landscape of different types of thyroid tumors includes genetic alterations that occur in different tumor types and alterations that are specific for certain types of thyroid tumors (Fig. 10.1).

10.2.1 Papillary Thyroid Cancer
Papillary thyroid cancer (PTC) is the most common type of thyroid cancer, accounting for approximately 80% of all thyroid malignancies and 90% of thyroid cancers in children. Most papillary thyroid cancers are associated with a low mortality rate, with a survival rate of > 98%. 1 However, approximately 10% of patients will have recurrent disease. 2
A common molecular alteration, seen in approximately 45% of papillary thyroid cancers, is mutation of the BRAF gene. 1 , 3 BRAF is a serine-threonine kinase that functions in the MAPK pathway. BRAF is activated by RAS. Activated BRAF then activates MEK, leading to downstream activation of effectors in the MAPK pathway. BRAF is typically activated by point mutation, with the BRAF V600E mutation seen in more than 98% of cases. The BRAF K601E mutation can be seen in 1 to 2% of cases, and other BRAF mutations, such as small in-frame insertions or deletions, account for the remainder. 4 , 5 , 6 , 7 In addition to point mutations and insertion or deletion mutations, BRAF may be activated as a result of chromosomal rearrangement, such as one leading to a fusion of BRAF to AKAP9 (A kinase protein anchor 9). 8 The AKAP9–BRAF fusion is seen in up to 11% of cases associated with radiation exposure but is uncommon in sporadic papillary cancers. 8 Specific types of PTC are associated with different BRAF mutations. The BRAF V600E mutation is seen most frequently in classical and tall-cell variants of PTC and the BRAF K601E mutation is frequently seen in the follicular variant of PTC. 9 , 10 , 11
Another important alteration in PTC involves fusion of the RET (rearranged during transfection) gene with other partner genes. The RET gene is a receptor tyrosine kinase, and typically the 3´ portion of RET encoding the tyrosine kinase domain is involved in oncogenic fusions with the 5´ portion of partner genes. 12 , 13 Several types of RET rearrangements, termed RET/PTC, have been described. 14 , 15 , 16 , 17 , 18 , 19 , 20 , 21 , 22 Of these RET/PTC rearrangements, the most common types are RET/PTC1 (which is formed by the fusion of RET with CCDC6) and RET/PTC3 (which is formed by the fusion of RET with NCOA4). 14 , 15 RET/PTC rearrangements are found in approximately 10 to 20% of PTC cases. 23 , 24 Tumors carrying RET/PTC rearrangement are usually classic PTC, although these rearrangements have been observed in other variants of PTC. 25 , 26 , 27 RET/PTC rearrangements are typically found more commonly in children and young adults, and in association with radiation exposure. 28 , 29 , 30
The RAS genes (HRAS, NRAS, and KRAS) also play a role in PTC. RAS proteins are G proteins that function in signaling to the MAPK and PI3K pathways. RAS mutations are more typically associated with follicular cancers and will be discussed in further detail later in the chapter, but RAS mutations also account for 10 to 20% of PTCs. 31 , 32 , 33 RAS mutations are seen almost exclusively in the follicular variant of PTC, in contrast to other mutations, such as BRAF V600E, which are rarely found in this variant of PTC. 34
Rearrangements involving the NTRK genes account for up to 5% of PTCs. NTRK1 and NTRK3 are members of the neurotrophic receptor tyrosine kinase family of genes, and their fusions also activate the MAPK signaling pathway. To date, three fusion partners, TPM3, TPR, and TFG, have been identified for NTRK1, and one fusion partner, ETV6, has been identified for NTRK3. 35 , 36 , 37 , 38 , 39 These rearrangements may occur in higher frequencies in patients with radiation exposure. 8 , 39 , 40 Rearrangements involving the ALK gene, particularly STRN-ALK, occur in approximately 1% of PTCs. 41
10.2.2 Follicular Thyroid Tumors
Follicular thyroid carcinomas, including the conventional and oncocytic (Hürthle cell) types, account for 10 to 15% of thyroid cancers. Follicular thyroid adenoma is a benign tumor belonging to this group. Point mutations in RAS genes (HRAS, NRAS, and KRAS), which typically occur in codons 12, 13, and 61, are found in 40 to 50% of follicular carcinomas and in 20 to 40% of follicular adenomas. 31 , 32 , 33 Of the RAS genes, the most commonly mutated is NRAS codon 61, followed by HRAS codon 61, and then KRAS codons 61 and 12/13. A recent study of 204 thyroid nodules found that nodules with KRAS codon 12/13 mutation had a lower risk of carcinoma (41.7%) than nodules with NRAS codon 61 mutation (86.8% risk) or HRAS codon 61 mutation (95.5% risk), and furthermore that KRAS codon 12/13 mutation was associated with oncocytic change. 42
Another important alteration in follicular thyroid tumors involves rearrangement of the PAX8 and PPARG genes. PAX8 is a paired domain transcription factor and PPARG is a nuclear hormone receptor. The PAX8/PPARG rearrangement has been reported in 30 to 40% of follicular carcinomas. 43 , 44 , 45 This rearrangement is also present at lower frequencies in oncocytic (Hürthle) cell carcinomas, in the follicular variant of PTC, and in follicular adenomas. 43 , 44 , 45 , 46 , 47
Genes involved in the PI3K/AKT signaling pathway have also been implicated in follicular tumors. Aberrant signaling of the PI3K/AKT pathway may be due to activation of upstream regulators, may result from activating mutations in PIK3CA and AKT1, or may result from inactivation of PTEN, which negatively regulates this pathway. PIK3CA is a catalytic subunit of PI3Ks and is typically activated by mutations in exon 9 and exon 20. Mutation of PIK3CA has been reported in 6 to 13% of follicular carcinomas. 48 , 49 , 50 PTEN mutation may occur as germline or somatic events; germline PTEN mutations can cause follicular tumors in patients with PTEN hamartoma syndrome, and somatic PTEN mutations can be seen in both follicular thyroid tumors and follicular adenomas. 49 , 50 , 51 , 52 , 53
10.2.3 Poorly Differentiated and Anaplastic Thyroid Cancer
Poorly differentiated thyroid carcinomas are partially dedifferentiated cancers, whereas anaplastic (undifferentiated) carcinomas lose entirely the phenotypical features of thyroid and even epithelial differentiation. It is thought that most poorly differentiated and anaplastic carcinomas arise via dedifferentiation of a well-differentiated cancer. Both well-differentiated and poorly/undifferentiated components are often seen in these tumors, and both components often share mutations in RAS or BRAF.
RAS mutations have been reported in 25 to 30% of poorly differentiated carcinomas and 40 to 50% of anaplastic carcinomas, whereas BRAF mutation has been reported in 10 to 15% of poorly differentiated carcinomas and 20 to 30% of anaplastic carcinomas. Poorly differentiated or anaplastic tumors with RAS or BRAF mutation often harbor other mutations as well, which contribute toward tumor dedifferentiation. The most common additional mutations involve the TP53 and CTNNB1 genes. TP53 is a tumor suppressor with roles in DNA repair, cell cycle regulation, and apoptosis. Most mutations in TP53 are point mutations within the DNA binding domain. TP53 mutations have been seen in 20 to 30% of poorly differentiated carcinomas and 70 to 80% of anaplastic carcinomas. 54 , 55 , 56 , 57 , 58 CTNNB1 (beta-catenin) is a gene involved in the Wnt signaling pathway. Typically, mutations in CTNNB1 occur at phosphorylation sites, resulting in stabilization of the protein and increased signaling. Mutations in CTNNB1 have been reported in 25% of poorly differentiated carcinomas and 60 to 70% of anaplastic carcinomas. 59 , 60 ALK fusions are found in 5 to 10% of these tumors and result in constitutive activation of ALK kinase. 41
The PI3K pathway also plays a role in these tumors. Mutations in PIK3CA have been observed in 10 to 20% of anaplastic carcinomas. 48 , 49 In addition, one study reported the presence of AKT1 mutation in metastatic poorly differentiated carcinoma. 61
Recently, two mutations in the promoter region of telomerase (TERT) have been described in thyroid cancer. Telomerase is a reverse transcriptase that functions to maintain telomere length and is frequently expressed in tumor cells. 62 These TERT promoter mutations, c.–124C > T (C228T) and c.–146C > T (C250T), were first described in melanoma and were found to increase promoter activity. 63 , 64 In thyroid cancer, these mutations were detected in follicular cell–derived thyroid cancers (but not in medullary carcinoma), and were present at the highest frequency in poorly differentiated and anaplastic carcinomas. 65 , 66 , 67 , 68
10.2.4 Medullary Thyroid Cancer
Medullary thyroid carcinoma arises from C (parafollicular) cells and accounts for approximately 3 to 4% of thyroid cancers. 1 The majority of medullary carcinomas are sporadic, but medullary carcinoma may also arise in multiple endocrine neoplasia type 2A or 2B (MEN2A or MEN2B) syndrome, or in familial medullary thyroid carcinoma (FMTC). Medullary carcinomas that develop in the context of familial syndromes typically are multicentric and occur in younger patients.
RET gene mutation is commonly found in both familial and sporadic medullary thyroid carcinoma. In contrast to papillary thyroid cancer, where the 3´ portion of the RET gene is activated via chromosomal rearrangement, in medullary thyroid carcinomas, RET is activated by point mutation. In sporadic medullary carcinomas, the most common mutation is RET M918T. 69 , 70 The M918T mutation is located within the tyrosine kinase domain and results in altered substrate specificity. 71 The M918T mutation is also seen in > 90% of medullary carcinomas arising in MEN2B syndrome. 70 , 72 , 73 , 74 In MEN2A and FMTC, RET mutations usually occur at cysteine residues in the cysteine-rich extracellular domain. In MEN2A, this commonly involves codon 634, whereas in FMTC, other cysteine residues can be involved. 75 , 76 Mutation of a cysteine residue in the extracellular domain confers the mutant RET protein the ability to undergo ligand-independent dimerization with another mutant RET protein.
More recent studies have shown that RAS mutations also occur in sporadic medullary thyroid carcinomas, and are mutually exclusive with RET mutations. 77 , 78 , 79 HRAS and KRAS mutations occur in approximately 8% of these tumors, which tend to behave less aggressively. 78
10.2.5 Genetic Alterations in Hyperfunctioning Thyroid Nodules
Hyperfunctioning (hot) thyroid nodules, which on histopathologic examination may be diagnosed as follicular adenomas, hyperplastic nodules, or, rarely, follicular carcinomas, often carry mutations in thyroid-stimulating hormone receptor (TSHR) or GNAS. TSHR encodes a membrane receptor whose activity is mediated by G proteins. Activating mutations in TSHR have been reported in 50 to 80% of nodules. 80 , 81 These mutations are typically located in the extracellular and transmembrane domains of TSHR. GNAS encodes an alpha subunit of heterotrimeric G protein complexes, and thus functions downstream of TSHR. Activating mutations of GNAS, which mostly occur in codons 201 and 227, have been seen in 3 to 6% of hyperfunctioning nodules. 82 , 83 , 84 Although TSHR mutations at specific hotspots may also be seen in thyroid carcinomas, in a limited series of cases, all nodules with GNAS mutation were benign. 53 Thus GNAS mutation may be a unique marker of benign nodules.
10.2.6 Additional Molecular Alterations in Thyroid Cancer
miRNA Expression in Thyroid Carcinomas
A micro RNA (miRNA) is a small, noncoding RNA that functions in regulating gene expression. miRNAs bind to the 3´ untranslated region of target mRNAs and cause degradation or translation inhibition. Several miRNAs have been identified in miRNA microarray studies and found to be recurrently up- or downregulated in thyroid carcinoma.
In PTC, many miRNAs have been identified, but some of the best characterized include miR-187, -221, -222, and -146b, which have been found to be significantly overexpressed in tumor tissue in several studies. 85 , 86 , 87 , 88 miR-146b upregulation has been associated with advanced tumors, extrathyroidal extension, and poor prognosis. 89 , 90 In follicular carcinomas, miR-221, -222, and -146b as well as miR-182, -183, -96, -874, and -449a were reported to be upregulated, and miR-542–5p, -574–3p, -455–3p, -455–5p, -199a-5p, -199a-3p, and 125a-3p were downregulated. 86 , 91 , 92 , 93 Interestingly, differential expression of miR-885–5p was observed between conventional and oncocytic follicular carcinomas. 93 A number of miRNAs were found to be altered in poorly differentiated and anaplastic thyroid carcinomas. 86 , 94 , 95
Methylation in Thyroid Carcinomas
DNA methylation typically occurs on cytosine residues in CpG islands (regions with a high occurrence of CpG dinucleotides), which typically are located in gene promoters. Hypermethylation of promoters may result in silencing of tumor suppressor genes, and, conversely, hypomethylation can result in increased transcription of oncogenes. In addition, hypomethylation is thought to lead to chromosome instability.
In PTC, hypermethylation of several genes has been observed, including tissue inhibitor of metalloproteinases 3 (TIMP3), SLC5A8, death-associated protein kinase 1 (DAPK1), and TSHR. 96 , 97 , 98 , 99 Methylation of these genes was observed in association with the BRAF V600E mutation. 99 These genes function in several cellular pathways: DAPK1 is a calmodulin-dependent serine threonine kinase with roles in apoptosis; SLC5A8 is an iodide, monocarboxylate, and short-chain fatty acid transporter; and TSHR is thyroid-stimulating hormone receptor. TIMP3 functions by binding to the metalloproteinase zinc cofactor, thereby inactivating metalloproteinase. Studies have suggested that silencing of the TIMP3 gene and the subsequent loss of metalloproteinase inhibition are associated with extrathyroidal invasion and lymph node metastasis. 99
In follicular thyroid tumors, hypermethylation of genes, including PTEN and RASSF1, have been reported. 98 , 100 , 101 , 102 Alterations of the PI3K-AKT pathway, in which PTEN functions, are common in follicular tumors. RASSF1 is a gene related to the RAS genes and has roles in cell cycle regulation and apoptosis. PTEN and RASSF1 hypermethylation have been seen in both follicular thyroid tumors and benign lesions, such as follicular adenomas and hyperplastic nodules. 98 , 100 , 101 , 103
Altered DNA methylation patterns have been seen in poorly differentiated and anaplastic thyroid carcinomas as well. Some evidence exists that, compared to well-differentiated thyroid carcinomas, anaplastic thyroid carcinomas tend to have aberrant gene promoter hypomethylation rather than hypermethylation. 104
10.3 Utility of Molecular Testing in Diagnosis, Management, and Therapy
10.3.1 Molecular Diagnostics in Indeterminate Cytology Thyroid Nodules
The diagnosis of thyroid cancer through the use of ultrasound examination and cytological examination of cells obtained by fine-needle aspiration (FNA) typically diagnoses the majority of thyroid nodules as benign or malignant. 105 , 106 However, in approximately 20 to 30% of nodules, cytological analysis of the cells results in an indeterminate classification. 107 , 108 These indeterminate categories include atypia of undetermined significance or follicular lesion of undetermined significance (AUS/FLUS) (Bethesda category III), follicular neoplasm or suspicious for a follicular neoplasm (FN/SFN) (Bethesda category IV), and suspicious for malignancy (SUSP) (Bethesda category V). 107 , 109 The risk of malignancy is 5 to 15% in the AUS/FLUS category; 15 to 30% in the FN/SFN category, and 60 to 75% in the SUSP category. 109 However, on surgical resection, only 10 to 40% of indeterminate nodules are malignant. 105 , 110 , 111
Molecular testing of indeterminate cytology thyroid nodules offers the ability to correctly identify and diagnose malignancies, including low-grade malignancies, and to classify malignancies based on likelihood of progression or aggressive behavior. In evaluating the utility of any test, comparisons of sensitivity, specificity, positive predictive value (PPV), and negative predictive value (NPV) are important. A test that is useful to rule out malignancy should have a high sensitivity (percentage of correctly identified positives) and a high NPV (percentage of patients with a negative test result who do not have the disease). To rule in malignancy, a test with high specificity (percentage of correctly identified negatives) and high PPV (percentage of patients with a positive test result who have the disease) should be used. It is important to note that, while sensitivity and specificity of a test reflect test performance, NPV and PPV can vary based on the prevalence (or pretest probability) of malignancy. In clinical practice, prevalence of malignancy may change not only based on the patient population but also by institutional variations in malignancy rates conferred by each cytological diagnosis.
Approaches to Molecular Testing
Many different diagnostic tools have been used in indeterminate cytology thyroid nodules in an attempt to better classify these nodules. These diagnostic tools were developed based on knowledge accumulated about mechanisms underlying thyroid tumorigenesis and include immunohistochemical markers, miRNAs, gene mutations/rearrangements, and gene expression markers. 105 , 106 , 111 , 112 Although all of these tools have been shown to have utility as diagnostic markers, the two types of molecular markers currently being used in clinical practice are gene mutations/rearrangements and gene expression markers, and will be discussed in further detail. Gene mutation/rearrangement testing is being offered in institutions and commercial laboratories as single-gene testing or panel testing, and a gene expression panel is currently offered commercially.
Gene Mutation/Rearrangement Testing
The molecular genetics of thyroid tumors are very well characterized, with many mutations and rearrangements that are specific for malignancy. The single gene mutation BRAF V600E is specific and has a high PPV for malignancy, and testing for this mutation has been shown to increase the sensitivity of FNA biopsies. 111 , 113 , 114 Mutations in the RAS genes have a lower PPV for malignancy of 74 to 87%. 110 , 115 , 116 This is due to the occurrence of RAS mutations in benign follicular adenomas. However, although follicular adenomas are benign, some studies have found evidence that RAS mutations in follicular adenomas are precancerous changes that may progress to malignancy. 117 , 118 , 119 , 120
Increased sensitivity and specificity from single-gene testing can be achieved by using multigene panels. A multigene panel consisting of the seven (or eight) most commonly altered genes (BRAF, KRAS, HRAS, NRAS, PAX8/PPARG, RET/PTC1, RET/PTC3 [and TRK rearrangement]) has been demonstrated to provide high specificity and PPV for cancer detection in three prospective studies. 114 , 118 , 119 With the seven gene panel for molecular testing, high NPV (94%) was achieved for AUS/FLUS cytology nodules; though lower NPV was seen in FN/SFN and SUSP nodules. 110
Advances in technology have made it possible to test for more gene mutations using the limited amount of DNA available in FNA biopsy specimens. Next-generation sequencing (NGS) platforms allow high-throughput, massively parallel nucleic acid sequencing, and highly sensitive and quantitative assessment of the mutant allele (Fig. 10.2). NGS has been increasingly integrated into clinical use for molecular testing as an efficient and cost-effective way of analyzing multiple genes in one reaction (Fig. 10.3). Expanded diagnostic panels are expected to further increase sensitivity and NPV, as was shown in a recent report of an NGS thyroid gene mutation panel that included > 60 genes and gene fusions. 121 This panel expanded from the previously described seven-gene panel to also test for mutations in hotspots of RET, GNAS, TSHR, CTNNB1, TP53, AKT1, PTEN, PIK3CA, and TERT, and 42 types of rearrangements involving RET, BRAF, PPARG, NTRK1, NTRK3, ALK, and THADA. In FN/SFN cytology thyroid nodules, the 60-gene panel showed high sensitivity (90%), specificity (93%), PPV (83%), and NPV (96%) for cancer detection. 121


An additional advantage of NGS-based mutational analysis is the ability to detect the presence of multiple mutations. Although the majority of thyroid nodules with mutations have only a single molecular alteration, in a small number of cases, multiple mutations may be present. 121 , 122 Thus identification of molecular alterations can be useful diagnostically, but finding multiple mutations in a thyroid nodule may have prognostic implications as well and will be discussed in further detail.
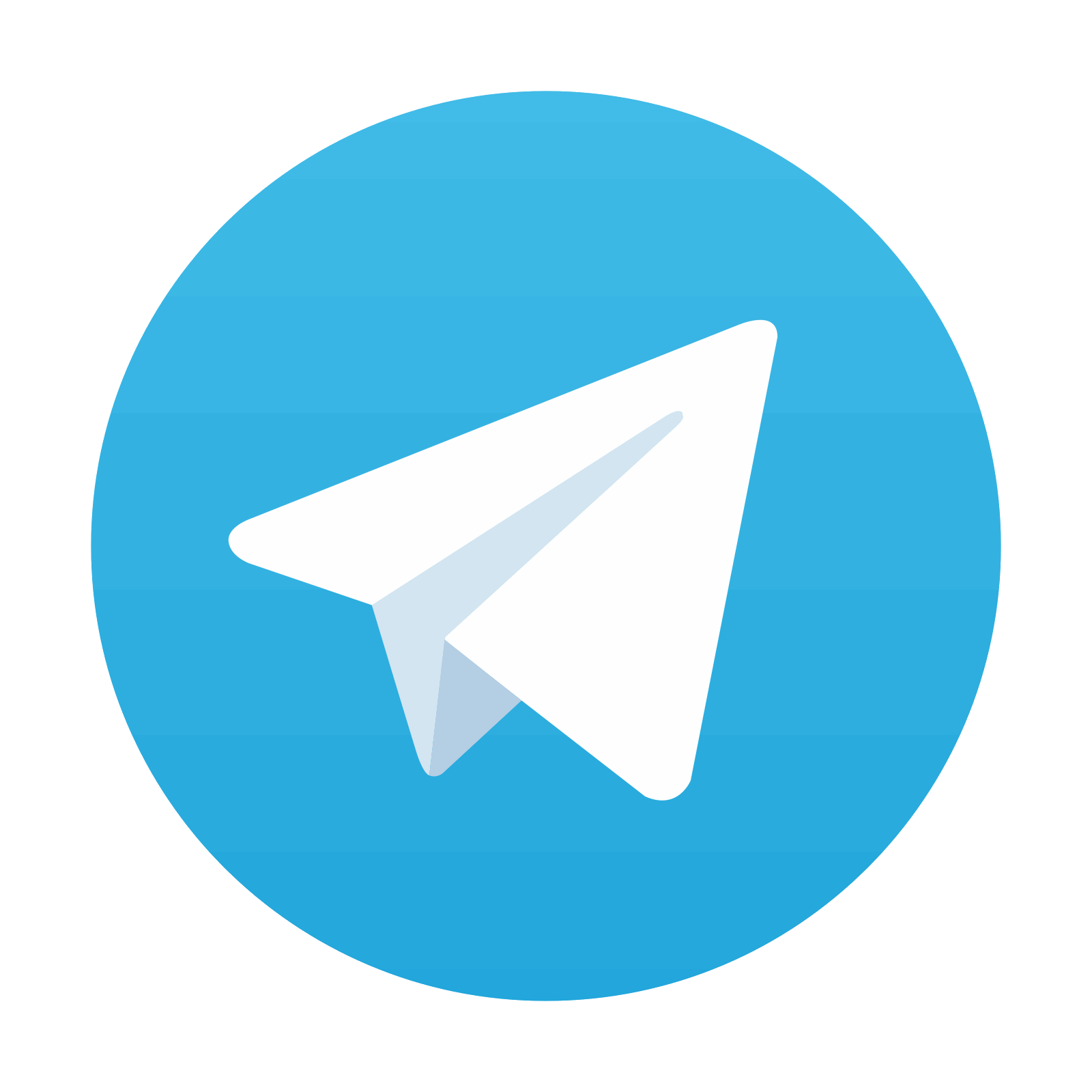
Stay updated, free articles. Join our Telegram channel

Full access? Get Clinical Tree
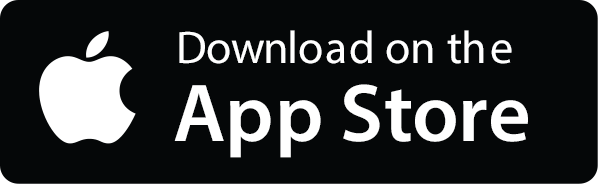
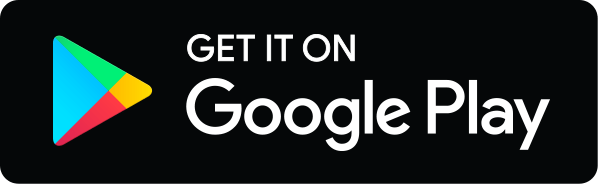
